Abstract
The purpose of the present study was to prepare itraconazole-loaded chitosan nanosuspension and evaluate it for ocular delivery. Itraconazole-loaded chitosan nanosuspension was prepared by controlled co-precipitation of chitosan and itraconazole from aqueous acetate solution using a combination of pH change and non-solvent addition. The co-precipitated suspension was evaluated for particle size, zeta-potential, entrapment efficiency and solubility study. It was observed that co-precipitation of itraconazole and chitosan from chitosan–lysine system in the presence of Poloxamer-188 as stabiliser provided nanosuspension of the smallest particle size with a 12-fold increase in aqueous saturation solubility of itraconazole and the fastest in vitro release. Transmission electron micrographs of the nanosuspension showed ovoid-shaped particles. A comparative evaluation of the itraconazole (1%, w/v) nanosuspension with commercial itraconazole suspension (1%, w/v) revealed a significantly higher percentage cumulative permeation of itraconazole across the isolated goat cornea from the nanosuspension dosage form as compared to the commercial suspension (P < 0.01).
1. Introduction
Chitosan, a natural biocompatible, mucoadhesive and biodegradable polymer is obtained by partial deacetylation of chitin. Chitosan and its derivatives have been investigated extensively for a variety of pharmaceutical applications such as delivery of drugs, peptides, vaccines, mucoadhesion of delivery systems at the site of delivery, enhancement of drug penetration or absorption.[Citation1] Chitosan has also been reported to enhance the dissolution rate of poorly water-soluble drugs such as naproxen,[Citation2] aceclofenac [Citation3] and indomethacin.[Citation4] Chitosan has excellent ocular tolerance [Citation5] and has been found to improve the corneal retention and/or penetration of ciclosporin, aciclovir, ofloxacin and indomethacin.[Citation6]
Itraconazole is a triazole anti-fungal agent with a broad spectrum of activity against Malassesia furfur, Candida, Aspergillus and Fusarium species. Itraconazole belongs to class II of the biopharmaceutical classification system (BCS) of drugs, i.e. it has poor solubility but high permeability. In earlier studies, approaches such as solid dispersion,[Citation7] salt formation [Citation8] and cyclodextrin complexation [Citation9] have been investigated for enhancing the dissolution rate of itraconazole.
Itraconazole has been found to be useful for the treatment of fungal keratitis caused by Aspergillosis or Curvularia species.[Citation10] Orally administered (200 mg/day) itraconazole is effective for the treatment of non-severe fungal keratitis but ineffective for severe fungal keratitis due to poor penetration into the ocular tissues.[Citation11] Higher ocular concentrations can be achieved by topical administration of drugs but it requires frequent administration of conventional topical formulations because of limited pre-corneal residence. Itraconazole is commercially available as ophthalmic suspension (1%, w/v). Earlier studies revealed that the vehicle used in the formulation of eye drops affects the corneal penetration of itraconazole,[Citation12] and the efficacy of itraconazole in treatment of corneal ulcers caused by Aspergillus fumigatus was found to be related to its solubility in the vehicle.[Citation13] Recently, ionically gelled chitosan nanoparticles have been explored for pulmonary delivery of itraconazole.[Citation14]
Thus, considering the poor aqueous solubility of itraconazole, promising ocular tolerance and penetration of chitosan and nanosuspension-based formulations prompted us to explore chitosan-based nanosuspension formulations of itraconazole. In this study, chitosan and itraconazole were co-precipitated by controlled addition of non-solvent and pH change. The co-precipitated nanosuspension was evaluated for particle size, solubility, in vitro release and ex vivo corneal permeation study. Further, the nanosuspension was characterised by Fourier-transform infrared (FTIR) spectroscopy, differential scanning calorimetry (DSC), X-ray diffraction (X-RD) and transmission electron microscopy (TEM).
2. Experimental
2.1. Materials
Itraconazole and Poloxamer-188 were obtained as gift samples from Jubilant Organosys Ltd. (Noida, India). Chitosan (MW: 360 kDa, degree of deacetylation: 86%) was generously gifted by Central Institute of Fisheries Technology, (Kochi, India). High performance liquid chromatography (HPLC) grade acetonitrile, glacial acetic acid, diethanolamine, water and lysine were procured from Qualigens Fine Chemicals (Mumbai, India). All other chemicals used were of reagent's grade. Fresh whole eyeballs of goats were obtained from a local butcher shop (Hisar, India).
