Abstract
Water-soluble chitosan derivative-based nanoparticles (carboxymethyl chitosan-chitooligosaccharide nanoparticles (CMC-COS NP) and sulphated chitosan-chitooligosaccharide nanoparticles (SC-COS NP)) were prepared by the formation of polyelectrolyte complexes. SC-COS NP and CMC-COS NP both induced the proliferation of mouse fibroblasts, whereas they elicited dose-dependent inhibitory effects on the proliferation of both HeLa and mouse B16 melanoma cells. Injection of SC-COS NP and CMC-COS NP modulated serum Th cytokines and stimulated the proliferation of splenic lymphocytes (CD4+ and CD8+ T cells, CD19+ B cells and NK cells) in mice, indicating the ability of these particles to regulate both humoral and cell-mediated immune responses. These properties demonstrated their promising potential for application as biomedical materials.
1. Introduction
Chitosan, a cationic polysaccharide consisting of β(1 → 4) linked N-acetyl-D-glucosamine and D-glucosamine units, is considered to be a biocompatible material that possesses such bioactivities as antitumor, immune-enhancing and antimicrobial properties Citation1,Citation2. Although chitosan is biodegradable and noncytotoxic Citation3, it is only soluble in aqueous acidic solutions, which limits its pharmaceutical and biomedical applications under physiological conditions Citation4. Water-soluble chitosan derivatives, such as chitooligosaccharide (COS), carboxymethyl chitosan (CMC) and sulphated chitosan (SC), have therefore been developed to overcome this solubility limitation.
COS, a water-soluble oligomer prepared from chitosan by either chemical or enzymatic methods, is reported to have anti-inflammatory effects and anti-tumor activities Citation5–7. SC and CMC, the water-soluble chitosan derivatives, also have been further developed as drug carriers encompassing vaccine and gene delivery systems Citation8–12. These data suggest that nanoparticles prepared using water-soluble chitosan derivatives are useful drug vehicles. However, to date, there has been no report regarding the immuno-modulatory activities of the water-soluble chitosan derivative-based nanoparticles.
In this study, we developed the polyelectrolyte nanoparticles by combining COS (a cationic cross-linker) with an anionic chitosan derivative polymer, SC and CMC (SC-COS NP and CMC-COS NP). We first investigated the effect of SC-COS NP, CMC-COS NP and their starting materials (COS, SC, and CMC) on the proliferation of mouse fibroblasts, the cell model often utilised to analyse if the testing materials are cytotoxic Citation13; we also evaluate the cytotoxic effects of these nanoparticles on mouse B16 melanoma and human Hela cell lines, both of which are reported to have high metastatic potentials Citation14,Citation15, and then the in vivo immunological regulatory effects of these materials on serum cytokines and splenic lymphocyte subpopulations in mice after their intraperitoneal administration were evaluated.
2. Experiments
2.1. Preparation of N-CMC
N-CMC was prepared according to Muzzarelli et al. Citation16. In brief, 5.0 g of chitosan (MW 54,700 Da, degree of deacetylation 98%; Koyo Chemical Company, Tottori, Japan) was dissolved in 700 mL aqueous 0.7% (v/v) acetic acid solution. Glyoxylic acid monohydrate (8.6 g) (MW 92.05 Da; Wako, Osaka, Japan) was then added to chitosan solution under stirring at room temperature for 60 min. The solution was adjusted to pH 4.5 with 1 M NaOH and stirring was continued for further 45 min. Subsequently, 72 mL 5% (w/v) aqueous solution of sodium borohydride (MW 37.83 Da; Wako, Osaka, Japan) was added dropwise over 30 min to the chitosan solution and the mixture was left for 1 h under stirring at room temperature, then excess of acetone was added and the precipitate was collected by centrifugation (1000 g, 10 min). The pellet was re-dissolved in deionised water and the remaining precipitate was removed by filtration (Grade 1 Whatman cellulose filters, Whatman, Tokyo, Japan) under vacuum. The filtrate was adjusted to pH 7.0 with 1 M NaOH, dialysed against deionised water using seamless cellulose tubing (MWCO 12,000–16,000; Wako, Osaka, Japan) for 72 h, and freeze-dried to obtain CMC powder. The degree of substitution (DS) of the carboxymethylated groups in CMC was determined by elemental analysis (Elemental Vario EL III, Hanau, Germany). The average DS of CMC used in this study was 0.95 per glucose moiety.
