Abstract
Indigenously available natural dyes have been used as natural sensitisers for dye-sensitised solar cells (DSSCs) in this study. The chlorophyll extract from Azadirachta indica (Neem) leaves and anthocyanin extract from poinsettia bracts were obtained using ethanol. Nanoparticles of TiO2 having an average size of 80 nm were deposited on fluorine-doped tin oxide (FTO) coated glass using the electrophoretic deposition method. Counter electrode was prepared by spraying conductive graphite over FTO glass substrates. A sandwich-type cell was assembled, and the fill factor and the power conversion efficiency were measured under a light source simulating AM 1.5 with an intensity of 100 mW/cm2. DSSCs containing chlorophyll and anthocyanin exhibited open circuit voltages of 404 and 406 mV with fill factors of 40.1% and 45.8%, respectively. The mixture of the two natural dyes as sensitiser for DSSCs gave the best photovoltaic conversion efficiency of approximately 1%.
1. Introduction
Dye-sensitised solar cell (DSSC) is a photoelectrochemical device invented by Michael Gratzel.[Citation1] Conventional silicon solar cells convert sunlight into electricity using the photovoltaic effect that exists at semiconductor p–n junctions. The two processes of light absorption and the separation of the electric charges formed as a consequence of that absorption are performed simultaneously. On the other hand, DSSCs work on a principle wherein the processes of light absorption and charge separation are differentiated. The working photoelectrode of DSSC is made of transparent conducting oxide substrate and a wide band gap semiconductor film on which dye molecules are adsorbed. Photoexcited dye electrons are transferred to the semiconductor film and then they flow to the counter electrode through an external circuit, oxidising the electrolyte and completing the cycle. DSSCs can be assembled at room temperature by a simple and easy manufacturing process and the costs involved are much less than silicon-based photovoltaic devices.
The sensitiser has an important role in the functioning of a DSSC and it mainly defines its performance. The dyes with a broader absorption range and higher molar absorption coefficients are required for efficient light harvesting with TiO2 films. All the high-efficiency DSSC devices are based on expensive synthetic dyes, such as N-719, N-749 and N-3.[Citation2–4] These dyes based on ruthenium polypyridyl complexes are costly and harmful to the environment, thus forcing the researchers to look for alternatives. Researchers are experimenting with low-cost and eco-friendly alternatives to ruthenium-based dyes.[Citation5–7]
DSSCs derive inspiration from photosynthesis, by which green plants convert sunlight into food. Many leaves, flowers and fruits contain various colour pigments that can be used as natural sensitisers for DSSCs. Chlorophyll extract from green plants has been used as a sensitiser for DSSC applications in various studies. Recently, the chlorophyll extracts from spinach and pomegranate leaves were demonstrated to have photoelectric conversion efficiencies of 0.131% and 0.597%, respectively.[Citation8,9] Anthocyanins are natural compounds responsible for the red–purple colours of various leaves, flowers and fruits. They have also been used and studied as sensitisers for DSSCs.[Citation10–12]
All these studies use eco-friendly and low-cost natural dyes, but they still use an expensive metal such as platinum (Pt) as a counter electrode due to its high catalytic activity. However, some reports have claimed that platinum gets corroded with triiodide-containing electrolytes and forms platinum iodides such as PtI4.[Citation13,14] Large-scale solar energy conversion systems require abundantly available low-cost materials in the long run and, hence, there is a need to explore a counter electrode that is less expensive and non-corrosive in electrolyte.[Citation15,16] In this study, we have used graphite as a counter electrode in order to achieve this goal.
To date, there have been a very few reports on DSSC applications of natural dyes extracted from the vast diversity of Indian flora. Poinsettia is a common garden plant in India popularly known for its bright red leaf-like bracts. Neem is one of the most widespread and important trees of the Indian subcontinent and is considered to be the ‘nature's drugstore’ in Ayurvedic and Unani medicine. The chlorophyll dye extracted from Neem is very stable and does not degrade for a long time. In this study, chlorophyll extract from Neem leaves and anthocyanin extract from poinsettia bracts have been used as natural sensitisers for eco-friendly DSSCs.
2. Experimental details
2.1. Preparation of natural dye
About 5 g of fresh Neem leaves and poinsettia bracts were separately placed in 50 ml of ethanol and heated up to 70 °C for 15 min to extract their chlorophyll and anthocyanin, respectively. The solutions were filtered using a filter paper (200 nm) to obtain pure natural dyes. The extracts of Neem leaves and poinsettia bracts were mixed in the ratio of 1:1 to obtain a natural dye mixture containing chlorophyll and anthocyanin.
