Abstract
Zinc oxide and multi-walled carbon nanotube (ZnO/MWCNT) hybrid nanocomposites were synthesised by microwave-assisted method using the mixed solution of zinc acetate dehydrate (Zn(CH3COO)2·2H2O) and treated MWCNTs. The syntheses were carried out at various microwave irradiation powers. The characterisation of the as-synthesised nanocomposites was conducted by powder X-ray diffraction (XRD) and scanning electron microscopy (SEM). The XRD results revealed that the composites were composed of two phases of MWCNTs and hexagonal wurzite ZnO. The SEM results showed that the ZnO nanoparticles were well decorated on the surface of MWCNTs. The amount of ZnO nanoparticles and their size increased with increasing irradiation power. Thick-film sensors were fabricated onto interdigitated conducting electrodes using as-synthesised hybrid composites as sensing materials. The alcohol-sensing behaviour of the hybrid composite films was investigated. The results indicated that the irradiation power had significant influence on the sensing response of the sensors toward alcohol. The sensor fabricated from the composite synthesised at higher irradiation power exhibited an enhanced alcohol-sensing performance.
1. Introduction
Since their discovery by Iijima in 1991, carbon nanotubes (CNTs) have drawn worldwide attentions because of their novel structural characteristics such as high surface area, high stability and nanorange hollow tubes with a narrow pore-size distribution. Due to their exceptional structural, mechanical, electronic, optical and thermal properties, the CNTs have been utilised as functional materials in various kinds of high-technology applications such as biosensor,[Citation1] supercapacitor,[Citation2] humidity sensors [Citation3] and electron field emitters.[Citation4] To further improve their properties, one of the most effective techniques can be obtained by modifying the functionalisation of the surface of the CNTs with inorganic and metallic low-dimensional materials attached to the surface of CNTs in the form of hybrid composite. Recently, it has been reported that the CNTs properties can be strongly influenced by surface modification and decoration with nanoparticles [Citation5,6] and metal oxide nanostructures.[Citation7,8] As one of the earliest discovered metal oxides, ZnO has been recognised as a widely applied gas-sensing material because of its high mobility of conduction electrons, and good chemical and thermal stability. Typically, the gas-sensing properties of metal oxides depend on their catalytic or surface chemical properties as well as their physical or morphological properties such as grain size [Citation9] and porosity.[Citation10] Meanwhile, the CNTs have been considered as a building block of sensing materials with enhanced performance at low temperature due to their high surface area, excellent chemical stability and adjustable electrical properties.[Citation11] It is believed that novel functionality combined with exceptional properties of ZnO and CNT, especially in the form of low-dimensional composite structures, is expected to emerge. Recently, the metal oxide/CNT-based composites have been synthesised by various methods with remarkable functional properties. Zhu et al. [Citation12] employed a versatile sol–gel technique to coat ZnO nanoparticles on the multi-walled CNTs (MWCNTs) with better photocatalytic performance than pure ZnO nanoparticles. Liu et al. [Citation13] employed a simple chemical route to synthesise SnO2/MWCNTs composite with excellent sensing response and fast recovery to liquefied petroleum gas and ethanol gas. Park et al. [Citation14] reported on the synthesis of one-dimensional ZnO nanostructures on screen-printed CNT films via a thermal chemical vapour deposition using gold nanoparticle as a catalyst. Since the sensing mechanisms of these devices strongly rely on the chemisorption reactions that take place at the surface of the metal oxide, it is believed that sensor sensitivity can be improved by increasing the sensitive surface areas to make contact with the surrounding gases, leading to the enhancement of sensing response of the device. Recently, various techniques have been employed to synthesise functional nanomaterials. However, for economical propose, a facile, effective, low-cost technique without complex equipment to synthesise the hybrid composite is necessary. In this regard, microwave-assisted technique using microwave irradiation as an energy source has been extensively used for the synthesis of wide range of desired materials including nanoparticles,[Citation15] porous materials [Citation16] and nanocomposites.[Citation17] Moreover, microwave can provide significant advantages over conventional heating such as non-contact, instantaneous and rapid heating rate resulting in the reduction of reaction time and uniform heat transfer.
In this work, the synthesis of hybrid ZnO/MWCNTs composites via microwave-assisted method is reported. The effect of microwave irradiation power on the structural properties of as-synthesised composite was characterised by X-ray diffraction (XRD) and scanning electron microscopy (SEM). The alcohol-sensing performance of the composite was conducted and it was found that its sensitivity was profoundly dependent on the microwave irradiation power.