2.2. Preparation of 2-N,6-O-SC
2-N,6-O-SC was prepared according to Zhou et al. Citation17. In brief, 50 mL of formamide (MW 45.04 Da; Wako, Osaka, Japan) and 2 mL formic acid (MW 46.03 Da; Wako, Osaka, Japan) was added to 500 mL three-necked bottomed flask containing 0.5 g chitosan. The mixture was heated to 55°C and stirred at 55°C for 1 h to dissolve chitosan. To prepare the sulphating reagent, 8 mL HClSO3 (MW 116.52 Da; Wako, Osaka, Japan) was added dropwise over 15 min to pre-cooled N,N-dimethylformamide (DMF) (4°C) (50 mL) (MW 73.09 Da; Wako, Osaka, Japan) under stirring. This reagent was then added dropwise to chitosan solution under mechanical agitation and argon atmosphere. The reaction was maintained at 55°C for 2.5 h, and a homogeneous pale yellow solution was finally obtained. Ethanol (95%, 300 mL) was then slowly added and the precipitate was collected by filtration under vacuum. The collected precipitate was re-dissolved in deionised water and the remaining precipitate was removed by centrifugation (1000 g, 10 min). The supernatant was adjusted to pH 7.0 with 1 M NaOH, dialysed against deionised water using seamless cellulose tubing for 72 h, and freeze-dried to obtain SC powder. The DS of the sulphur groups in SC was determined by elemental analysis. The average DS of SC used in this study was 0.77 per glucose moiety.
2.3. Preparation of CMC-COS NP and SC-COS NP
CMC-COS NP or SC-COS NP was prepared via ionotropic gelation between positively charged crosslinker COS (KoyoOligochitosan, weight-average molecular weight 1.8 kDa; Koyo Chemical Company, Tottori, Japan) and negatively charged chitosan derivatives, CMC or SC, that was prepared as described above. To prepare nanoparticles, the molar ratio of amino groups in COS to carboxyl groups in CMC and to sulphate groups in SC was set at 1.0–1.1 and 1.0–0.8, respectively.
COS (1.0 mg/mL), CMC (2.0 mg/mL) and SC (2.0 mg/mL) were dissolved in deionised water, respectively. CMC-COS NP and SC-COS NP was spontaneously formed upon the dropwise addition of 22 mL CMC solution and 16 mL SC solution, respectively, to 30 mL COS solution under stirring at a speed of 2000 rpm (LT400D Stirrer, Yamato Instrument, Tokyo, Japan) at room temperature. Stirring was maintained for 30 min to stabilise the CMC-COS NP and SC-COS NP systems. The mixture was centrifuged at 6000 g for 30 min at 15°C (Compact High Speed Refrigerated Centrifuge 7780; KUBOTA, Japan) to remove the precipitate. The supernatant was then filtered through a sterilised 1.0-µm membrane filter (Omnipore Membrane Filter, Millipore, Ireland) to obtain the CMC-COS NP and SC-COS NP suspensions.
To calculate the production yield of CMC-COS NP and SC-COS NP, nanoparticles was separated by centrifugation at 25,000 g for 30 min at 4°C (High Speed Refrigerated Centrifuge MX-305; TOMY, Japan), and the precipitate was washed with deionised water using the same centrifugation condition (twice) and was then freeze-dried to obtain dried CMC-COS NP and SC-COS NP. The production yield was calculated as follows:
2.4. Characterisation of CMC-COS NP and SC-COS NP
The particle sizes of nanoparticles prepared as described above were measured using a particle size analyser, SALD 2000A (Shimadzu Corporation, Tokyo, Japan), and the zeta values of nanoparticles were determined using Horiba nano Partica SZ-100 (Horiba Corporation, Kyoto, Japan). The morphological examination of nanoparticles was performed using a scanning electron microscopy (SEM) (JOEL-JSM 5800; Nippon Electric Industry, Tokyo, Japan).
2.5. Effect of CMC-COS NP and SC-COS NP on cell proliferation of 3T6 mouse fibroblasts, HeLa cells, and B16 mouse melanoma cells
COS, CMC, SC, CMC-COS NP and SC-COS NP, prepared as described above, were tested in this study.
