Abstract
The primary aim of the study was to observe the morphological changes taking place on Escherichia coli when treated with non-cationised placebo lipidic emulsion (NCPLE) and cationised placebo lipidic emulsion (CPLE). The particle size of NCPLE and CPLE indicated the mean diameter of 10.77 ± 0.20 nm and 56.98 ± 1.718 nm, respectively. The zeta potential of NCPLE and CPLE depicted the potential of −10.8 ± 0.80 mV and +20.9 ± 3.25 mV, respectively. Agar diffusion study indicated higher inhibition zone of 21.4 ± 0.85 mm for CPLE as evaluated against 14.7 ± 0.76 mm for NCPLE. Transmission electron micrographs of Escherichia coli treated with NCPLE and CPLE showed the potential of blank nanoemulsions as antibacterial, which can be used as a therapeutic agent. The bacterial cell being negatively charged attracts the cationised nanoemulsions from the bulk of dispersion. As the concentration of nanoemulsion increased in the vicinity of the bacterial cell, the degree of collisions increased between the cationised nanoemulsion leading to coalescence and the formation of a pool of emulsion containing bacterial cells leading to cell death. The study demonstrated that placebo lipidic nanoemulsion (NCPLE & CPLE) may itself be used as an active therapeutic agent for the treatment of bacterial infections. Moreover, it can also be used as a carrier system for antibacterial drugs for the two-pronged synergistic effect.
1. Introduction
There are several methods to evaluate the impacts of antimicrobial treatments on bacterial populations. Normally, this is conducted using proper selection of microbiological plating and techniques for detection, identification and enumeration of the bacterial population to ensure that a correct count of the microorganism is reported.[Citation1] Although enumeration of bacterial populations before and after treatment provides information on the treatment effect on viability, limited information is available on the type or extent of morphological or physical damage that occur to the bacterial cells. Visual information is extremely useful in providing insight into the microstructure of the cell. It can also assist in characterising the type and magnitude of changes that occur to the cell composition in response to the treatment, and it enhances our understanding of how and why a given treatment if effective/non-effective against a particular microorganism.[Citation2]
There is considerable interest in developing new strategies to combat bacterial infections because of the increasing occurrence of clinical cases caused by drug-resistant bacteria for which traditional antibiotic therapy becomes ineffective.[Citation3] An important aspect of the effort to develop novel antimicrobial strategies is the involvement of lipids in several different contexts. In this pretext, lipid-based nanoemulsions could perform a considerable role in combating the mounting emergence of drug-resistant bacteria.
Lipidic nanoemulsions are thermodynamically stable, transparent and isotropic dispersions consisting of lipid and water, stabilised by an interfacial film of surfactant molecules, typically in conjunction with a cosurfactant.[Citation4] There are a limited number of reports in the literature on microemulsion use for antimicrobial purposes. It was reported that an oil in water (o/w) microemulsion as pharmaceutical preparations gave a 5 log reduction in the numbers of Staphylococcus aureus or Pseudomonas aeruginosa in only 45 seconds,[Citation5] and effectively reduced the viability of an established biofilm population of Pseudomonas aeruginosa.[Citation6] In most of the studies, the antimicrobial drug-loaded nanoemulsions were evaluated for their suitability as antimicrobial therapy. However, no pharmaceutical products containing lipids as active compounds have yet been approved for clinical use as prophylactic or therapeutic drugs against bacterial and viral infections, even after the proven antimicrobial activities of lipids.[Citation7] Apparently, the great accomplishment of chemotherapy using synthetic compounds has discouraged researchers and the pharmaceutical industry in making serious efforts to develop drugs containing simple natural compounds. However, this may now be changing with the increasing problem of bacterial and viral resistance to antibiotic and antiviral drugs. It has become apparent to some microbiologists that in addition to the treatment with synthetic drugs, which inhibit the replication of pathogenic organisms, there may be a place for lipids as antimicrobial agents that may kill pathogens, either before they infect at the site of entry, such as skin or mucosal membranes, or after an infection has occurred.[Citation7]
Under this manoeuvre, we have prepared a placebo cationised and non-cationised lipidic nanoemulsion and carried out antibacterial activities against an opportunistic bacterium Escherichia coli. The lipidic nanoemulsion was characterised for their particle size analysis and zeta potential using photon correlation spectroscopy. Finally, the exact mechanism of antimicrobial activity presented by lipidic nanoemulsion (cationised and non-cationised) was elucidated by transmission electron microscopy. The present study focuses and signifies the importance of placebo lipidic nanoemulsion as antibacterial that can be exploited to be delivered for clinical use as prophylactic or therapeutic dosage forms. It was decided to study the antibacterial studies of lipid-based nanoemulsions without any drug loading so that it may be used as a carrier system to ferry the antimicrobial drugs in future and may be used as a therapeutic agent by itself.