2. Experiment
Zinc acetate dehydrate (Zn(CH3COO)2·2H2O) (AR grade, Ajax Finechem Pty Ltd.) was used as ZnO source. ZnO/MWCNTs composites were prepared by following procedures. Firstly, 6.6 g of Zn(CH3COO)2·2H2O was dissolved in 100 ml deionised water, stirred for 2 h at 100 °C and then cooled down to reach the room temperature. A 3 wt% of MWCNTs with diameter about 20–50 nm was supplied in stock solution. The detail of MWCNTs synthesis was reported elsewhere.[Citation18] Before loading into precursor, a certain amount of MWCNTs was preliminarily treated under ultra violet/ozone for 40 min to modify their surfaces and improve their solubility. The precursor with MWCNTs was consecutively sonicated in ultrasonic bath for 30 min, and then stirred at 180–200 °C for 2 h. Subsequently, the mixture was heated using a household microwave oven with various irradiation powers of 300 W, 450 W and 700 W, and then cooled down to room temperature. After that, as-obtained mixture was annealed at 100 °C for 15 min by heating process until dry composite was obtained. The structural properties of ZnO/MWCNTs composites were examined by XRD (D8 Advance Bruker) with monochromatised Cu Kα (λ = 1.5046 Å) incident radiation. The microstructure of all samples was investigated by field emission-SEM (FE-SEM, Hitachi S-4700).
Before the fabrication of gas sensor, the final composites were dispersed in deionised water and fluorosilicic acid solution, which was used as a binder to form a binding paste. The sensing-element substrate was fabricated using a copper interdigitated electrode for resistance measurement. The ZnO/CNT composite was then screen-printed onto the substrates and the element was finally annealed at 90–100 °C for 1 h in air atmosphere. As-fabricated sensor is schematically depicted in . The electrical resistance measurements of the sensor were carried out in air ambient. The responses of the sensor were studied within the test chamber with an inlet and an outlet. The gas-sensor response (S) for a given gas is typically defined as follows: [Citation19](1)
3. Results and discussion
3.1. Structural characterisation
shows the typical XRD patterns of ZnO/MWCNTs nanocomposites prepared by microwave-assisted method with various irradiation powers. The diffraction peaks located at 2θ = 26.2°, which are observed in all samples, is assigned to (002) d-spacing of the CNTs. The other peaks at 2θ = 44.4° correspond to the face centred cubic structure of nickel catalyst using in the MWCNTs synthesis process. The characteristic peaks of ZnO can be observable as the irradiation power is higher than 300 W. These results imply that the formation of ZnO can be initiated at this point due to sufficient energy supplied to the system by the increasing irradiation power. At power of 450 W and 700 W, the prominent peaks of ZnO situated at 2θ = 31.8°, 34.4°, 36.2°, 47.5° and 56.7° attribute to (100), (002), (101), (102) and (110) orientation planes of ZnO with hexagonal wurtzite structure, respectively. The XRD peak intensity of ZnO becomes stronger and more intense as irradiation power increases, suggesting that the crystallinity of ZnO significantly improves with the increasing irradiation power. The XRD results also suggest that the as-prepared composite is the mixtures of two phases of wurzite ZnO and MWCNTs. The average crystalline size of ZnO can be determined by Scherrer's formula,(2)
Figure 2. The XRD patterns of ZnO/MWCNTs nanocomposites prepared at irradiation powers of 300, 450 and 700 W.
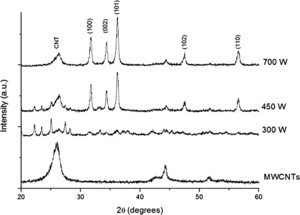
exhibits the morphologies of the as-synthesised composites prepared with irradiation power of 300 W, 450 W and 700 W. From the SEM images, it is clearly observed that, by microwave-assisted technique, ZnO nanoparticles can be formed and thoroughly attached on the MWCNTs surfaces. The amount of ZnO nanoparticles and their size tend to increase with the increasing irradiation power due to the greater amount of supplied energies for the nucleation of ZnO nanoparticles. The particle size of ZnO viewed by the SEM images is in good harmony with the calculated results obtained from the XRD patterns. In addition, ZnO particles preferably agglomerate to generate secondary particle cluster as the irradiation power reaches to 700 W. From all the SEM images, it is suggested that the CNTs can be used as a scaffold to induce the formation of ZnO nanoparticles controlled by microwave irradiation power.