Swiss albino mouse embryonic fibroblasts 3T6 (JCRB9059) were cultured in a complete growth culture medium at 37°C in a 5% CO2 incubator. Cells were seeded into 96-well flat-bottomed tissue-culture plates at a density of 2 × 104 cells/well. After culture for 24 h, the cells were immediately treated with various doses (25, 50, 75 and 100 µg/mL) of samples for another 48 h. The effect of different treatments on cell proliferation was assessed using Cell Counting Kit-8 (CCK8, Dojindo Laboratories, Kumamoto, Japan). Non-treated cells were also cultured under the same conditions using PBS (pH 7.4) (non-toxic control group) or 1% Triton X-100 (Sigma) (toxic control group) in place of test solutions.
Human epitheloid cervical carcinoma cells (HeLa cells, JCRB9004) and mouse melanoma (B16, JCRB0202) were seeded into 96-well flat-bottomed tissue-culture plates at a density of 1 × 105 and 2 × 105 cells/well, respectively, and were then cultured and assayed as described above.
2.6. Effect of CMC-COS NP and SC-COS NP on serum cytokines and splenocyte subpopulation in mice
Healthy male 5-week-old BALB/c mice, weighing 15–25 g, were used for this study. Animal treatments were performed on the basis of the Guidelines for Animal Experimentation of the Faculty of Agriculture of Tottori University, the Japanese Government Animal Protection and Management Law (no. 105), and the Japanese Government Notification on Feeding and Safekeeping of Animals (no. 6) with the permission of the Animal Committee of the Faculty of Agriculture of Tottori University (approval no. 1996-1).
The endotoxin levels of CMC-COS NP, SC-COS NP, COS, CMC and SC used in this study were below the limits of detection using a limulus amebocyte lysate assay (<0.1 EU/mL) (Lonza QCL-1000 Chromogenic LAL Assay, Lonza Walkersville Inc., Walkersville, Maryland, USA). Five mouse sample treatment groups (CMC-COS NP, SC-COS NP, COS, CMC and SC, n = 15 per group) received 10.0 mg sample/kg body weight by intraperitoneal injection (i.p.), the injected dosage (10.0 mg/kg) was used according to our previous study Citation18,Citation19. At each designated time point (1 and 3 h (short-term effects) and 24 h (long-term effects) after injection), five mice from each sample treatment group were killed, and blood was collected from the heart. Blood collected from the control group (n = 5) (without any treatment) was assigned as 0 h. Serum cytokines (GM-CSF, IFN-gamma, IL-1, IL-2, IL-4, IL-5, IL-6, IL-10, IL-17, TGF-beta, TNF-alpha and IL-23) were analysed using the FlowCytomix Mouse Th complex kit (eBiosceince, San Diego, California, USA) and a Flow Cytometer (FACS Aria; BD Biosciences, San Jose, California, USA) according to the instructions provided by the manufacturer.
In addition, mouse spleens were harvested aseptically from control group and each sample treatment group at each designated time point (1, 3 and 24 h after injection), placed in disposable Petri dishes containing sterile, ice-cold PBS with 5% fetal calf serum, and were then mechanically disrupted with a syringe plunger and passed through a 70 µm nylon mesh strainer (BD Biosciences; Bedford, Massachusetts, USA). Erythrocytes were hemolysed in red blood cell lysis buffer (0.15 M NH4Cl/1 M KHCO3/0.1 mM Na2EDTA, pH 7.2) for 5 min and cells were then washed in RPMI 1640 medium containing 10% fetal calf serum. After centrifugation (1000 g, 5 min.), the cells were collected and washed twice with RPMI 1640 medium containing 10% fetal calf serum. After the second wash, the cells were suspended in PBS containing 10% fetal calf serum at a density of 1 × 106 cells/mL. The viability of the cell suspension was approximately 95% based on a trypan blue dye exclusion assay. Splenocyte suspensions (1 × 106 cells/mL) were stained with fluorescent-labelled antibodies (1 µg/L × 106 cells) against the following cell surface markers (BD Pharmingen, BD Biosciences, San Jose, California, USA): CD19 (clone: 1D3), CD4 (clone: RM4-5), CD8a (clone: 53–6.7), NKG2A/C/E (clone: 20d5) and antibody isotype control: rat IgG2a (clone: R35-95). The cells were incubated on ice for 45 min, washed twice with cold PBS containing 5% fetal calf serum and then fluorescence was analysed using a flow cytometer (FACSAria; BD Biosciences, San Jose, CA, USA). The distribution of the splenocyte markers was performed by using BD FACSDiva Software V6.0 (BD Biosciences).
2.7. Statistical analysis
Data were analysed using SPSS 16.0 for Windows (SPSS, Chicago, IL, USA) and were presented as means ± standard deviation.