2.2. Preparation of chitosan–itraconazole nanosuspension
Chitosan–itraconazole nanosuspensions were prepared by nanoprecipitation method.[Citation15] Briefly, itraconazole along with chitosan and/or lysine and with or without Poloxamer-188 () was added to 8 ml of glacial acetic acid under constant stirring, followed by drop-wise addition of equal quantity of water (HPLC grade). Nanoprecipitation of itraconazole from the solution was achieved by controlled addition of aqueous sodium hydroxide solution (1 N). The nanosuspension so obtained was lyophilised in laboratory model freeze dryer (Alpha 2-4 LD Plus, Martin Christ, Germany) for 48 h, at −90°C and at 0.0010 mbar.
Table 1. Composition of various batches of chitosan–itraconazole co-precipitated suspension.
2.3. HPLC analysis
Assay of itraconazole, in samples, was carried out by injecting 20 μl of the solution, spiked with metronidazole (as internal standard), into a chromatographic system equipped with 600 pump controller (Waters), 2487 dual wavelength absorbance UV detector (Waters) and 7725i manual injector (Rheodyne). The chromatographic separation of itraconazole was achieved using mobile phase composition of acetonitrile:water:diethanolamine (70:29.95:0.05, v/v) at a flow rate of 1 ml/min, in an isocratic run through Kromasil 100 (Flexit Jour Lab. Pvt. Ltd., Pune, India), C8 5μ (150 × 4.6 mm i.d.) column. The eluant was monitored for itraconazole at 254 nm. The peak height ratios of itraconazole to metronidazole exhibited linear correlation with the concentration of itraconazole, with the equation of line being Y = 0.023X + 0.002 (R2 = 0.998). The retention time of metronidazole and itraconazole was 1.683 and 4.046 min, respectively. The lowest limit of detection and quantification was 0.2 and 0.8 μg/ml, respectively. The method was found to be precise as the results of intermediate precision and repeatability studies showed relative standard deviation < 2%. Accuracy of the method was established with recovery of 98.4% on commercial eye-drop formulation.
2.4. Characterisation of chitosan–itraconazole nanosuspension
2.4.1. Particle-size and zeta-potential determination
The samples were dispersed in HPLC-grade water and sonicated in an ultrasonicator for 15 min. Particle size and zeta potential were measured using Zetasizer (Malvern Instruments, UK).
2.4.2. Solubility study
Saturation solubility measurements were performed in triplicate using the shake-flask method. Chitosan–itraconazole co-precipitated nanosuspension powders (equivalent to itraconazole-20 mg) were placed in simulated gastric fluid (SGF, 10 ml, pH 1.2). The mixture was then shaken in water bath shaker at 25°C for 48 h. The samples were filtered through a nylon syringe filter (0.45μ) and the concentration of itraconazole dissolved was analysed by HPLC. Solubility of itraconazole bulk was also determined following the same procedure as above.
2.4.3. FTIR spectroscopy
Samples of chitosan–itraconazole co-precipitated nanosuspension (ITN 6), itraconazole, chitosan, Poloxamer-188 and lysine were subjected to FTIR spectroscopy in a FTIR spectrophotometer (Perkin Elmer Spectrum B X-II, USA) in the range of 4400–400 cm−1 as KBr pellets.
2.4.4. Powder X-ray diffraction
Powder X-RD patterns were recorded using a diffractometer (Philips X’PertPRO, Panalytical, Germany). The measurement conditions were: radiation source: Cu Kα; scan type: continuous; scan step size: 0.0170; voltage: 45 kV and current: 40 mA. The scanned range was 5°–50° (2θ).
2.4.5. Differential scanning calorimetry
The thermal characteristics of itraconazole, lysine, chitosan, Poloxamer-188, itraconazole-loaded nanoparticles and physical mixture of itraconazole, lysine, chitosan and Poloxamer-188 were recorded using differential scanning calorimeter (Q10, TA Systems, USA). Samples in the range of 3–5 mg were heated in an aluminium pan and DSC analysis was carried out at a constant nitrogen flow rate of 50 ml/min and at a heating rate of 10°C/min, from 40°C to 250°C.