2. Materials and methods
Glyceryl mono caprylate (GMC) and caprylocaproyl polyoxyl-8 glycerides NF (CPG) was provided ex gratis by Abitec Corporation (Janesville, Germany) and Gattefosse (Saint-Priest, Cedex, France), respectively. Cremophor RH40 (CrRH40) was a generous gift from BASF (Ludwigshafen, Germany). Oleyl amine (OA) was purchased from Fluka (Steinheim, the Netherlands), Escherichia coli (MTCC-1678) was purchased from Microbial Type Culture and Gene Bank (IMT, Chandigarh, India). Escherichia coli were maintained in Nutrient Agar (NA) at pH 7.0 and were revived every 20 days in fresh NA media. All other chemicals and reagents were AnalaR grade and were used as such.
2.1 Preparation of lipidic emulsions
Non-cationised placebo lipidic emulsion (NCPLE) was prepared by taking a mixture of GMC (500 mg), CPG (500 mg) and CrRH40 (1000 mg) in the ratio of (1:1:2). The weight ratio of GMC, CPG and CrRH40 was taken in a pre-sterilised beaker (100 mL) and heated on a water bath with continuous stirring till an isotropic mixture was obtained. The pre-warmed Millipore water (100 mL) maintained at the same temperature was added slowly with continuous stirring to get a clear NCPLE. The cationised placebo lipidic emulsion (CPLE) (100 mL) was prepared by a similar procedure by adding 0.5% w/w OA in an isotropic mixture of GMC, CPG and CrRH40.
2.2 Characterisation of lipidic emulsion
The mean particle size (MPS) and zeta-potential (ZP) of NCPLE and CPLE (100 mL) was determined by the dynamic light scattering (DLS) technique using Zetasizer (Nano ZS; Malvern Instruments, UK) using He–Ne red laser, 4.0 mW, 633 nm; temperature, 25°C; refractive index, 1.333; or with adjustment if needed. All measurements were in triplicates, done using disposable polystyrene cuvettes (Malvern Instruments, UK).
2.3 Solid medium diffusion method
The solid medium diffusion procedure using wells in plates was used to determine the antibacterial activity of NCPLE and CPLE. Petri plates (90 mm diameter) (Tarson, India) were prepared by pouring 25 mL sterile nutrient agar (NA) and allowed to solidify. Plates were dried and 500 μL of an inoculum suspension (5.5 × 106 cfu/mL) was poured and uniformly spread. After the inoculum's absorption by agar, wells were made using sterile tubes (diameter 5 mm), which were filled with 100 μL of the NCPLE and CPLE. The plates were incubated in an incubator at 37°C for 24 hr. The negative control used in this method was sterile water for injection. The inhibition zones were measured in millimetres using vernier callipers. Each assay in this experiment was carried out in triplicates.