3. Results and discussions
3.1. Preparation and characterisation of CMC-COS NP and SC-COS NP
CMC-COS NP or SC-COS NP, which were formed by ionic gelation of COS and CMC or SC (). The pH values of the suspensions of CMC-COS NP and SC-COS NP were 6.7 and 6.6, respectively, which is very close to the physiological pH value. The average particle size of CMC-COS NP (311.3 ± 12.3 nm) was half that of SC-COS NP (676.9 ± 29.2 nm), while the production yield of CMC-COS NP (77.3% ± 2.6%) was lower than that of SC-COS NP (83.5% ± 3.5%). The zeta potential values of aqueous solutions of COS, CMC and SC were 8.5, −14.7 and −12.3 mV, respectively, whereas the zeta values of both blank (drug-free) and drug-loaded CMC-COS NP and SC-COS NP suspensions were zero, indicating that there was complete electrostatic attraction between the positively charged amino groups of COS and the negatively charged carboxyl groups of CMC or sulphate groups of SC in the nanoparticles Citation20.
3.2. Effect of CMC-COS NP and SC-COS NP on 3T6 mouse fibroblasts proliferation
COS, CMC and SC, the materials for the preparation of CMC-COS NP and SC-COS NP, had no effects on the proliferation of 3T6 mouse fibroblasts in this study. In contrast, both CMC-COS NP and SC-COS NP could enhance proliferation of these cells: 25, 50, 75 and 100 µg/mL SC-COS NP showed 26.3%, 30.0%, 28.7% and 26.8% enhancement of cell proliferation, respectively; 25, 50, 75 and 100 µg/mL CMC-COS NP showed 28.7%, 32.8%, 30.2% and 29.0% enhancement of cell proliferation, respectively. These results demonstrate that COS, CMC, SC, CMC-COS NP and SC-COS NP, at concentrations of 25–100 µg/mL, were not cytotoxic towards fibroblasts and that CMC-COS NP induced higher cell proliferation than did SC-COS NP. Additionally, the concentration of both CMC-COS NP and SC-COS NP that induced optimal cell proliferation was 50 µg/mL (p < 0.05), but not 100 µg/mL. This result implies that an excessive amount of chitosan-based nanoparticles may have an unfavourable effect on cells, which was similar to the previously reported reduction in fibroblasts proliferation that was observed following application of chitosan-tripolyphosphate nanoparticles Citation21.
3.3. Inhibitory effects of CMC-COS NP and SC-COS NP on the proliferation of HeLa cells and B16 mouse melanoma cells
CMC had no effects on the proliferation of either HeLa or B16 cells in this study, whereas SC slightly inhibited HeLa cell proliferation (5.8% and 8.4% inhibition at 75 and 100 µg/mL, respectively) (). Although SC did not affect the proliferation of B16 melanoma cells in this study, SC has been reported to effectively inhibit the in vitro proliferation of human melanoma A375 cells Citation22. These results suggest that different cell models may have different sensitivities to SC.
Figure 2. In vitro effects of blank CMC-COS NP and SC-COS NP on human HeLa and mouse melanoma (B16) proliferation. Notes: *Significantly different vs. SC-COS NP. (p < 0.05). §Significantly different vs. COS and SC (p < 0.05). #Significantly different vs. control (nanoparticles-free) (p < 0.05).
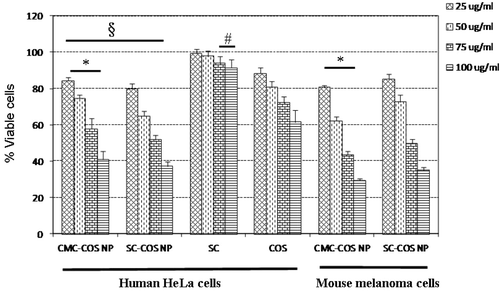
The COS showed dose-dependent inhibitory effects on the proliferation of HeLa cells (22.5–38.5% inhibition at concentrations of 25–100 µg/mL) (), while it weakly inhibited B16 cell proliferation (4.2% inhibition at 100 µg/mL), suggesting that different cancer cell models also have different sensitivities to COS Citation23. CMC-COS NP and SC-COS NP, the nanoparticles made from COS, CMC and SC, displayed dose-dependent inhibitory effects on the proliferation of both HeLa and B16 cells, and these inhibitory effects were stronger than those of COS and SC (). In addition, SC-COS NP showed stronger inhibition of HeLa cell growth than CMC-COS NP, but weaker inhibition of B16 cell growth than CMC-COS NP (), indicating that different tumor cell models also have different sensitivities to CMC-COS NP and SC-COS NP.