3.2. Gas-sensing characteristics
Alcohol-sensing performance of ZnO/MWCNT-based sensor was conducted and the corresponding results are exhibited in . For comparison, the sensing measurement of pure ZnO nanoparticle-based sensor was also carried out and the corresponding result exhibited insensitiveness of the sensor due to low operating temperature. For ZnO/MWCNTs-based sensor, when alcohol gas was introduced into the chamber, the electrical resistance changed abruptly, reflecting dynamically fast response and good recovery. Moreover, as monitored from the results, the sensing response drastically improves as the irradiation power increases. The corresponding sensing response increases from 20% to 50% as the irradiation power elevates from 300 W to 700 W. This feature may attribute to the fact that the increase in heating provided to the system by increasing the irradiation power can result in the better formation of nanocrystalline ZnO onto the CNT surface, providing greater specific surface area and more active sites to alcohol-sensing reaction. It can be also deduced that ZnO particles play a major role on the sensing activity; meanwhile, the CNTs matrix can act as a scaffold for the formation of ZnO nanoparticles and may assist the electrical charge transfer of the system. This is acknowledged when ZnO particle, which is an n-type semiconductor, is exposed to air, oxygen molecules can adsorb on the surface of the particle or on the grain boundaries, then induce electrons from the conduction band of ZnO and trap the electrons at the surface to form negatively charged, chemisorbed O2− or O22− species. This leads to a band bending and an electron-depleted region so-called space-charge layer in the surface region of the particle. This feature consequently results in a higher surface potential barrier and higher resistance. Under sensing condition, target alcohol gas could favourably experience dehydrogenation reaction as follows:[Citation20](3)
exhibits the dependence of the sensitivity of ZnO/MWCNTs nanocomposite-based sensor response toward alcohol on microwave irradiation power. It is evident that the dynamic-sensing response increases with increasing of the irradiation power. The magnitude of sensitivity drastically heightens from less than 10 to over 50 as the irradiation power increases from 450 W to 700 W. This feature can be understood from the fact that at higher power, greater amount of ZnO particles can be nucleated and larger specific active areas are provided. This presumption is supported by the XRD and SEM results. Further investigation is focused on the response time of the device based on the composite prepared at different irradiation power. Characteristic response times (τ) of the sensors can be obtained by fitting the sensor response using the exponential time dependent equation,(6)
4. Conclusion
The ZnO/MWCNTs nanocomposites were synthesised by microwave-assisted method. The XRD results exhibited that the as-prepared composites are composed of two phases of MWCNTs and ZnO. The SEM results revealed that the amount of ZnO nanoparticles attached on the surface of CNT increased with the increasing irradiation power. The as-prepared composites were utilised as the functional material for alcohol sensing and the performance was investigated. It can be deduced that enhancement of sensing performance of the composite is attained as the irradiation power during the synthesis increases. The possible mechanisms and the role of ZnO and MWCNTs participated in the alcohol sensing are suggested.
Acknowledgements
This work has partially been supported by the National Nanotechnology Center (NANOTEC), NSTDA, Ministry of Science and Technology, Thailand, through its program of Center of Excellence Network. Authors would like to thank Thai Microelectronic (TMEC) for FE-SEM measurement.
References
- Odaci D, Telefoncu A, Timur S. Pyranose oxidase biosensor based on carbon nanotube (CNT)-modified carbon paste electrode. Sens. Actuators B 2008;132:159–165.
- Wie S, Kang WP, Davidson JL, Huang JH. Supercapacitive behavior of CVD carbon nanotubes grown on Ti coated Si wafer. Diam. Relat. Mater. 2008;17:906–911.
- Tsai JTH, Lu CC, Li JG. Fabrication of humidity sensors by multi-walled carbon nanotubes. J. Exp. Nanosci. 2010;5:302–309.
- Rakhi RB, Sethupathi K, Ramaprabhu S. Electron field emitters based on multi-walled carbon nanotubes coated with conducting polymer/metal/metal-oxide composites. J. Exp. Nanosci. 2009;4:67–76.