2.2. Synthesis of TiO2 nanoparticles
This work used a previously reported DC arc plasma method to synthesise TiO2 nanoparticles.[Citation17] Commercially available titanium disc was used as anode. The arc was struck in oxygen atmosphere, between graphite cathode and the water-cooled titanium anode; the arc current was maintained at 50 A. The powder formed during the process was allowed to settle on the walls of the chamber and was collected subsequently.
2.3. Preparation of TiO2 photoelectrode and the counter electrode
The synthesised nanoparticles were dispersed in ethanol and deposited using the electrophoretic deposition method on FTO-coated conducting glass substrates at room temperature, forming TiO2 thin films with the average thickness of 12 µm. The TiO2 films were heated in air at 450 °C for one hour to increase film compactness and to vaporise any remaining trace of ethanol in the porous structure of the films. The sintered TiO2 films were immersed in the natural dyes for 24 h at room temperature to allow dye molecules to get adsorbed on TiO2 nanoparticles. Afterwards, the films were gently rinsed in ethanol to remove any unadsorbed dye and other impurities; the photoelectrodes were then ready for encapsulation and testing. The counter electrode was prepared by spraying conductive graphite (Kaiser Fluids) over FTO glass, and the electrode was allowed to dry in air at room temperature for one hour.
2.4. Assembly of dye-sensitised solar cells
DSSCs were assembled in a sandwich-type configuration described in literature [Citation18] as shown in . A DSSC consists of FTO glasses, thin films of dye-adsorbed TiO2 nanoparticles, electrolytes, spacers and counter electrodes. Plastic tape was stuck on all sides of the counter electrode and the photoelectrode was placed on top. The iodide electrolyte solution (0.5 M potassium iodide mixed with 0.05 M iodine in acetonitrile) was injected through the edges and it spread over the active area by capillary action. Finally, two binder clips were used to hold the electrodes together for electrical characterisation.
2.5. Measurements and characterisation
The X-ray diffraction (XRD) pattern was generated using the XRD (Bruker Advance D8) technique. Nickel-filtered Cu Kα radiation (α = 1.54 Å) generated at 40 kV and 40 mA was used for the angle (2θ) range from 20° to 80°. Furthermore, grain size of the nanostructures was confirmed using field emission scanning electron microscopy (FESEM; Zeiss) at 3.70 kV. Molecular bondings were analysed with using Fourier transform infrared spectroscopy (FTIR; Bruker) within the range of 600–4000 cm−1. The absorption spectra of dye solutions and the dyes mixed with TiO2 nanoparticles were recorded using an ultraviolet–visible (UV–vis) spectrophotometer (Ocean Optics). Degradation of the material with respect to temperature was identified by thermogravimetric analysis (TGA) using PerkinElmer STA 6000. The fabricated cells were subjected to the irradiation of a solar simulator (Oriel, USA) with an intensity of 100 mW/cm2 (AM 1.5G) and the current–voltage (I–V) characteristics were measured using Keithley 2420 source measurement unit (SMU). All the measurements were carried out in air at room temperature.
3. Results and discussions
shows the XRD pattern of synthesised TiO2 nanoparticles. The pattern exhibits strong diffraction peaks at 25° and 48°, indicating the anatase phase of TiO2. The peaks are in agreement with the standard spectrum (JCPDS no. 84-1286). The average crystallite size of TiO2 nanoparticles was calculated by Scherrer equation [Citation19] and it was found to be 83 nm. It is well known that in anatase phase of TiO2, electron transfer rate as well as absorption coefficient are higher. These factors help to enhance the photo conversion efficiency of the DSSC.[Citation20] The FESEM image of the sintered TiO2 film on FTO-coated glass substrates is shown in . It can be seen from (a), the morphology of the thin film depicts the compact nature of TiO2 after annealing and better interconnectivity among the nanoparticles. (b) shows the cross section of TiO2 thin film which gives a better idea about the thickness and packing density of TiO2. The mesoporous structure of the electrophoretically deposited film can also be appreciated for dye adsorption. The porosity of the film was measured by image analysis using BioVis 3D software and found to be approximately 15%.
Figure 3. FESEM images of TiO2 nanoparticles deposited on FTO glass by electrophoresis. (a) Morphology of TiO2 nanoparticles. (b) Cross section of TiO2 nanoparticles with FTO.