2.4.6. Transmission electron microscopy
The morphology of the nanoparticles was observed under TEM. A drop of nanosuspension was stratified onto a carbon-coated copper grid and air-dried. Thereafter, the sample was stained with 1% phosphotungstic acid and observed under JEM-1230 transmission electron microscope (JEOL Ltd., Japan) at a voltage of 80 kV.
2.4.7. In vitro release study
In vitro release of itraconazole from various batches of nanosuspension was carried out using 200 ml of pH 1.2 SGF containing sodium lauryl sulfate (0.5%, w/v) as the dissolution medium, employing USP 23 Type II apparatus (TDT 08L, Electrolab, India). Sodium lauryl sulfate was used in the dissolution medium to provide sink conditions.[Citation16] Required quantities of nanoparticles were dispersed in distilled water to prepare nanosuspensions containing itraconazole (1%, w/v). A conventional aqueous suspension containing itraconazole (1%, w/v) was also prepared. To study the in vitro release kinetics of itraconazole from the suspensions, 5 ml of the freshly prepared suspension was placed in a dialysis sac (cut off: 10,000 Da). The sac was immersed with the help of a sinker into 200 ml of SGF at 37°C in a USP dissolution rate test apparatus (TDT 08L, Electrolab, India). The medium was stirred by means of a paddle at 100 rpm. Aliquots of 5 ml sample were withdrawn at various time intervals, filtered using 0.45-μm syringe filter and analysed for itraconazole content using HPLC. The withdrawn samples were replaced with an equal volume of medium.
2.4.8. Entrapment efficiency
The entrapment efficiency (%) of the itraconazole-loaded nanosuspensions was determined indirectly upon separation of the nanoparticles by centrifugation of 10 ml of the nanosuspension prepared by dispersing 25 mg of the lyophilised powder in 10 ml of distilled water at 20,000 rpm (C-24 BL, Remi Equipments Pvt. Ltd, Mumbai, India) for 20 min. The supernatant was treated with glacial acetic acid to dissolve the suspended unentrapped itraconazole and assayed by HPLC method. The entrapment efficiency of itraconazole-loaded nanosuspension was calculated as the ratio of itraconazole loaded into the nanosuspension with respect to the total amount of itraconazole used in the preparation of nanosuspension as follows:(1) where ITZt is the total amount of itraconazole used in the preparation of the nanosuspension and ITZf is the free itraconazole present in the supernatant. All samples were measured in triplicate.[Citation17]
2.5. Preparation of ophthalmic formulation
Required quantity of lyophilised chitosan–itraconazole nanosuspension powder (ITN6) was dispersed in Sorenson's phosphate buffer (pH 7.4) with sonication to prepare itraconazole (1%, w/v) ophthalmic nanosuspension. Benzalkonium chloride was added as the preservative in the concentration of 0.02% (w/v).
2.6. Ex vivo corneal permeation study
The corneal permeation characteristic of the itraconazole nanosuspension (1% w/v) was evaluated comparatively with the commercial itraconazole suspension (1% w/v, ITRAL®, Jawa Pharmaceuticals, India) using the isolated goat cornea model.[Citation18] Whole eyeballs of a goat were transported from the local butcher shop to the laboratory in cold (4°C) normal saline within an hour of slaughtering of the animal. The corneas were carefully excised along with 2–4 mm of surrounding scleral tissue and were washed with cold normal saline until the washing was free from proteins as determined by testing with Folin–Ciocalteu's phenol reagent.[Citation19] Each isolated cornea was mounted by sandwiching the surrounding scleral tissue between clamped donor and receptor compartments of an all-glass modified Franz diffusion cell in such a way that its epithelial surface faced the donor compartment. The corneal area available for diffusion was 0.95 cm2. The receptor compartment was filled with 11 ml of freshly prepared phosphate buffer saline (pH 7.4) and maintained at 35 ± 1°C with constant stirring, using a Teflon-coated magnetic stir bead. One millilitre of ophthalmic formulation was placed on the cornea and the opening of the donor compartment was sealed with a glass cover slip. At appropriate time intervals 1 ml sample was withdrawn from the receptor compartment and analysed for the contents of itraconazole by HPLC method of analysis. Each withdrawn sample was replaced with equal volume of phosphate buffer saline. At the end of the experiment, each cornea (freed from the sclera) was weighed, soaked in 1 ml methanol, dried overnight at 90°C and reweighed. From the difference in weights, corneal hydration was calculated. The study was designed with paired corneas, i.e. one cornea of an animal received commercial itraconazole ophthalmic suspension while the contra lateral cornea received the nanosuspension formulation.