2.4 Transmission electron microscopy studies
Transmission electron microscopy (TEM) study was conducted to elucidate the microstructure of NCPLE, CPLE and to study the morphological changes on the bacterial cell when it is treated with NCPLE and CPLE using TEM (Tecnai12, 120 KV, FEI Company, the Netherlands). Bacterial cell dispersions (5.0 mL) were treated by NCPLE (1.0 mL) and CPLE (1.0 mL) followed by incubation for 30 minutes. After 30 minutes of contact a drop of treated bacterial specimen, NCPLE and CPLE, was spread on a copper grid coated with a carbon film and excess droplets were instantly removed using a filter paper. Next, a drop of 2% w/v phosphotungstic acid solution was dripped on the copper grid. After 60 seconds of contact, an excess of solution was then removed. TEM was conducted by a negative staining method. The grid was then dried in the air at ambient temperature before loading in the microscope.[Citation8,9]
3. Results and discussion
The primary aim of the study was to expound the morphological changes taking place on Escherichia coli when treated with NCPLE and CPLE. In the recent past it has become evident that in addition to treatment with synthetic drugs, there may be place for microbicidal compounds like lipids to kill pathogens. It may thus be reasonable to use a bilateral attack on the invading pathogen with lipid-based emulsion (LPE) and antibiotics loaded in LPE acting in concert. In the present study it was decided to study the anti-bacterial studies of NCPLE and CPLE without any drug loading that may be used as a carrier system to ferry antimicrobial drugs in future.
3.1 Mean particle size and zeta potential studies
Particle size frequency curve of NCPLE () and CPLE () indicates a mean particle diameter of 10.77 ± 0.20 nm () and 56.98 ± 1.718 nm (), respectively. It is reasonable to detect a marginal increase in mean particle diameter in CPLE as OA added to induce cationisation is insoluble in the aqueous phase. Moreover, it is expected that OA will form a cationised layer on the o/w nanoemulsions. The layer formed on the interface may lead to a marginal increase in the mean particle size of CPLE. The frequency curve of zeta potential of NCPLE () and CPLE () depicts the potential of −10.8 ± 0.80 mV and +20.9 ± 3.25 mV, respectively. NCPLE showed negative potential because of orientation of fatty acids present in GMC and CPG at the interface of oil in water nanoemulsions. The present finding is in agreement with results reported by Singh et al., 2010.[Citation9] The zeta potential of CPLE was found to be positive (+20.9 ± 3.25 mV) () because OA has successfully imparted cationisation to the nano droplets.
Table 1. The inhibition zones (mm) of nanoemulsions against E. coli along with their mean particle size and zeta potential.
Figure 1. Frequency curves with oversized curve of non-cationised placebo lipidic emulsion (NCPLE); mean particle size: 10.77 ± 0.20 nm; n = 3.
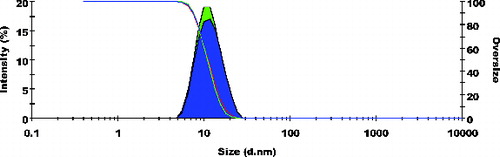
Figure 2. Frequency curves with oversized curve of cationised placebo lipidic emulsion (CPLE); mean particle size: 56.98 ± 1.718 nm; n = 3.
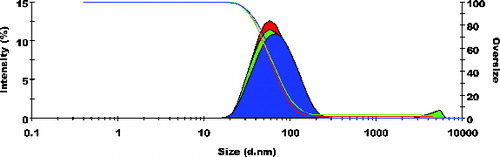
3.2 Solid medium diffusion study
The zone of inhibition assay for NCPLE and CPLE is tabulated in . A comparison assay indicates higher inhibition zone of 21.4 ± 0.85 mm for CPLE as evaluated against 14.7 ± 0.76 mm for NCPLE. This effect was well anticipated as E. coli is a gram-negative bacteria and both the outer leaflets of the plasma membrane as well as the outer membrane contain anionic molecules orienting towards the exterior of the cell.[Citation10] This leads to a negative charge on the bacterial surface. The CPLE being cationic in nature (+20.9 ± 3.25 mV) may lead to better electrostatic adhesion between positively charged emulsion and negatively charged bacterial surface. The enhanced adhesion would direct a larger zone of inhibition (21.4 ± 0.85 mm) for CPLE as compared to NCPLE (14.7 ± 0.76 mm). To further understand the mechanism of the microbicidal effect of NCPLE and CPLE, transmission electron microscopic study was carried out.