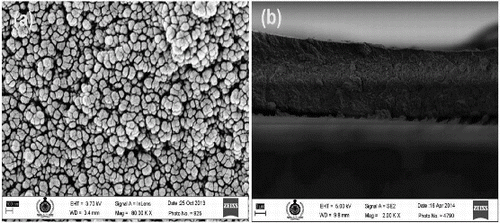
The main pigments of Neem and poinsettia extracts are chlorophyll a and chlorophyll b and anthocyanin, respectively, and their molecular structures are shown in (a) and (b). These structures are well supported by UV–vis spectra reported in literature,[Citation21,22] details of which are discussed in subsequent paragraphs. shows the FTIR spectra of (i) Neem, (ii) poinsettia and (iii) cocktail. Spectrum (i) shows the typical signatures of both chlorophyll a and chlorophyll b. These signatures have been recognised as N–H (def) (1523 cm−1) and N–H (str) (2356 cm−1) in chlorophyll a and chlorophyll b, respectively. Since the extract is ethanol based, free O–H group occurs at 3352 cm−1. Other prominent peaks for Neem extract are C=C (1696 cm−1), C–O (str) (1742 cm−1) and C–H (str) (2859 cm−1). Spectrum (ii) illustrates the anthocyanin extract from poinsettia, where C–H (def) aliphatic and C–H (def) aromatic rings are present at 1419 and 886 cm−1, respectively. Other major groups indicated are C–O (str) (1047 cm−1), C=C (1657 cm−1) and C–H (2960 cm−1). Spectrum (iii) indicates the presence of both chlorophyll a and chlorophyll b of Neem and anthocyanin of poinsettia. However, minor shift in prominent peaks can be observed due to the formation of intermolecular bonding. There is no disappearance of key peaks, which indicates that mixing of dyes has no suppressing effect on each other. Stability of the individual natural dyes was also checked in terms of weight percentage with respect to temperature. indicates that Neem dye can sustain temperature of about 200 °C, whereas poinsettia starts to degrade at 160 °C. Therefore, it is clear that both these dyes can work efficiently under normal sun conditions, where the temperatures are much lower comparatively.
Figure 4. Molecular structures of main pigments of natural dyes. (a) Chlorophyll a and chlorophyll b. (b) Anthocyanin.
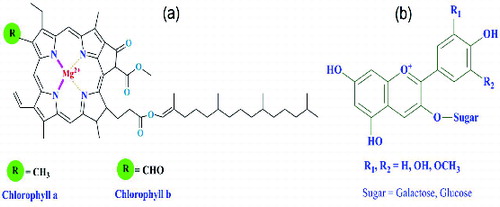
The absorption spectra of Neem extract, poinsettia bract extract and the mixture of Neem and poinsettia bracts (cocktail) are shown in . It can be observed from this figure that chlorophyll of Neem has two broad absorption peaks centred at 471 and 662 nm (green line). Anthocyanin of poinsettia bracts has absorption peaks centred at 383 and 537 nm (red line). Anthocyanin is a class of flavonoid and it contains polyphenol which is responsible for the red colour. This helps enhance the photosynthesis process. The cocktail dye exhibits peaks corresponding to the absorption peaks of the constituent dyes (blue line). It is obvious from this figure that cocktail dye retains the characteristics of both the constituents of natural dyes in the visible region. Hence, it can be inferred that the cocktail dye, which covers almost whole visible region, is a more efficient absorber of solar energy.
shows the comparison of absorption spectra of the films of (a) TiO2 nanoparticles (black line), (b) Neem-adsorbed TiO2 nanoparticles (green line), (c) poinsettia-adsorbed TiO2 nanoparticles (red line) and (d) cocktail-dye-adsorbed TiO2 nanoparticles (blue line). In all the three dye spectra, it is seen that with the dye adsorbed on TiO2, the absorbance of the film in the visible region has significantly improved. The absorption spectrum on TiO2 thin film gets broader at the centre of the peak than its individual dyes, which is very important for good photoconversion efficiency of the solar cell.
Figure 8. (Colour online) UV–visible absorption spectra of pure TiO2 nanoparticles (black line), Neem extract adsorbed TiO2 nanoparticles (green line), poinsettia extract adsorbed TiO2 nanoparticles (red line) and cocktail adsorbed TiO2 nanoparticles (blue).