3. Results
shows the results of particle size analysis, zeta potential, entrapment efficiency and solubility study of the various batches of itraconazole nanosuspension. It can be observed from the results that the itraconazole suspensions precipitated from the chitosan and lysine system provided significantly smaller size than the suspensions precipitated from chitosan or lysine system alone. Further, the itraconazole suspensions precipitated in the presence of Poloxamer-188 provided suspension of a significantly smaller size and narrower size distribution than without Poloxamer-188. As reported by Moschwitzer and group, nanoparticles range in size from about 100 to 1000 nm.[Citation20] The formulation ITN6 was found to have average particle size of 289 nm. Formulations ITN1, ITN3 and ITN 5 were suboptimal as the average particle size was above 1000 nm. Furthermore, the saturation solubility of itraconazole in SGF (pH 1.2) was determined to be 3.12 μg/ml at 25°C, while the nansuspensions of itraconazole provided saturation solubility ranging from 11.78 to 38.40 μg/ml.
Table 2. Characterisation of chitosan–itraconazole co-precipitated suspensions.
compares the in vitro release of different batches of the formulated itraconazole nanosuspension with the suspension of the pure drug. It can be observed from the plots that only 43% of the drug dissolved in 120 min from the conventional suspension of itraconazole bulk, while the formulated nanosuspension provided much higher release with 97% of the drug being released from batch ITN6.
Figure 1. In vitro release profiles of conventional suspension and different batches of chitosan–itraconazole co-precipitated suspensions.
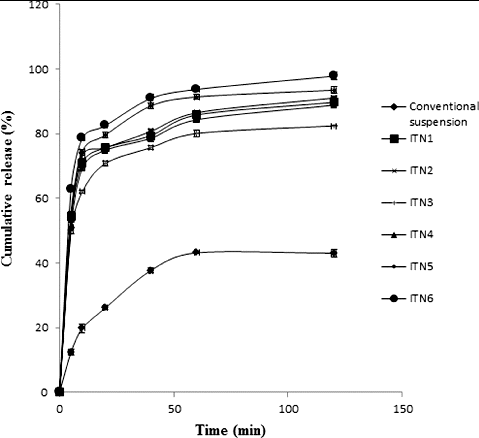
The chitosan–itraconazole nanosuspension of batch ITN6 that provided the particles of the smallest size was further characterised by FTIR, DSC, X-RD and TEM study. The FTIR spectra of itraconazole presented C–Cl stretching at 737 cm−1, triazole ring breathing at 1041 cm−1 and the bands at 1701, 1228 and 821 cm−1 which can be attributed to imine group, asymmetric aralkyl ether and C–H out-of-plane bending for ortho-para benzene substitution, respectively. Spectra of chitosan showed N–H bending vibrations at 1641 cm−1 and O–H stretching at 3435 cm−1, while lysine absorption peaks were observed at 1406 and 2922 cm−1 assigned for symmetric carboxylate stretching and NH3+ stretching, respectively. Poloxamer-188 gave its absorption peaks at 1114 cm−1, assigned for splitting of C–O–C bond due to branching on the carbon atom adjacent to the oxygen atom. Spectra of ITN6 showed N–H bending at 1641 cm−1 and O–H stretching at 3435 cm−1, which has been contributed by chitosan and the contribution of lysine is observed at 1406 cm−1 and 2922 cm−1 assigned for symmetric carboxylate stretching and broad, strong NH3+ stretching band, respectively. The spectra showed splitting of the C–O–C bond due to branching on the carbon atom adjacent to the oxygen atom at 1114.12 cm−1, which has been contributed by Poloxamer-188.
