Abstract
Commercial nanofiltration polyethylene terephthalate (PET) thin-film composite membranes were treated by low-pressure gamma-ray plasma, and the effects of the plasma treatment were investigated in terms of the membrane hydrophilicity, thickness and deposition rate. Experimental results indicated that the membrane surface hydrophilicity was increased by the plasma treatment and changes in the hydrophilicity, as well as membrane performance including yield strength varied with the original membrane characteristics (e.g., roughness and hydrophilicity).
Keywords:
1. Introduction
Commercial interest in electrochromic devices (ECDs) has centred around three main types of product: displays, mirrors and windows.[Citation1] Several companies and major glass manufacturers have built laminated or monolithic demonstration devices on glass. Electrochromic devices on flexible substrates have been investigated for the specific advantage of having versatile shapability. Since the discovery of the electrochromic phenomenon in tungsten oxide films,[Citation2] this material has remained the most promising candidate for large-scale uses of electrochromic devices.
PET/metal film has been widely used in the electronic packaging field for application such as flexible printed circuits, printed circuit boards and cable assembles. For such applications, PET film has been well known as one of the attractive materials because of their excellent thermal stability, good mechanical properties, low dielectric constant and good chemical resistance.[Citation3] To meet the recent demands for miniaturisation of the electronic circuits, the direct metallisation on the PET film without any adhesive is required. However, there are still critical issues to be resolved for their use. One of the technical concerns for the PET/metal system is the improvement of the interfacial adhesion. The surface modification of polymer is a main technique to achieve good adhesion by forming the new chemical species that can create bonds to overlay metal films.[Citation4]
In the past 10 years, a variety of inorganic nanofillers have been considered as the reinforcements to improve the creep resistance of polymer matrix because they have high surface-to-volume ratio and the possibility of forming network structure by dispersing nanofillers dispersed into polymer matrix to restrict the mobility of polymer chain.[Citation5] Low-pressure plasma treatment is well known for its ability to generate reactive radicals that can lead to polymer grafting and introduction of chemical functional groups onto membrane surfaces.[Citation6] The advantage of plasma treatment is its ability to tailor the surface property without affecting the bulk structure of membrane for enhanced membrane performance.[Citation7] Many studies have attempted to modify the membrane by plasma to improve its antifouling characteristics. Variables evaluated include plasma sources, treatment methodologies and types of membrane originally used. The use of oxygen, nitrogen, ammonia, argon and helium, or mixture gases as plasma source, was common to create antifouling membranes.[Citation8–10]
In this research, PET was modified by gamma ray. This approach combined mechanical activation of the substrates and their intensive mixing with a reactant under the action of pressure and shear strains which allows one to obtain new polymer materials. The technique has numerous advantages, since the entire modification proceeds in a solid state and does not require any component melting or an employment of any solvents as a reaction medium.
2. Experimental
2.1. Materials and composite preparation
PET film with a thickness of 25 mm was provided by Shanghai Bang Kai Co. All membranes were rinsed with ethyl alcohol (95%, Fisher Scientific, China) and de-ionised water, then dried in oven (50 °C) for 24 h. Low-pressure plasma treatment in a bell-jar reactor system was used here. The plasma system was evacuated below 3 m Torr in every start to remove the residue moisture. At vacuum, plasma source gas (NH3, anhydrous 99.99%, Scott Specialty Gases, Pennsylvania, PA) was introduced to 50 m Torr. Radiofrequency (13.56 MHz) power in a range of 10–90 W and predetermined treatment time (from 1 to 10 min) was applied for treatment.
The PET films were cut into 3 × 3 cm sections for TiO2 coating. Before the coating, the films were washed ultrasonically in acetone and distilled water for 30 min and then dried at room temperature. Thin TiO2 layers were deposited on PET substrates by dip coating method in a titanium isopropoxide sol. The titania solution consisted of Ti (O-i-C3H7)4 (Alfa Aesar, India), H2O, C2H5OH and HNO3 (SD Fine Chemie Limited, India) in a 1.0:1.0:9.25:0.1 in molar ratio. Precursor solutions for TiO2 layers were prepared by the following method. Titanium isopropoxide dissolved in ethanol was vigorously stirred for 2 h at 0 °C of temperature and then a mixture of water and HNO3 was added dropwise to the above solution with a burette under stirring. The resultant alkoxide solution was kept standing at 45 °C temperature for hydrolysis reaction for 36 h, resulting in the TiO2 sol. PET films were used as the substrate for thin-film deposition. Before TiO2 coating, the PET samples were subjected to air plasma for 10 min, and the working pressure and the discharge potential was 0.2 mbar and 400 V, respectively.
2.2. Determination of contact angles
The contact angles of the membrane support layers were measured using the sessile drop method on a CAM 101 series contact angle goniometer (KSV Company, Linthicum Heights, MD). The values were taken as an average of at least four points with a volume of 10 ± 1 mL.