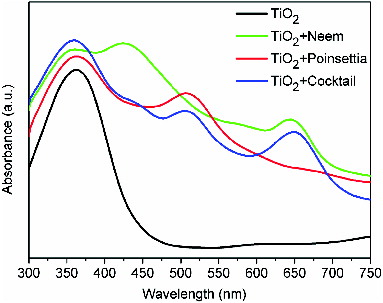
compares the photoelectrochemical properties of DSSCs sensitised by various natural dyes. Neem DSSC has an open circuit voltage (Voc) of 404 mV and a short-circuit current density (Jsc) of 0.43 mA/cm2. The corresponding values for poinsettia DSSC are 406 mV and 0.30 mA/cm2. The Voc for the two DSSCs are nearly the same, but the Jsc is higher in the case of Neem DSSC, which indicates the better charge transfer to TiO2 nanoparticles. The fill factor of poinsettia DSSC is 45.8%, which is better than that of Neem DSSC (40.1%). However, the photoelectric conversion efficiency of Neem solar cell is 0.72%, which is better than that of the poinsettia-based solar cells (0.56%).
Table 1. Photoelectrochemical parameters of the fabricated DSSCs.
The cocktail-dye-based DSSC exhibits Jsc of 0.52 mA/cm2 and power conversion efficiency of approximately 1%. The fill factor of the cell sensitised by the cocktail dye is 46.7%, which actually helps improve the overall efficiency of DSSC. Thus, the performance of the cocktail-dye-based DSSC is superior to that of the other two cells. This is because the absorption range of wavelength for the cocktail dye is broader than that of the individual dyes, and the charge generation and its transportation to TiO2 nanoparticles also get improved as an evidence of higher Jsc and fill factor (FF). The Jsc of cocktail-dye-based DSSC is 70% higher than that of poinsettia DSSC and almost 25% higher than that of Neem DSSC. This demonstrates that the principle of harvesting more number of photons by making a cocktail dye works very effectively in this case. shows the current density–voltage (J–V) curves for the three DSSCs based on Neem extract, poinsettia extract and the cocktail of these two extracts. The DSSC based on cocktail dye shows the highest efficiency and it is approximately 1%.
shows the comparison of performance characteristics of DSSCs based on natural dyes which have been reported earlier in the literature [Citation21,Citation23,Citation24] with our DSSCs. It is clear that the major difference is Jsc which is much higher in the case of cells with platinum as the counter electrode, whereas the fill factor and the open circuit voltage are comparable. Platinum is a better catalyst than graphite, and since it is a metal, its sheet resistance is much lower. These factors are responsible for higher current densities exhibited by DSSCs with platinum as the counter electrode. However, as we wanted to use a counter electrode that does not undergo corrosion, we were able to achieve this goal with graphite as a cost-effective counter electrode.
Table 2. Comparison of photoelectrochemical properties of dye-sensitised solar cells based on natural extracts.
4. Conclusions
TiO2 nanoparticles synthesised by DC arc plasma method were utilised for fabrication of a photoelectrode of DSSC. The DSSCs based on natural dyes extracted from Neem leaves and poinsettia bracts as well as a mixture of these dyes were fabricated and tested under AM 1.5 illumination. The highest efficiency of cocktail-dye-based DSSC is 1%, and its efficiency is comparable with published results based on other natural dyes. It also displayed the highest fill factor and short-circuit current density. Finally, we have fabricated environment-friendly DSSCs based on nanostructure of TiO2, natural dyes as sensitisers and conducting graphite while exploring for a cheaper and durable alternative to the counter electrode.
Acknowledgements
The authors would like to thank the Vice Chancellor of DIAT, Pune, for extending all the facilities of the institute. Tejashree Bhave and Sanjay Sahare acknowledge financial support from DRDO-DIAT Programme on Nanomaterials from ER-IPR of Defence Research and Development Organization.
References
- O’Regan B, Gratzel M. A low-cost, high-efficiency solar cell based on dye-sensitized colloidal TiO2 films. Nature. 1991;353:737–740.
- Nazeeruddin MK, De Angelis F, Fantacci S, Selloni A, Viscardi G, Liska P, Ito S, Takeru B, Gratzel M. Combined experimental and FT-TDDFT computational study of photoelectrochemical cell ruthenium sensitizers. J Am Chem Soc. 2005;127:16835–16847.
- Gao F, Wang Y, Shi D, Zhang J, Wang M, Jing X, Humphry-Baker R, Wang P, Zakeeruddin MK, Gratzel M. Enhance the optical absorptivity of nanocrystalline TiO2 film with high molar extinction coefficient ruthenium sensitizers for high performance dye-sensitized solar cells. J Am Chem Soc. 2008;130:10720–10728.