3.4. Regulation of the levels of serum cytokines in mice by CMC-COS NP and SC-COS NP
All mice of the in vivo study remained healthy before being sacrificed for the subsequent analysis of serum cytokines and splenic lymphocytes in this study. None of the injected samples induced serum IFN-gamma, IL-1, or IL-2. All injected samples down-regulated serum levels of the pro-inflammatory cytokines IL-4, IL-5, GM-CSF, and TNF-alpha within 24 h compared to the control group (0 h) (except for SC that had no effect on the serum IL-5 level) (). IL-10, an anti-inflammatory cytokine, was also induced after injection of all samples (). The induction of IL-10 has been reported to suppress the production of IL-1 and IFN-gamma Citation24, which may be the reason why IL-1 and IFN-gamma could not be detected in this study.
Figure 3. Effects of (a) COS, (b) CMC, (c) SC, (d) CMC-COS NP or (e) SC-COS NP on serum cytokine levels in mice. Notes: *Significantly different vs. control (0 h) (p < 0.05). #Significantly different vs. control (0 h) (p < 0.01).
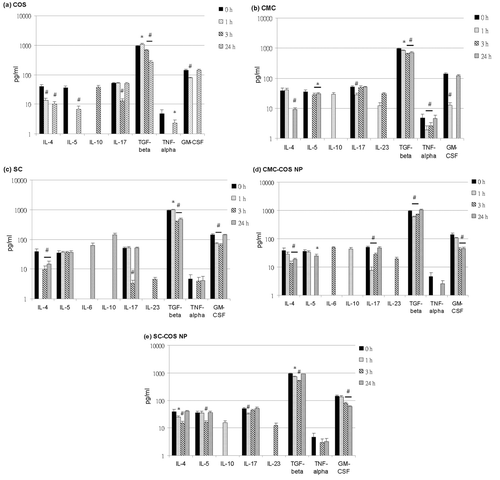
IL-6, which behaves as both an anti-inflammatory agent and a pro-inflammatory mediator, was induced after injection of SC (62.8 pg/mL, at 24 h) and CMC-COS NP (48.1 pg/mL, at 3 h) (c and d). IL-17, a pro-inflammatory cytokine that is closely involved in autoimmune disease Citation25, was decreased after injection of COS (at 3 h), SC (at 3 h), CMC (at 1 h), CMC-COS NP (at 1 and 3 h), and SC-COS NP (at 1 and 3 h) (). IL-17 is reported to be up-regulated in the presence of IL-6/TGF-beta and IL-23, while it is down-regulated in the presence of IL-10/TGF-beta and IL-4 () Citation26–28. Both IL-10 and IL-23 were induced at 1 h after injection of CMC, at which time IL-17 was reduced (), indicating that the combined effect of IL-10 and TGF-beta (although TGF-beta was slightly reduced, ) on the down-regulation of IL-17 may predominate over IL-17 up-regulation by IL-23. In contrast, at 3 h after injection of CMC, when more IL-23 was induced and IL-10 and IL-4 were undetectable, the level of IL-17 was recovered (), indicating that IL-17 was up-regulated under this condition through IL-23. At 3 h after SC injection, the IL-4 level recovered slightly and IL-23 was induced, but IL-17 was down-regulated (), indicating that the effect of the recovered IL-4 on IL-17 downregulation may predominate over IL-17 up-regulation by IL-23. At 24 h after SC injection, when IL-4 levels were further increased and IL-10 was induced, the induction of IL-6, a slight recovery of TGF-beta levels and full recovery of IL-17 levels were also observed (), indicating that the effect of IL-6/TGF-beta on the up-regulation of IL-17 may predominate over IL-17 downregulation by IL-4 and IL-10/TGF-beta.
Figure 4. Under the influence of different cytokines indicated with black arrow, Naive T cells can differentiate into Th2, Treg or Th17 cells. IL-4 and IL-10/TGF-beta (indicated with red arrow), which are secreted by Th2 and Treg cell, respectively, are reported to have the down-regulation effects of IL-17 produced by Th17 cell.