3. Results and discussion
3.1. Contact angel
shows the contact angle of the polymer substrates with different plasma pretreatment time. In PET substrate, the contact angle decreased from 62.2° to 5.2° after the plasma pretreatment. The lower contact angle indicates the higher surface energy and it also indicates the increase of adhesive force at the polymer surface by forming functional group on the surface and also the removal of organic surface contaminants. The contact angle decreases significantly as the plasma treatment time increases up to 60 s and the angle slightly decreases as the plasma treatment time increases from 60 to 600 s.
shows the substrate temperature variation with the PET film thickness. It was found that the substrate temperature during deposition increase linearly as the thickness, although the substrate was not intentionally heated during the deposition, because the film thickness hinders the evaporating of heat according to the rate of heat exchange. The substrate temperature during deposition increased, respectively, from 46 to 54, 62, 68 and 86 °C, when the film thickness increased from 72 to 158, 204, 305 and 447 nm.
3.2. Thermal properties
The thermal stability of both crystallised pure PET and TiO2/PET film samples containing 2 wt% nano-TiO2 load at different heating rates is investigated by thermo gravimetric analysis (TGA), with their patterns shown in , where (a) and (b) represent the thermal degradation curves for PET and TiO2/PET samples. It is seen that with the increase in heating rate, the values of Tonset, Td and Tend for both PET and TiO2/PET samples increase. At the same heating rate, however, Tonset, Td and Tend of TiO2/PET are all higher than those of pure PET, showing that the thermal stability of TiO2/PET is superior to that of PET. Nano-TiO2 can prevent PET from degradation by creating many polarised fibers, which makes the stability of TiO2/PET enhance.
3.3. Effect of surface modification on membrane surface chemical structure
The Fourier transform infrared (FTIR) spectra of PET samples in absorption mode are shown in . On comparing the FTIR spectra of PET film to metallised PET films, several new peaks that indicated chemical interaction between PET and Ti have been observed. The absorption peak at 420 cm−1 is attributed to the vibration of Ti cations. A sharp and low-intensity band at 640 cm−1 in FTIR spectra of metallised PET films is attributed to Ti–O stretching. A sharp band around 960 cm−1 could be assigned to longitudinal stretching vibrations of Ti–H. This metal bonding confirms that the metal ions have been attached to the polymer chain of PET, hence altering its mechanical behaviour.
It is clearly seen from the figure that both the characteristic absorption peaks of monomer PET are presented in the spectrum of copolymer. The peaks at 2972 and 2879 cm−1 are attributed to the C–H stretching vibrations of methyl and ethyl, and the asymmetry and symmetry bending vibrations of –CH3 appear at 1456 and 1363 cm−1, respectively. The bands of amide I at 1641 cm−1 and amide II at 1543 cm−1 are considered to be from the C–O stretching and the N–H in-plane bending, respectively, while the peak at 1265 cm−1 corresponds to the C–N stretching vibration of amide. Additionally, the carbonyl stretching bond attributed to the carboxylic acid group of AAc units is observed at 1713 cm−1in the copolymer.
The attenuated total reflection (ATR)-FTIR was employed to investigate the change of surface chemical structure of the composite membranes before and after modification.
The ATR-FTIR spectra of a representative modified membrane, which was coated with TiO2 layer, and a virgin PET membrane are presented in . It can be clearly seen that, after coating with TiO2 surface layer, the peaks at 1606, 1581,1487 and 1449 cm−1 as shown in the spectra of the virgin membrane, which are assigned to the vibration of benzene, disappear in the spectra of the modified membrane. The band of amide I at 1657 cm−1 of the virgin membrane shifts to a lower frequency of about 1648 cm−1 after modification. The possible reason is that when the C–O groups form hydrogen bonds with more N–H groups on the surface of the modified membrane, the hydrogen bonding tends to decrease the double-bond character of the C–O moiety, shifting the adsorption band to lower frequency. Furthermore, a new peak at 1710 cm−1, which is assigned to C–O stretching bond of the carboxylic acid group, appears after modification. All the results of ATR-FTIR analysis suggest that TiO2 has been successfully coated on the surface of thin-film composite (TFC) membrane even after being rinsed with de-ionised water of room temperature.
The variation of the values of yield strength with temperature for PET and TiO2/PET is shown in . It has been observed from this figure that yield strength of these samples decreases almost linearly with the increasing temperature up to a certain temperature beyond which it shows a slightly increasing trend. Yield strength decreases with the increasing temperature. This behaviour indicates that as temperature increases, the movement of the chains becomes easier and, consequently, the resistance to deformation is smaller. Hence the metallised PET films showed a higher resistance to deformation than that of commercial PET film.
shows the surface structure of PET materials from the SEM images. As shown in , the PET substrate is smoother than the coatings. Many surface protrusions are observed on the surfaces of these coatings. A rough surface could provide more capacity for the coating to capture inks and also facilitate the penetration of inks into the coating. With the help of SEM, the dispersion of nano-TiO2 in coatings was examined as shown in . Some open structures with some pores and severe aggregated TiO2 particles were observed in (a), which can decrease the void volume. When the ink hits the surface, the surface structures presented in (a) easily prevented normal diffusion. As shown in (b), there are many more open smaller pores, fine, closely modified structures with a large surface area, and large pigments are equably distributed with subtle aggregation. The results indicate that the dispersibility of TiO2 particles is improved well.