- Park NG, Kim K. Transparent solar cells based on dye-sensitized nanocrystalline semiconductors. Phys Status Solidi A. 2008;205:1895–1904.
- Choi H, Lee JK, Song K, Kang SO, Ko J. Novel organic dyes containing bis-dimethylfluorenyl amino benzo[b]thiophene for highly efficient dye-sensitized solar cell. Tetrahedron. 2007;63:3115–3121.
- Chen R, Yang X, Tian H, Wang X, Hagfeldt A, Sun L. Effect of tetrahydroquinoline dyes structure on the performance of organic dye-sensitized solar cells. Chem Mater. 2007;19:4007–4015.
- Hou W, Pavaskar P, Liu Z, Theiss J, Aykol M, Cronin SB. Plasmon resonant enhancement of dye sensitized solar cells. Energy Environ Sci. 2011;4:4650–4655.
- Chang H, Wu HM, Chen TL, Huang KD, Jwo CS, Lo YJ. Dye-sensitized solar cell using natural dyes extracted from spinach and ipomoea. J Alloys Compounds. 2010;495:606–610.
- Chang H, Lo YJ. Pomegranate leaves and Mulberry fruit as natural sensitizers for dye-sensitized solar cells. Sol Energy. 2010;84:1833–1837.
- Zhu H, Zeng H, Subramanian V, Masarapu C, Hung K, Wei B. Anthocyanin-sensitized solar cells using carbon nanotube films as counter electrodes. Nanotechnology. 2008;19:465204–465208.
- Polo AS, Murakami TN, Iha NY. Blue sensitizers for solar cells: natural dyes from Calafate and Jaboticaba. Sol Energy Mater Sol Cells. 2006;90:1936–1944.
- Patrocinio AOT, Mizoguchi SK, Paterno LG, Garcia CG, Iha NY. Efficient and low cost devices for solar energy conversion: efficiency and stability of some natural-dye-sensitized solar cells. Synth Met. 2009;159:2342–2344.
- Olsen E, Hagen G, Lindquist SE. Dissolution of platinum in methoxy propionitrile containing LiI/I2. Sol Energy Mater Sol Cells 2000;63:267–273.
- Veerappan G, Bojan K, Rhee SW. Sub-micrometer-sized graphite as a conducting and catalytic counter electrode for dye-sensitized solar cells. ACS Appl Mater Interfaces. 2011;3:857–862.
- Huang Z, Liu X, Li K, Li D, Luo Y, Li H, Song W, Chen L, Meng Q. Application of carbon material as counter electrodes of dye sensitized solar cell. Electrochem Commun. 2007;9:596–598.
- Roy-Mayhew JD, Bozym DJ, Punckt C, Aksay IA. Functionalized graphene as a catalytic counter electrode in dye-sensitized solar cells. ACS Nano. 2010;4:6203–6211.
- Banerjee I, Karmakar S, Kulkarni NV, Nawale AB, Mathe VL, Das VK, Bhoraskar SV. Effect of ambient pressure on the crystalline phase of nano TiO2 particles synthesized by a dc thermal plasma reactor. J Nanopart Res. 2009;12:581–590.
- Smestad GP. Education and solar conversion: demonstrating electron transfer. Sol Energy Mater Sol Cells. 1998;55:157–178.
- Patterson AL. The Scherrer formula for X-Ray particle size determination. Phys Rev. 1939;56:978–982.
- Park NG, Lagemaat J, Frank AJ. Comparison of dye-sensitized rutile and anatase based TiO2 solar cells. J Phys Chem. B. 2000;104:8989–8994.
- Wongcharee K, Meeyoo V, Chavadej S. Dye-sensitized solar cell using natural dyes extracted from rosella and blue pea flowers. Sol Energy Mater Sol Cells. 2007;91:566–571.
- Luiza A, Nery P, Liegel RM, Fernandez C. Fluorescence and chemiluminescence: teaching basic principles by simple demonstration experiments. Chem Educ J. 2009;13:13–22.
- Calogero G, Marco GD. Red Sicilian orange and purple eggplant fruits as natural sensitizers for dye-sensitized solar cells. Sol Energy Mater Sol Cells. 2008;92:1341–1346.
- Hao S, Wu J, Huang Y, Lin J. Natural dyes as photosensitizers for dye-sensitized solar cell. Sol Energy. 2006;80:209–214.