exhibits the DSC thermograms of itraconazole, chitosan, lysine and Poloxamer-188, physical mixture of chitosan, lysine, itraconazole and Poloxamer-188, and the lyophilised chitosan–itraconazole co-precipitated nanosuspension (ITN6). Thermogram of itraconazole showed a sharp endothermic peak characteristic of crystalline substances at 169.9°C with heat of fusion of 90.9 J/g. Thermal curve of chitosan presented a broad endotherm at 99.4°C with heat of fusion of 166.2 J/g. The DSC curve of lysine presented sharp endothermic peaks at 52.7°C and 66.8°C with heats of fusion of 22.3 and 61.03 J/g, followed by a splitting peak comprising of three peaks at 154.1°C, 157.4°C and 161.7°C, with heats of fusion of 10.3, 42.7 and 5.5 J/g, respectively. Thermal curve of lysine also presents a broad endotherm at 227.6°C with heat of fusion of 308.5 J/g. The thermogram of Poloxamer-188 showed peak at 50.85°C with heat of fusion of 139.2 J/g. The physical mixture of itraconazole, Poloxamer-188, chitosan and lysine showed the peak at 51.2°C, which appears to be the overlapping contribution of Poloxamer-188 and lysine, with heat of fusion of 17.21 J/g, and peaks at 65.7°C, 107°C and 166.6°C with heats of fusion of 18.5, 67 and 25.2 J/g, respectively. Thermal curve of chitosan–itraconazole co-precipitated nanosuspension showed endotherm at 48.5°C, 133.3°C, 158.5°C and 219.3°C with heats of fusion of 20, 19.3, 27.2 and 56.3 J/g, respectively.
Figure 2. DSC thermogram of (A) itraconazole, (B) chitosan, (C) poloxamer, (D) lysine, (E) their physical mixture and (F) chitosan–itraconazole co-precipitated nanosuspension (ITN6).
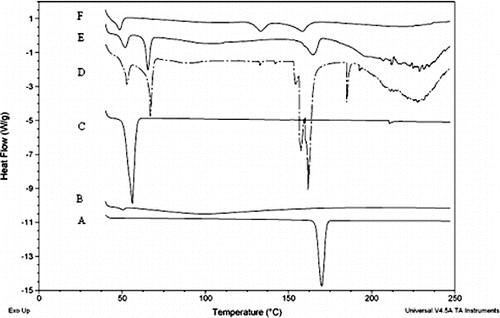
shows the X-RD pattern of chitosan, itraconazole, lysine, Poloxamer-188 and lyophilised itraconazole nanosuspension powder (ITN6). The diffraction pattern of chitosan showed its characteristic peaks at 12.51°, 15.81°, 17.13°, 19.95°, 22.53°, 24.24°, 25.31°, 27.42°, 28.70°, 36.36° and 43.81° (2θ). The diffraction pattern of itraconazole showed the peaks at 8.83°, 10.83°, 14.53°, 17.65°, 18.09°, 19.48°, 20.45°, 21.28°, 22.50°, 23.59°, 25.39°, 31.64° and 37.42° (2θ), while the characteristic peaks of lysine appeared at 8.63°, 10.55°, 15.43°, 17.29°, 19.66°, 20.15°, 21.49°, 23.57°, 24.44°, 25.99°, 28.83°, 31.07°, 30.07°, 37.24° and 41.58° (2θ) in lysine Poloxamer-188, and showed two diffraction peaks at 19.1° and 23.4° (2θ). The diffractogram of lyophilised nanosuspension of itraconazole (ITN6) exhibited the diffraction peaks at 9.01°, 11.71°, 19.37°, 20.72°, 21.83°, 22.72°, 24.47°, 25.27°, 36.87°, 37.31° and 38.51° (2θ), which arise due to the overlapping contribution of chitosan, lysine, Poloxamer-188 and itraconazole. The transmission electron micrograph of the aqueous dispersion of the lyophilised nanosuspension (ITN6) shows the presence of ovoid-shaped particles of uniform morphology ().
Figure 3. X-ray diffractogram of (A) chitosan, (B) itraconazole, (C) lysine, (D) poloxamer and (E) chitosan–itraconazole co-precipitated nanosuspension.