- Zhang M, Su L, Mao L. Surfactant functionalization of carbon nanotubes (CNTs) for layer-by-layer assembling of CNT multi-layer films and fabrication of gold nanoparticle/CNT nanohybrid. Carbon 2006;44:276–283.
- Zhou L, Gan T, Zheng D, Yan J, Hu C, Hu S. High-density gold nanoparticles on multi-walled carbon nanotube films; a sensitive electrochemical nonenzymatic platform of glucose. J. Exp. Nanosci. 2012;7:263–273.
- Wang X, Zhang F, Xia B, Zhu X, Chen J, Qiu S, Zhang P, Li J. Controlled modification of multi-walled carbon nanotubes with CuO, Cu2O and Cu nanoparticles. Solid State Sci. 2009;11:655–659.
- Wang X, Xia B, Zhu X, Chen J, Qiu S, Li J. Controlled modification of multi-walled carbon nanotubes with ZnO nanostructures. J. Solid State Chem. 2008;181:822–827.
- Deshpande S, Karakoti A, Londe G, Cho HJ, Seal S. Room temperature hydrogen detection using 1-D nanostructured tin oxide sensor. J. Nanosci. Nanotechnol. 2007;7:3354–3357.
- Christoulakis S, Suchea M, Koudoumas E, Katharakis M, Katsarakis N, Kiriakidis G. Thickness influence on surface morphology and ozone sensing properties of nanostructured ZnO transparent thin films grown by PLD. Appl. Surf. Sci. 2006;252:5351–5354.
- Leghrib R, Pavelko R, Felten A, Vasiliev A, Cané C, Gràcia I, Pireaux JJ, Llobet E. Gas sensors based on multiwall carbon nanotubes decorated with tin oxide nanoclusters. Sens. Actuators B 2010;145:411–416.
- Zhu LP, Liao GH, Huang WY, Ma LL, Yang Y, Yu Y, Fu SY. Preparation, characterization and photocatalytic properties of ZnO-coated multi-walled carbon nanotubes. Mater. Sci. Eng. B 2009;163:194–198.
- Liu YL, Yang H-F, Yang Y, Liu Z-M, Shen G-L, Yu RQ. Gas sensing properties of tin dioxide coated onto multi-walled carbon nanotubes. Thin Solid Films 2006;497:355–360.
- Park SS, Lee JM, Yoon SI, Lee DG, Kim SJ, Kim SH, Maeng S, Kim SW. Low-temperature synthesis of one-dimensional ZnO nanostructures on screen-printed carbon nanotube films. Physica E 2008;40:2526–2530.
- Ai LH, Jiang J. Rapid synthesis of nanocrystalline Co3O4 by a microwave-assisted combustion method. Powder Technol. 2009;195:11–14.
- Zhang P, Li GC, Zhang HP, Yang LC, Wu YP. Preparation of porous polymer electrolyte by a microwave assisted effervescent disintegrable reaction. Electrochem. Commun. 2009;11:161–164.
- Hong RY, Pan TT, Li HZ. Microwave synthesis of magnetic Fe3O4 nanoparticles used as a precursor of nanocomposites and ferrofluids. J. Magn. Magn. Mater. 2006;303:60–68.
- Singjai P, Changsarn S, Thongtem S. Electrical resistivity of bulk multi-walled carbon nanotubes synthesized by an infusion chemical vapor deposition method. Mater. Sci. Eng. A 2007;443:42–46.
- Park Y, Dong KY, Lee J, Choi J, Bae GN, Ju BK. Development of an ozone gas sensor using single-walled carbon nanotubes. Sens. Actuators B 2009;140:407–411.
- Xu J, Han J, Zhang Y, Sun Y, Xie B. Studies on alcohol sensing mechanism of ZnO based gas sensors. Sens. Actuators B 2008;132:334–339.
- Alivov YI, Kalinina EV, Cherenkov AE, Look DC, Ataev BM, Omaev AK, Chukichev MV, Bagnall DM. Fabrication and characterization of n-ZnO/p-AlGaN heterojunction light-emitting diodes on 6H-SiC substrates. Appl. Phys. Lett. 2003;83:4719–4721.
- Liu P, Wei Y, Jiang K, Sun Q, Zhang X, Fan S, Zhang S, Ning C, Deng J. Thermionic emission and work function of multiwalled carbon nanotube yarns. Phys. Rev. B 2006;73:235412.