3.3 Transmission electron microscopic studies
TEM images of NCPLE and CPLE are shown in a–c. It could be seen that spherical nanoemulsions were formed (shown by arrows) with no signs of coalescence indicating the stability o/w nandroplets. The TEM image of NCPLE indicates that most of the droplets have particle size less than 25 nm in agreement with the DLS analysis where the mean particle size was found to be 10.77 ± 0.20 nm. The particle size of CPLE obtained from the TEM analysis (b and c) is also in close agreement with the DLS analysis. A closer analysis of the TEM image of CPLE (b) and the magnified area of the identified region (c) reveals that most of the globule is surrounded by a thick layer (see the region between broken arrow of c). We can hypothesise that the formed thick layer is of OA that provides a cationic charge to the nanoemulsions (zeta potential: +20.9 ± 3.25 mV). This assumption is based on the finding of PCS experiment where we have observed that the mean particle size of CPLE was 56.98 ± 1.718 nm as compared to 10.77 ± 0.20 nm of NCPLE. The increase in mean particle size can hence be attributed to the presence of a thick layer of OA in CPLE.
Figure 5. Transmission electron microscopy of non-cationised placebo lipidic emulsion (NCPLE) (a); cationised placebo lipidic emulsion (CPLE) (b) along with the magnified portion of (CPLE) (c). Broken black arrows indicate the spherical nanodroplets. The region within the broken red arrows indicates the thickness of the oleylamine layer.
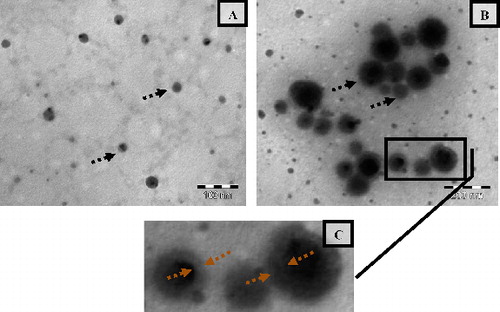
The TEM image of untreated E. coli cells is depicted in . The E. coli cells appeared normal rod shaped with intact intracellular components. The cell wall appeared compact and complete with smooth surfaces. a–c indicates the representative TEM images of E. coli cells treated with NCPLE from the same copper grid. A closer analysis of a–c indicates the presence of numerous nanoemulsions in the vicinity of E. coli (shown by black arrows) as well as on the bacterial surface. The bacterial surface appeared rough, constricted with broken cell wall at many places (shown by blue and green broken arrows). Besides a roughening of the membranes, all the cells showed irregular shaped outer membranes and enlargement of the periplasmic space. At some points, the outer membrane appeared to be broken in such a way that the inner and outer membranes were fused (see the broken arrow in a) leading to the release of vital cellular contents and finally cell death. A similar structural observation on E. coli was also reported by Van der Kraan et al. [Citation11] when treated with bLF peptides.
Figure 6. Transmission electron micrographs of untreated E. coli cells. CW = cell wall; PM = plasma membrane.
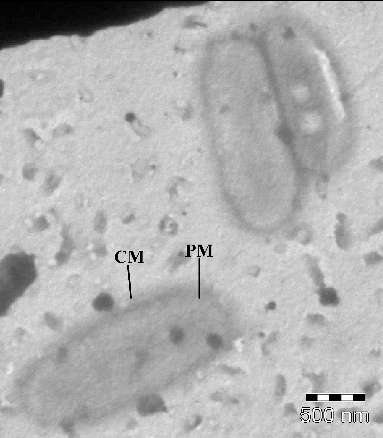
Figure 7. Transmission electron micrograph of E. coli treated with NCPLE. The images are taken from the same copper grid from different regions (a–c). Black arrows indicate the nanoemulsions present in the vicinity of bacterial cells. Black broken arrows indicate the fusion of outer and inner membranes leading to cell leakage. Blue broken arrows indicate the surface roughness of bacterial cells. Green broken arrows indicate the rupture of cell membrane.