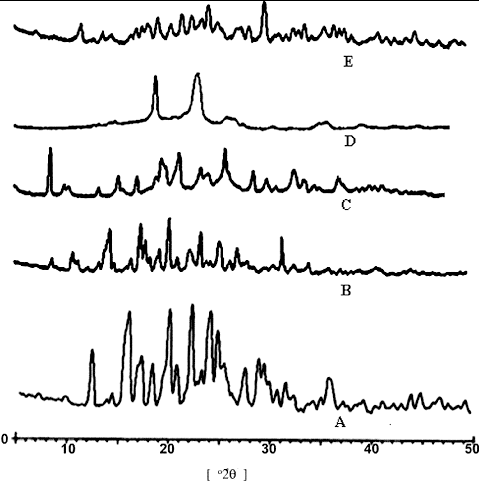
Figure 4. Transmission electron micrograph of aqueous dispersion of chitosan–itraconazole co-precipitated nanosuspension (ITN6).
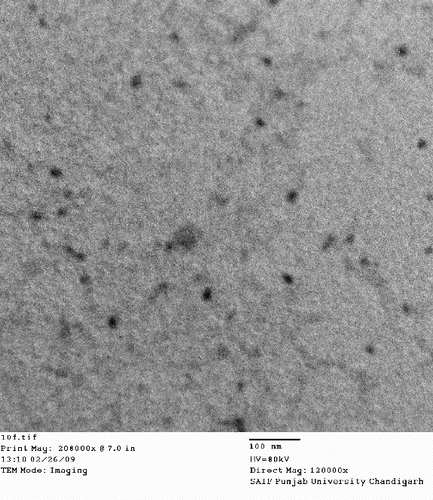
compares the cumulative corneal permeation of itraconazole across the isolated goat cornea from the commercial formulation of itraconazole suspension and the test formulation of itraconazole nanosuspension. The results showed that significantly higher (P < 0.01) amount of itraconazole permeated from the nanosuspension (5.22%) compared to the commercial suspension formulation (3.01%).
Table 3. Cumulative corneal permeation of itraconazole from commercial formulation and chitosan–itraconazole co-precipitated nanosuspension formulation.
4. Discussion
Itraconazole, a triazole anti-fungal agent with pKa of 3.7 has a very low and pH-dependent aqueous solubility (6 μg/ml at pH 1, 0.1 N HCl and 1 ng/ml at neutral pH). It is soluble in dichloromethane; sparingly soluble in tetrahydrofuran; and practically insoluble in water and dilute acidic solutions.[Citation21] Earlier studies reported exceptionally higher solubility of itraconazole in aqueous solutions of volatile organic acids such as aqueous acetic acid and aqueous formic acid.[Citation22] In the present investigation, itraconazole was dissolved in aqueous acetic acid to prepare solutions of higher concentration. The nanoprecipitation of itraconazole from the aqueous acetate solution was achieved by combination of pH change and addition of non-solvent. Chitosan, a cationic mucoadhesive polysaccharide that is insoluble at neutral pH but dissolves in weakly acidic solutions, was also incorporated in the itraconazole solution. The concurrent precipitation of chitosan and itraconazole was accomplished by addition of aqueous alkali to the aqueous acetate solution. Thus, nanoprecipitation of itraconazole from chitosan and/or lysine system followed by lyophilisation will produce matrix systems of itraconazole and chitosan/lysine.
Poloxamer-188, a stabiliser, prevents the agglomeration of smaller particles of higher surface energy. The average particle size and width of particle size distribution (polydispersity index) are the important parameters influencing the saturation solubility, dissolution rate and physical stability of suspensions.[Citation23] For a nanosuspension to be physically stable, it should have a zeta potential of ±20 mV. Since the prepared nanosuspensions have zeta potential of >±20 mV, they are fairly stable. The entrapment efficiency of the various batches of suspension varied from 72% to 91%.
The higher saturation solubility of itraconzole in nanosuspension form to that of bulk drug can be explained on the basis of Ostwald–Freundlich's equation [Citation24]:(2) where Cs – saturation solubility; Cα – solubility of the solid consisting of large particles; σ – interfacial tension; V – molar volume of the particle material; R – gas constant; T – absolute temperature; ρ – density of the solid; and r – radius of the particles. The equation is clearly demonstrating that decrease in particle size corresponds to increase in saturation solubility. Therefore, the faster rate of release from nanosuspensions can be attributed to their smaller size and higher saturation solubility.