The carrier concentration and Hall mobility values are shown in . The results indicated that the Hall mobility was decreased when the thickness grew, and the carrier concentration was raised. Hall mobility decreased with the increase in grain size due to the electron scattering at the grain boundaries. As the film thickness increases, the crystal grain size becomes bigger, which will lead to an obvious decrease of the density of the grain boundary and the carrier mobility. As the substrate temperature increases, the sputtered atoms or particles on the growing surface will gain more kinetic energy, which will also be beneficial to the film crystallisation, and will decrease the voids and defect creation.
Figure 8. The variations of the carrier concentration and the carrier mobility of the ITO films with different thickness.
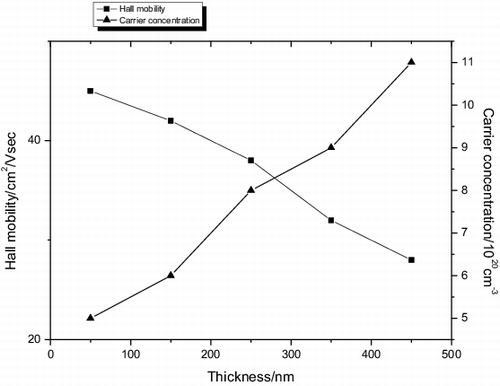
It had been reported that the carrier mobility in thin films was limited by the ionised impurity scattering, the neutral impurity scattering, and the grain boundary scattering. The carrier concentration and mobility are also closely related with the structure of films. Several researchers have proposed that the main conduction mechanism of films deposited at low-substrate temperature is due to the creation of oxygen vacancies, which donate two electrons for each vacancy. When the structure of the films is amorphous, some electrons are bound in short range by the net non-uniform structure. While the structure of the films becomes crystalline, these electrons will be released from the bound. Near the amorphous–crystalline transition point, we think that the lattice transition was the major influence on the carrier mobility.
4. Conclusions
TiO2 has been successfully coated on the surface of TFC membrane even after being rinsed with de-ionised water of room temperature. The thermal properties and contact angle measurements revealed that the surface layer of the film casted after dissolution of the blend in organic solvent was enriched with the TiO2 component. The plasma treatment of the composite films as well as the films prepared from initial components led to the surface hydrophilicity increase. The metallised PET films showed higher resistance to deformation than that of commercial PET film. Nano-TiO2 can prevent PET from degradation by creating many polarised fibers, which makes the stability of TiO2/PET enhance.
References
- Kim E-S, Yu Q, Deng B. Plasma surface modification of nanofiltration (NF) thin-film composite (TFC) membranes to improve anti organic fouling. Appl Surf Sci. 2011;257(23):9863–9871.
- Xu J, Feng X, Gao C. Surface modification of thin-film-composite polyamide membranes for improved reverse osmosis performance. J Memb Sci. 2011;370(1–2):116–123.
- Popov C, Kulisch W, Bliznakov S, Ceccone G, Gilliland D, Sirghi L, Rossi F. Surface modification of nanocrystalline diamond/amorphous carbon composite films. Diam Relat Mater. 2008;17(7–10):1229–1234.
- Chau JLH, Yang C-C. Surface modification of silica nanopowders in microwave plasma. J Exp Nanosci. 2014;9(4):357–361.
- Mansourpanah Y, Momeni Habili E. Preparation and modification of thin film PA membranes with improved antifouling property using acrylic acid and UV irradiation. J Memb Sci. 2013;430:158–166.
- Mu S, Wu Z, Wang Y, Qi S, Yang X, Wu D. Formation and characterization of cobalt oxide layers on polyimide films via surface modification and ion-exchange technique. Thin Solid Films. 2010;518(15):4175–4182.
- Daoush WM, Lim BK, Nam DH, Hong SH. Microstructure and mechanical properties of CNT/Ag nanocomposites fabricated by spark plasma sintering. J Exper Nanosci. 2014;9(6):588–596.
- Demina T, Zaytseva-Zotova D, Yablokov M, Gilman A, Akopova T, Markvicheva E, Zelenetskii A. DC discharge plasma modification of chitosan/gelatin/PLLA films: surface properties, chemical structure and cell affinity. Surf Coat Technol. 2012;207:508–516.
- Yamamoto G, Yamashita T, Matsuo K, Hyodo T, Shimizu Y. Effects of polytetrafluoroethylene or polyimide coating on H2 sensing properties of anodized TiO2 films equipped with Pd–Pt electrodes. Sens Actuator B. 2013;183:253–264.
- Zhan J, Tian G, Qi Wu, S Z, Wu D, Jin R. Fabrication and mechanism study of CuO layers on double surfaces of polyimide substrate using surface modification. Comp Sci Technol. 2012;72(9):1020–1026.