Abstract
Chitosan/polyrhodanine nanocomposite has been synthesised by in situ chemical oxidation polymerisation of rhodanine in chitosan aqueous solution and characterised by scanning electron microscopy, transmission electron microscopy (TEM), and Fourier transform infrared spectroscopy (FTIR). The FTIR measurement exhibited the existence of relevant functional groups of both polyrhodanine and chitosan in the nanocomposite. The result of TEM image showed the dispersion of polyrhodanine in chitosan matrix performed successfully and the diameter of polyrhodanine nanoparticle estimated smaller than 30 nm. This nanocomposite was used for Ni(II) removal from aqueous solution. Batch adsorption studies were carried out with various Ni(II) concentrations, amount of adsorbent, pH, contact time, and temperature. The pH 8.0 was found to be an optimum pH for Ni(II) adsorption. The Langmuir, Freundlich, and Sips adsorption isotherm model was applied to describe the isotherms. This adsorbent gave the maximum equilibrium uptakes of Ni(II) of and
according to the Langmuir and Sips isotherm models, respectively. The thermodynamic data showed that adsorption processes were thermodynamically favourable, and exothermic in nature. Kinetics studies indicated that the removal of Ni(II) ions followed pseudo-second-order kinetics, suggesting that the specific interaction with surface functional groups was the major route of the removal process.
1. Introduction
The removal of toxic heavy metals from aqueous streams is an important issue facing industries discharging effluents bearing heavy metals.[Citation1] Wastewater discharge from electroplating, electronics, and metal cleaning industries often contains high concentrations of nickel ions and causes serious water pollution. The presence of nickel ions above critical levels may cause various types of acute and chronic disorder in human health.[Citation2,Citation3] There are many conventional methods that are being used to remove metal ions, including oxidation, reduction, precipitation, membrane filtration, ion exchange, and adsorption.[Citation4] Adsorption is a relatively effective and economical method compared with other methods, which occasionally suffer from high operation cost and low treatment efficiency.[Citation5]
Numerous efforts have been contributed to the development of effective adsorbents like activated carbon, chitosan, zeolite, polymer, functionalised silica, and clay.[Citation6] Among the adsorbents for removal of heavy metal, natural polymers are considered as outstanding candidates since they are non-toxic, reproducible, inexhaustible, biodegradable, and environment friendly. In particular, chitosan is one of the high-performance natural polysaccharide materials.[Citation7] The presence of a large number of functional groups, such as amino group (NH2) and hydroxyl group (OH), that acts as active sites on the surface of chitosan give it a high adsorption capacity for metal ions.[Citation8] Most natural polymers except chitosan have negative charges in solutions. However, chitosan is a polymer with positive charges in solutions which have attracted interest in wastewater treatment.[Citation9] The use of powder or flaked forms of chitosan in adsorption process is well known. There are disadvantages to these forms, such as their solubilisation in acid media and a decrease in the rate of the adsorption process.[Citation10] In the past few years, a considerable number of studies dealing with the synthesis of nanocomposites of chitosan with various synthetic and natural polymers, such as polyvinyl alcohol,[Citation11] polyethylene oxide,[Citation12] polyaniline,[Citation13] polypyrrol,[Citation14] polyacrylic acid,[Citation15] cellulose and its derivatives [Citation16] have been published.
In this investigation, a nanocomposite of chitosan and polyrhodanine was synthesised. The rhodanine derivatives have been applied for antiviral, antibacterial, antihistaminic, and anticorrosion agents.[Citation17] In addition, according to the hard and soft acids and bases theory introduced by Pearson, polyrhodanine can be expected as a promising candidate for efficient adsorbtion of heavy metal ions because it contains oxygen, nitrogen, and sulphur atoms in its monomeric structure.[Citation18] Both polyrhodanine and chitosan can adsorb heavy metals from aqueous environments. Hence, attempts have been made to investigate the metal adsorbing capacity of chitosan/polyrhodanine (CS/PRh) nanocomposite for heavy metal nickel removal from metal solutions.
2. Experimental section
2.1. Material
In this work, chitosan, rhodanine (C3H3NOS2), acetic ccid (CH3COOH), and iron (III) chloride (FeCl3) were used. Chitosan, of which the degree of deacetylation is 75%–85% and medium molecular weight was obtained from Orbital Company, India. All of the other reagents were supplied by Merk Company, Germany. All reagents were used without further purification. Distilled water was used throughout the experiment.