The shift in the melting endotherms and the decrease in the heat of fusion of the chitosan–itraconazole co-precipitated nanosuspension in comparison to the respective physical mixtures of components indicates reduction in crystallinity of itraconazole. The diffraction peaks of lower intensity and smaller area observed in the X-RD study indicate decreased crystallinity, validating the results of the DSC study. However, due to overlapping of the diffraction peaks, the relative degree of crystallinity of itraconazole could not be calculated.
The ocular irritation potential of the suspended particles is influenced by the particle size and shape. Particles with sharp angles and edges are more irritating than the isometric particles with obtuse angles and edges.[Citation25] Since the itraconazole nanosuspension particles are ovoid in shape, they are not expected to cause an irritation.
The commercial formulation of itraconazole (ITRAL) contained benzalkonium chloride (0.02% v/v) as preservative and had pH of 7.4. Thus, to compare the corneal permeation characteristics of the itraconazole from the nanosuspension, an ophthalmic dosage form was formulated using the lyophilised chitosan–itraconazole nanosuspension (ITN6), in phosphate buffer saline of pH 7.4 and containing benzalkonium chloride (0.02% v/v) as preservative.
It can be observed that the nanosuspension formulation provided a 1.73-fold higher corneal permeability of itraconazole than the conventional commercial suspension dosage form. The enhanced ex vivo permeation from the nanosuspension formulation can be attributed to smaller particle size of the formulation as compared to the conventional commercial formulation (having size of 8676 nm). Previous findings have reported that the higher corneal permeation of drugs from the nanosuspension-based formulations can be ascribed to the direct endothelial uptake of nanosuspension through ocular cells. Furthermore, availability of greater amounts of drug in solution form in nanosuspension increases the corneal permeation as compared to conventional suspension.[Citation26] Thus, based on ex vivo corneal permeation characteristics, the nanosuspension-based formulation appears to be the most promising drug delivery strategy.
5. Conclusion
Itraconazole-loaded chitosan nanosuspension was prepared by inexpensive, scalable, nanoprecipitation method. The suspension prepared with lysine and chitosan resulted in smaller particle size as compared to the suspension prepared with lysine or chitosan. Further addition of Poloxamer-188 provided a significant decrease in particle size than the formulation without Poloxamer-188. The nanosuspension afforded a 12-fold increase in aqueous saturation solubility of itraconazole. Further, the nanosuspension of itraconazole offered higher corneal permeability as compared to the commercially marketed ophthalmic suspension of itraconazole. Thus, the nanosuspension of itraconazole could be a satisfactory approach for designing an ophthalmic formulation with enhanced solubility. However, further in vivo studies are needed to comment on the therapeutic usefulness of nanosuspension in the management of fungal infections of eye.
Acknowledgements
The authors express gratitude to SAIF, Punjab University, Chandigarh for X-Ray diffraction and TEM analysis.
References
- Illum L. Chitosan and its use as a pharmaceutical excipient. Pharm Res. 1998;15:1326–1331.
- Zerrouk N, Mennini N, Maestrelli F, Chemtobm C, Mura P. Comparison of the effect of chitosan and polyvinylpyrrolidone on dissolution properties and analgesic effect of naproxen. Eur J Pharm Biopharm. 2004;57:93–99.
- Mutalik S, Anju P, Manoj K, Usha AN. Enhancement of dissolution rate and bioavailability of aceclofenac: a chitosan-based solvent change approach. Int J Pharm. 2008;350:279–290.
- Park KM, Bae JW, Joung YK, Shin JW, Park KD. Nanoaggregate of thermosensitive chitosan-Pluronic for sustained release of hydrophobic drug. Colloids Surf B Biointerfaces. 2008;63:1–6.
- Felt O, Furrer P, Mayer JM, Plazonnet B, Buri P, Gurny R. Topical use of chitosan in ophthalmology: tolerance assessment and evaluation of precorneal retention. Int J Pharm. 1999;180:185–193.
- Alonso MJ, Sanchez A. The potential of chitosan in ocular drug delivery. J Pharm Pharmacol. 2003;55:1451–1463.
- Verreck G, Six K, Van den Mooter G, Baert L, Peeters J, Brewster ME. Characterization of solid dispersions of itraconazole and hydroxypropyl methylcellulose prepared by melt extrusion – part I. Int J Pharm. 2003;251:165–174.