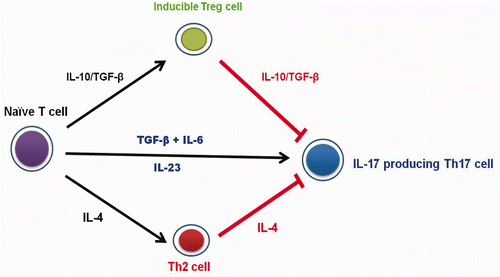
Although injection of CMC-COS NP and SC-COS NP reduced TGF-beta levels at 1 h, IL-10 was induced and IL-17 was decreased, and a similar trend in these cytokine levels was also observed at 3 h in COS group (), indicating that IL-10/TGF-beta might be a major cause of IL-17 down-regulation. At 3 h after injection of CMC-COS NP, IL-6 and IL-23 were induced, TGF-beta was recovered, IL-4 was decreased, IL-10 was undetectable and IL-17 was partially recovered (), suggesting that the observed up-regulation of IL-17 was mediated through IL-23 and IL-6/TGF-beta. In contrast, at 3 h after injection of SC-COS NP, IL-23 was the major cause of the IL-17 recovery since IL-6 was undetectable at this time point ().
These data suggested that COS, CMC, SC, CMC-COS NP and SC-COS NP might regulate the serum IL-17 level through modulation of IL-4, IL-6, IL-10 and IL-23, whether the TGF-beta level was up- or down-regulated (). Although TGF-beta has been reported to be an important cofactor for IL-6 and IL-10 for IL-17 regulation Citation27, the IL-6 and IL-10 that were induced following injection of the water-soluble chitosan materials in this study might be the major cytokines for modulation of the serum IL-17 levels in these mice, while TGF-beta might be a minor regulator of IL-17 under these conditions.
3.5. Regulation of mouse splenocyte subpopulations by CMC-COS NP and SC-COS NP
The proliferation of mouse splenic CD4+, CD8+ and CD19+ cells was stimulated within 24 h of injection of all samples, while NK cell proliferation was stimulated at 3 and 24 h after injection (). Nanoscale CMC-COS NP and SC-COS NP were more effective in stimulating these splenocyte subpopulations than their starting materials, COS, CMC, and SC (p < 0.05) (). Additionally, SC-COS NP was more effective than CMC-COS NP in stimulating the proliferation of CD4+ and NK cells at 3 h (p < 0.05), whereas CMC-COS NP was more effective than SC-COS NP in stimulating the proliferation of CD4+ cells at 24 h and of B CD19+cells at 1 h (p < 0.05) ().
Figure 5. Percentage of splenocyte subpopulations ((a) T CD4+, (b) T CD8+, (c) NK cells and (d) B CD19+ cells) in the mice treated with COS, CMC, SC, CMC-COS NP or SC-COS NP. Notes: *Significantly different vs. control (0 h) (p < 0.05). #Significantly different vs. control (0 h) (p < 0.01). §Significantly different vs. COS, CMC and SC (p < 0.05).
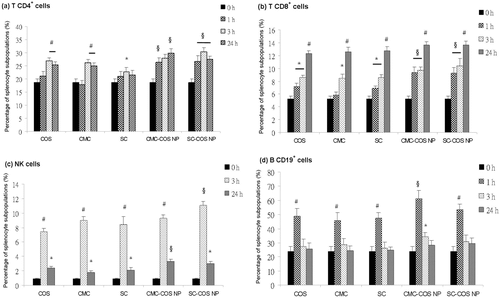
Activation of T and B cells involves the production of cytokines and cell proliferation. That the induction of serum cytokines, IL-6, IL-10 and IL-23, and the increase of serum TGF-beta levels () accompanied with the increased percentage of splenocyte subpopulations detected in this study () suggested that the injection of COS, CMC, SC, CMC-COS NP and SC-COS NP could in vivo significantly activate lymphocytes Citation29, and that nanoscale CMC-COS NP and SC-COS NP were more effective than their starting materials, the water-soluble chitosan derivatives.
Anticancer vaccines have been reported to induce T CD4+/CD8+ and NK cells Citation30; the ability of CMC-COS NP and SC-COS NP to regulate Th serum cytokines and to stimulate the proliferation of spleen lymphocytes indicates that both of these nanoparticles can regulate both humoral and cell-mediated immune responses and are therefore promising candidate vaccine adjuvants.
4. Conclusions
In this study, nanoparticles were successfully prepared using COS, SC and CMC via ionic gelation. CMC-COS NP and SC-COS NP, which were nontoxic to mouse fibroblasts and showed stronger inhibition of the proliferation of HeLa and B16 melanoma cells compared to their starting materials, COS, CMC and SC, could regulate both humoral and cell-mediated immune responses through modulating the serum level of Th cytokines and stimulating the proliferation of mouse spleen lymphocytes. These properties of CMC-COS NP and SC-COS NP, which were prepared using water-soluble chitosan derivates, demonstrate their potential usefulness for the immuno-active modulation.