2.2. Characterisation
The morphology and size of the resulting product were characterised using scanning electron microscopy (SEM; KYKY-EM3200-china) and transmission electron microscopy (TEM; Zeiss-EM10C-Germany). The molecular structures of the synthesised CS/PRh nanocomposite were investigated using the Fourier transform infrared (FTIR) spectroscopy (Bruker, karlsrohe, Germany).
2.3. Synthesis of CS/PRh nanocomposite by in situ polymerisation
The CS/PRh nanocomposite was obtained by polymerisation of rhodanine in chitosan solution. Chitosan solution 1 (w/v) was prepared in 50 mL aqueous solution of acetic acid 1% under magnetic stirring. Rhodanine (0.15 gr) was dissolved in 50 mL water at 60 °C –80 °C and dropped in the clear chitosan solution. Then solution of iron (III) chloride in 50 mL of aqueous solution as oxidant was dropped into the above solution with continued stirring. The polymerisation was carried out for 20 h at room temperature. After that, the solution was centrifuged, then washed with deionised water, and dried at 45 °C for 24 h.
3. Result and discussion
3.1. FTIR characterisation
FTIR analyses were conducted to consider the chemical structure of substances. shows the FTIR spectrums of pure chitosan and CS/PRh nanocomposite. In the chitosan spectrum ( (a)), there was a broad characteristic peak at around 3445 cm−1 corresponding to the stretching vibration of O–H and N–H bonds. A peak at 1651 cm−1 is attributed to N–H bending in NH2.[Citation19] The peaks at 2854 and 1384 cm−1 were both attributed to the C–H stretching vibration of alkyl group.[Citation20]
shows the spectra for CS/PRh nanocomposite which was obtained with the oxidation polymerisation of rhodanine in chitosan solution. In addition to the stretching vibration of chitosan, the peaks attributed to polyrhodanine were observed in FTIR spectra of this nanocomposite. The peak at 1180 cm−1 was assigned to the C–O− stretching vibration. A band at 1404 cm−1 is assigned to the C=N+ stretching in the polymer chain. The peak at around 1623 cm−1 was attributed to the stretching vibration of C=C groups in the polymer chain.[Citation21] The N–H stretching peak at around 3450 cm−1 increased compared with the peak of the chitosan. The FTIR spectra of nanocomposite reveal that all of the characteristic peaks of both chitosan and polyrhodanine exist in the synthesised nanocomposite structure. Based on the FTIR spectra, the proposed structure of nanocomposite is shown in .
3.2. SEM and TEM characterisations
The morphologies of CS/PRh nanocomposite were investigated by SEM () and TEM (). The TEM observation reveals the formation of the nanocomposite and the dispersion of the polyrhodanine nanoparticles in the chitosan matrix. We can deduce that chitosan is a good stabiliser for dispersion of nanoparticles. From TEM image, the average size of the nanoparticles is approximately smaller than 30 nm.
3.3. Adsorption experiments
The sorption experiment was carried out by the batch method in water. The samples of 0.1 g of CS/PRh nanocomposite were equilibrated with 10 mL of solutions of Ni (II) in the vials. The contents were shaken thoroughly using a thermostated shaker rotating at a speed of 400 rpm. The solution was then filtered and the residual nickel concentrations were measured using the atomic absorption spectrometer. Thermodynamic parameters of the adsorption were established by conducting the experiments at 303 K, 313 K and 323 K in a temperature-controlled mechanical shaker. The sorption capacity of the sorbents was studied at different conditions, such as the contact time of the sorbent for maximum sorption and pH of the medium.
The adsorbed amount of Ni(II) per unit weight of CS/PRh nanocomposite, , was calculated from the mass balance equation as
(1) where
and
are the initial Ni(II) concentration and the Ni(II) concentration at any time t, respectively; V (L) is the volume of the Ni(II) solution; and m (g) is the mass of the CS/PRh nanocomposite. Samples of the Ni(II) solution were collected at predetermined time intervals and analysed using an atomic spectrophotometer.