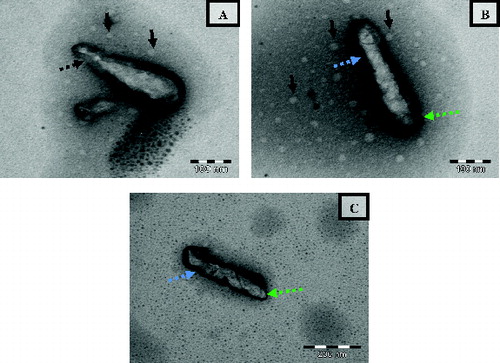
a–e portrays the collated TEM images of E. coli cells at different levels of injury when treated with CPLE from the same copper grid. A closer analysis of a–e indicates the presence of numerous nanoemulsions in the vicinity of E. coli (shown by black arrows) as well as on the bacterial surface. The concentration of nanoemulsion in the vicinity of bacterial cells appears to be abundant as compared to NCPLE-treated E. coli cells. This might be because of electrostatic attraction between negatively charged cell surface and positively charged nanoemulsion (CPLE). The finding further suffices the disk diffusion study where a zone of inhibition was found to be more in CPLE-treated cell as compared to NCPLE-treated cells. Further analysis of a–c will reveal an interesting finding where E. coli is confined to a pool of coalesced emulsion. The process of coalescence can be observed at the circumference of the pool (shown by curve arrows). Such a process of coalescence is not observed in other areas away from E. coli. The finding can be explained as follows: the bacterial cell being negatively charged attracts the cationised nanoemulsions from the bulk of dispersion. As the concentration of the nanoemulsion increases in the vicinity of the bacterial cell, the degree of collisions increases between the cationised nanoemulsion leading to coalescence and formation of pool of emulsion containing bacterial cell. This finding has not been reported by earlier researchers as far as we believe. In agreement with the finding of E. coli treated with NCPLE the bacterial surface appeared rough, constricted with broken cell wall at many places (shown by black broken arrows) when treated with CPLE. The cells showed irregular-shaped outer membranes with an enlargement of periplasmic space. Such phenomena of enlarged periplasmic space can be seen in e. At some points, the outer membrane was broken in such a way that the vital cytoplasmic contents exudates and finally cell death occurs. Such phenomena can be seen in d where a dividing cell has lost its outer and inner membrane (shown by a black broken arrow) leading to the release of cytoplasmic content (shown by a red broken arrow) leaving a ghost cell. It may thus be construed that the CPLE has tremendous potential to show antibacterial activity and can be considered in therapeutic dosage form.
Figure 8. Transmission electron micrograph of E. coli treated with CPLE. The images are taken from the same copper grid from different regions (a–c). Black unbroken arrows indicate the nanoemulsions present in the vicinity of bacterial cells. Black broken arrows indicate the disintegration of the cell wall. Curved arrows indicate the coalescence on nanodroplets with the circumference of the pool of emulsion containing E. coli. The red arrow indicates the cytoplasmic content exudated out from the cell after treatment. PM = Perplasmic space.
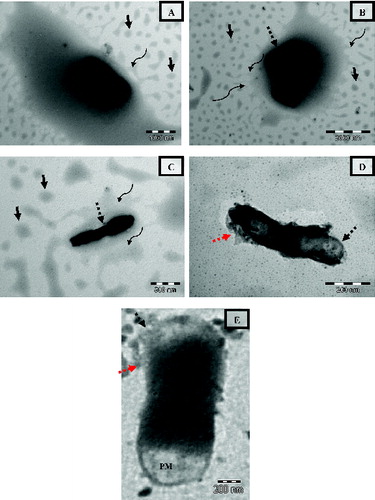
The present finding illustrates the significance of blank lipidic emulsion (NCPLE and CPLE) not only as a carrier system for antimicrobial drugs but also as a therapeutic agent. The lethal effect of antimicrobial drugs can be increased manifold if such drugs are delivered by lipidic emulsion. Moreover, the dose of the drug can also be reduced to a significant level leading to less dose burden and lesser chances of microbial drug resistance.