Acknowledgements
We thank Professor Yoshihiro Hosoi (Faculty of Engineering, Tottori University) for providing particle size analyser. We also thank to Kunio Ichino (Faculty of Engineering, Tottori University) for the technical assistance of SEM.
References
- Kong , M , Chen , XG , Xing , K and Park , HJ . 2010 . Antimicrobial properties of chitosan and mode of action: A state of the art review . Int. J. Food Microbiol. , 144 : 51 – 63 .
- Muzzarelli , RA . 2010 . Chitins and chitosans as immunoadjuvants and non-allergenic drug carriers . Mar. Drugs , 8 : 292 – 312 .
- Wang , JJ , Zeng , ZW , Xiao , RZ , Xie , T , Zhou , GL , Zhan , XR and Wang , SL . 2011 . Recent advances of chitosan nanoparticles as drug carriers . Int. J. Nanomedicine , 6 : 765 – 774 .
- Harish Prashanth , KV and Tharanathan , RN . 2007 . Chitin/chitosan: Modifications and their unlimited application potential─an overview . Trends Food Sci. Technol. , 18 : 117 – 131 .
- Aam , BB , Heggset , EB , Norberg , AL , Sorlie , M , Varum , KM and Eijsink , VG . 2010 . Production of chitooligosaccharides and their potential applications in medicine . Mar. Drugs , 8 : 1482 – 1517 .
- Qiao , Y , Bai , XF and Du , YG . 2011 . Chitosan oligosaccharides protect mice from LPS challenge by attenuation of inflammation and oxidative stress . Int. Immunopharmacol. , 11 : 121 – 127 .
- Park , JK , Chung , MJ , Choi , HN and Park , YI . 2011 . Effects of the molecular weight and the degree of deacetylation of chitosan oligosaccharides on antitumor activity . Int. J. Mol. Sci. , 12 : 266 – 277 .
- Luo , Q , Zhao , J , Zhang , X and Pan , W . 2011 . Nanostructured lipid carrier (NLC) coated with chitosan oligosaccharides and its potential use in ocular drug delivery system . Int. J. Pharm. , 403 : 185 – 191 .
- Qu , G , Yao , Z , Zhang , C , Wu , X and Ping , Q . 2009 . PEG conjugated N-octyl-O-sulfate chitosan micelles for delivery of paclitaxel: in vitro characterization and in vivo evaluation . Eur. J. Pharm. Sci. , 37 : 98 – 105 .
- Sahu , SK , Mallick , SK , Santra , S , Maiti , TK , Ghosh , SK and Pramanik , P . 2010 . In vitro evaluation of folic acid modified carboxymethyl chitosan nanoparticles loaded with doxorubicin for targeted delivery . J. Mater. Sci.: Mater. Med. , 21 : 1587 – 1597 .
- Sayin , B , Somavarapu , S , Li , XW , Thanou , M , Sesardic , D , Alpar , HO and Senel , S . 2008 . Mono-N-carboxymethyl chitosan (MCC) and N-trimethyl chitosan (TMC) nanoparticles for non-invasive vaccine delivery . Int. J. Pharm. , 363 : 139 – 148 .
- Zhang , YF , Yin , P , Zhao , XQ , Wang , J , Wang , CD , Ren , L and Zhang , QQ . 2009 . O-Carboxymethyl-chitosan/organosilica hybrid nanoparticles as non-viral vectors for gene delivery . Mater. Sci. Eng. C: Mater Bio. Appl. , 29 : 2045 – 2049 .
- Zhang , J , Chen , XG , Sun , GZ , Huang , L and Cheng , XJ . 2010 . Effect of molecular weight on the oleoyl-chitosan nanoparticles as carriers for doxorubicin . Colloids Surf. B: Biointerfaces , 77 : 125 – 130 .
- Hoang , TM and Eichenfield , LF . 2000 . The rising incidence of melanoma in children and adolescents . Dermatol. Nurs. , 12 : 188 – 189 .
- Krause , DR , Piva , TJ , Brown , SB and Ellem , KA . 1997 . Characterization and localization of mitochondrial oligopeptidase (MOP) (EC3.4.24.16) activity in the human cervical adenocarcinoma cell line HeLa . J. Cell. Biochem. , 66 : 297 – 308 .