- Tao T, Zhao Y, Wu J, Zhou B. Preparation and evaluation of itraconazole dihydrochloride for the solubility and dissolution rate enhancement. Int J Pharm. 2009;367:109–114.
- Hasan HA, Al-Marzouqi AH, Jobe B, Hamza AA, Ramadan GA. Enhancement of dissolution amount and in vivo bioavailability of itraconazole by complexation with β-cyclodextrin using supercritical carbon dioxide. J Pharm Biomed Anal. 2007;45:243–250.
- Kalavathy CM, Parmar P, Kaliamurthy J, Philip VR, Ramalingam MDK, Jesudasan CAN, Thomas PA. Comparison of topical itraconazole 1% with topical Natamycin 5% for the treatment of filamentous fungal keratitis. Cornea. 2005;24:449–452.
- Savani DV, Perfect JR, Cobo LM, Durack DT. Penetration of new azole compounds into the eye and efficacy in experimental Candida endophthalmitis. Antimicrob Agents Chemother. 1987;31:6–10.
- Guzek JP, Roosenberg JM, Gano DL, Wessels IF. The effect of vehicle on corneal penetration of triturated ketoconazole and itraconazole. Ophthalmic Surg Lasers. 1998;29:926–929.
- Martinez-R M, Claros-B JA, Vale-O MA, Siso-V E, Padilla R, Santiago A, Simon JA. Effect of the vehicle on the topical itraconazole efficacy for treating corneal ulcers caused by Aspergillus fumigates. Clin Experiment Ophthalmol. 2008;36:335–338.
- Jafarinejad S, Gilani K, Moazeni E, Ghazi-Khansari M, Najafabadi AR, Mohajel N. Development of chitosan-based nanoparticles for pulmonary delivery of itraconazole as dry powder formulation. Powder Technol. 2012;222:65–70.
- Fessi HC, Devissaguet JP, Puisiex F, Thies C. Process for the preparation of dispersible colloidal systems of a substance in the form of nanoparticles. US Patent 5, 118, 528. 1992.
- Sinswat P, Gao X, Yacaman MJ, Williams RO, Johnston KP. Stabilizer choice for rapid dissolving high potency itraconazole particles formed by evaporative precipitation into aqueous solution. Int J Pharm. 2005;302:113–124.
- Sharma R, Ahuja M, Kaur H. Thiolated pectin nanoparticles: preparation, characterization and ex vivo corneal permeation study. Carbohydr Polym. 2012;87:1606–1610.
- Yadav M, Ahuja M. Preparation and evaluation of nanoparticles of gum cordia, an anionic polysaccharide for ophthalmic delivery. Carbohydr Polym. 2010;81:871–877.
- Malhotra M, Majumdar DK. Aqueous, oil and ointment formulations of ketorolac: efficacy against the prostaglandin E2-induced inflammation and safety – a technical note. AAPS Pharm Sci Tech. 2006;7:E99–E104.
- Moschwitzer J, Achleitner G, Pomper H, Muller RH. Development of an intravenously injectable chemically stable aqueous omeprazole formulation using nanosuspension technology. Eur J Pharm Biopharm. 2004;58:615–619.
- Moffat AC, Osselton DM, Widdop B. Clarke's analysis of drugs and poisons. London (UK): Pharmaceutical Press; 2004.
- Kovacs K, Stampf G, Klebovich I, Antal I, Ludanyi K. Aqueous solvent system for the solubilization of azole compounds. Eur J Pharm Sci. 2009;36:352–358.
- Patravale VB, Date AA, Kulkarni RM. Nanosuspensions: a promising drug delivery strategy. J Pharm Pharmacol. 2004;56:827–840.
- Mosharraf M, Sebhatu T, Nystrom C. The effects of disordered structure on the solubility and dissolution rates of some hydrophilic, sparingly soluble drugs. Int J Pharm. 1999;177:29–51.
- Malhotra M, Majumdar DK. Permeation through cornea. Ind J Exp Biol. 2001;39:11–24.
- Calvo P, Vila-Jato JL, Alonso MJ. Comparative in vitro evaluation of several colloidal systems, nanoparticles, nanocapsules, and nanoemulsions, as ocular drug carriers. J Pharm Sci. 1996;85:530–536.