4.4 Conclusions and future scope
The primary aim of the study was to see the morphological changes taking place on Escherichia coli when treated with NCPLE and CPLE. In the recent past it has become evident that in addition to treatment with synthetic drugs, there may be place for microbicidal compounds like lipids to kill pathogens. It may thus be reasonable to use a bilateral attack on the invading pathogen with lipid-based emulsion (LPE) and antibiotics loaded in LPE acting in concert. In the present study it was decided to study the antibacterial studies of NCPLE and CPLE without any drug loading so that it may be used as a carrier system to ferry the antimicrobial drugs as well as a therapeutic agent in future. The particle size of NCPLE and CPLE indicated the mean diameter of 10.77 ± 0.20 nm and 56.98 ± 1.718 nm, respectively. The zeta potential of NCPLE and CPLE depicted the potential of −10.8 ± 0.80 mV and +20.9 ± 3.25 mV, respectively. The agar diffusion study indicated a higher inhibition zone of 21.4 ± 0.85 mm for CPLE as evaluated against 14.7 ± 0.76 mm for NCPLE. TEM images of E. coli treated with NCPLE and CPLE showed tremendous potential of blank nanoemulsions that can be used as therapeutic agents. The bacterial cell being negatively charged attracts the cationised nanoemulsions from the bulk of dispersion. As the concentration of the nanoemulsion increased in the vicinity of the bacterial cell, the degree of collisions increased between the cationised nanoemulsion leading to coalescence and formation of a pool of emulsion containing bacterial cells leading to cell death. The potential of explored nanoemulsions (NCPLE and CPLE) as a drug carrier system to ferry antibacterial drugs will be explored in future. Moreover, the extent to which the loading dose of an antibacterial drug can be reduced remains unexplored.
Declaration of interest
Authors report no conflict of interest. The authors alone are responsible for the contents and the writing of the paper.
Acknowledgements
This research work was financially supported by University Grants Commission, Government of India, vide sanction letter number ‘41-1423/2012 (SR)’, dated 30 July 2012, granted to Mrs Neeru Singh.
References
- Kang DH, Fung DYC. Thin agar layer method for recovery of heat-injured Listeria monocytogenes. J Food Prot. 1999;62:1346–1349.
- Hajmeer M, Ceylan E, Marsden JL, Fung DYC. Impact of Sodium chloride on Escherichia coli O157:H7 and Staphylococcus aureus analyzed using transmission electron microscopy. Food Microb. 2006;23:446–452.
- Epand RF, Mor A, Epand RM. Lipid complexes with cationic peptides and OAKs; their role in antimicrobial action and in the delivery of antimicrobial agents. Cell Mol Life Sci. 2011;68:2177–2188.
- Zhang H, Cui Y, Zhu S, Feng F, Zheng X. Characterization and antimicrobial activity of a pharmaceutical emulsion. Int J Pharm. 2010;395:154–160.
- Al-Adham ISI, Khalil E, Al-Hmound ND, Kierans M, Collier PJ. Microemulsions are membrane-active, antimicrobial, self-preserving systems. J Appl Microbiol. 2000;89:32–39.
- Al-Adham ISI, Al-Hmound ND, Khalil E, Kierans M, Collier PJ. Microemulsions are highly effective anti-biofilm agents. Lett Appl Microbiol. 2003;36:97–100.
- Thormar H, Hilmarsson H. The role of microbicidal lipids in host defense against pathogens and their potential as therapeutic agents. Chem Phy Lipids. 2007;150:1–11.
- Singh SK, Verma PRP, Razdan B. Glibenclamide-loaded self-nanoemulsifying drug delivery system: Development and characterization. Drug Dev Ind Pharm. 2010;36:933–945.
- Singh SK, Verma PRP, Razdan B. Development and characterization of a lovastatin loaded selfmicroemulsifying drug delivery system. Pharm Dev Technol. 2010;15:469–483.
- Junior AS, Teschke O. Dynamics of the antimicrobial peptide PGLa action of Escherichia coli monitored by atomic force microscopy. World J Microbiol Biotech. 2005;21:1103–1110.
- Van der Kraan MIA, Marle JV, Nazmi K, Groenink J, Van't Hof W, Veerman ECI, Bolscher JGM, Amerongen AVN. Ultrastructural effects of antimicrobial peptides from bovine lactoferrin on the membranes of Candida albicans and Escherichia coli, Peptides. 2005;26:1537–1542.