- Muzzarelli , RA , Tanfani , F , Emanuelli , M and Mariotti , S . 1982 . (Carboxymethylidene) chitosans and N-(Carboxymethyl)-chitosans – Novel chelating polyampholytes obtained from chitosan glyoxylate . Carbohydr. Res. , 107 : 199 – 214 .
- Zhou , H , Qian , J , Wang , J , Yao , W , Liu , C , Chen , J and Cao , X . 2009 . Enhanced bioactivity of bone morphogenetic protein-2 with low dose of 2-N, 6-O-sulfated chitosan in vitro and in vivo . Biomaterials , 30 : 1715 – 1724 .
- Lin , YS , Radzi , R , Morimoto , M , Saimoto , H , Okamoto , Y and Minami , S . Characterization of chitosan-carboxymethyl dextran nanoparticles as a drug carrier and as a stimulator of mouse splenocytes, J. Biomater Sci. Polym. Ed. (2011), in press
- Nishimura , K , Nishimura , S , Seo , H , Nishi , N , Tokura , S and Azuma , I . 1987 . Effect of multiporous microspheres derived from chitin and partially deacetylated chitin on the activation of mouse peritoneal macrophages . Vaccine , 5 : 136 – 140 .
- Xu , Y and Du , Y . 2003 . Effect of molecular structure of chitosan on protein delivery properties of chitosan nanoparticles . Int. J. Pharm. , 250 : 215 – 226 .
- Nasti , A , Zaki , NM , Leonardis , P , Ungphaiboon , S , Sansongsak , P , Rimoli , MG and Tirelli , N . 2009 . Chitosan/TPP and chitosan/TPP-hyaluronic acid nanoparticles: Systematic optimisation of the preparative process and preliminary biological evaluation . Pharm. Res. , 26 : 1918 – 1930 .
- Wang , R , Huang , J , Wei , M and Zeng , X . 2010 . The synergy of 6-O-sulfation and N- or 3-O-sulfation of chitosan is required for efficient inhibition of P-selectin-mediated human melanoma A375 cell adhesion . Biosci. Biotechnol. Biochem. , 74 : 1697 – 1700 .
- Shen , KT , Chen , MH , Chan , HY , Jeng , JH and Wang , YJ . 2009 . Inhibitory effects of chitooligosaccharides on tumor growth and metastasis . Food Chem. Toxicol. , 47 : 1864 – 1871 .
- Shibata , Y , Foster , LA , Kurimoto , M , Okamura , H , Nakamura , RM , Kawajiri , K , Justice , JP , Van Scott , MR , Myrvik , QN and Metzger , WJ . 1998 . Immunoregulatory roles of IL-10 in innate immunity: IL-10 inhibits macrophage production of IFN-gamma-inducing factors but enhances NK cell production of IFN-gamma . J. Immunol. , 161 : 4283 – 4288 .
- Deknuydt , F , Scotet , E and Bonneville , M . 2009 . Modulation of inflammation through IL-17 production by gammadelta T cells: Mandatory in the mouse, dispensable in humans . Immunol. Lett. , 127 : 8 – 12 .
- McGeachy , MJ , Bak-Jensen , KS , Chen , Y , Tato , CM , Blumenschein , W and McClanahan , T . 2007 . TGF-beta and IL-6 drive the production of IL-17 and IL-10 by T cells and restrain Th-17 cell-mediated pathology . Nat. Immunol. , 8 : 1390 – 1397 .
- Mills , KH . 2008 . Induction, function and regulation of IL-17-producing T cells . Eur. J. Immunol. , 38 : 2636 – 2649 .
- Sutton , CE , Lalor , SJ , Sweeney , CM , Brereton , CF , Lavelle , EC and Mills , KH . 2009 . Interleukin-1 and IL-23 induce innate IL-17 production from gammadelta T cells, amplifying Th17 responses and autoimmunity . Immunity , 31 : 331 – 341 .
- Delves , PJ , Martin , SJ , Burton , DR and Roitt , IM . 2011 . Roitt,s Essential Immunology 12th edition , Hoboken , New Jersey : Blackwell Publishing Ltd .
- Kalinski , P , Giermasz , A , Nakamura , Y , Basse , P , Storkus , WJ , Kirkwood , JM and Mailliard , RB . 2005 . Helper role of NK cells during the induction of anticancer responses by dendritic cells . Mol. Immunol. , 42 : 535 – 539 .