3.4. Effect of pH on the adsorption process
The pH value of the solution is one of the most important environmental factor influencing not only site dissociation, but also the solution chemistry of the heavy metals. Therefore, selecting an optimum pH is very important for the adsorption process. The pH was investigated over the range from 2 to 8. The pH value of solutions was adjusted by using diluted solutions of NaOH and HCl. As indicated in , the maximum capacity of Ni(II) absorption occurred at pH of 8. Nickel is presented essentially as hydrated Ni2+ in lower pH solution and as a monovalent cation Ni(OH)+, even as Ni(OH)2 with the increase of pH. The distribution of these species is function of pH. At pH value higher than 8, the adsorption studies could not be carried out because of the precipitation of Ni(OH)2. Increase in metal removal with increased pH can be explained on the basis of the decrease in competition between proton and metal cations for same functional groups and by decrease in positive surface charge, which results in a lower electrostatic repulsion between surface and metal ions.[Citation22]
3.5. Effect of contact time
Ni(II) removal by adsorbent as a function of contact time with initial concentration of Ni(II) is shown in where the adsorption rate of metal uptake was quite slow. Results indicated that metal ions removal was increased with an increase in contact time before equilibrium was reached. This means that a large amount of vacant adsorption sites were available at this stage. Further increase in contact time does not increase the uptake due to unavailability of adsorption sites of the adsorbent materials.
Rate of adsorption is of great significance for developing the adsorbent-based water technology.[Citation23] Thus, the ability of developed beads to adsorb maximum amount of Ni(II) within 3 h indicates their suitability as an effective adsorbent.
3.6. Effect of adsorbent dose
The dependence of Ni(II) adsorption was studied by varying the amount of adsorbents from 0.05 to 0.2 g, while keeping other parameters (pH, temperature, and contact time) constant. From , it can be observed that removal efficiency of the adsorbent generally improved by increasing its dosage. This is expected due to the fact that the higher dose of adsorbents in the solution, the greater availability of exchangeable sites for the ions.
3.7. Adsorption isotherms
In general, an adsorption isotherm is an invaluable curve describing the phenomenon governing the retention (or release) or mobility of a substance from the aqueous porous media or aquatic environments to a solid-phase at a constant temperature and pH.[Citation24] To quantify the sorption capacity of the sorbent studied for the nickel removal, the two most commonly used isotherms, namely the Langmuir and Freundlich from two parameter isotherms and the Sips from three parameter isotherms, have been adopted.
3.7.1. Two-parameter isotherms
3.7.1.1. Langmuir isotherm. The Langmuir isotherm assumes a surface with homogeneous binding sites, equivalent sorption energies, and no interaction between sorbed species. In mathematical form, it is written as
(2) where
is the maximum sorption capacity (corresponding to complete monolayer coverage), Ce is the equilibrium concentration in the solution
,
is the equilibrium
concentration in the sorbent
, and KL is the sorption affinity constant related to the binding energy of sorption
In addition, a dimensionless constant, separation factor or equilibrium parameter, , was calculated to characterise the isotherms
(3) where
is the initial
concentrations. The
values indicate whether the adsorption is unfavourable
, linear
, favourable
, or irreversible
[Citation25] For all the tested
concentrations,
values are in the range of
, which indicates that the adsorption of
on CS/PRh is a favourable process.
3.7.1.2. Freundlich isotherm. The Freundlich isotherm equation is used for the description of multilayer adsorption with the interaction between adsorbed molecules. The model applies to the adsorption onto heterogeneous surfaces with uniform energy distribution and reversible adsorption. The non-linear form of Freundlich equation may be written as
(4) where
is the empirical Freundlich constant or capacity factor
and 1/n is the Freundlich exponent.
The Freundlich isotherm parameter 1/n measures the adsorption intensity of metal ions on sorbent. The value of 1/n, less than unity is an indication that significant adsorption takes place at low concentration but the increase in the amount adsorbed with concentration becomes less significant at higher concentration and vice versa.
3.7.2. Three-parameter model
3.7.2.1. Sips model. Recognising the problem of the continuing increase in the adsorbed amount with an increase in concentration in the Freundlich equation, Sips proposed an equation similar in form to the Freundlich equation, but it has a finite limit when the concentration is sufficiently high
(5) where
is the Sips maximum adsorption capacity
,
the Sips equilibrium constant
, and
is the Sips model exponent.
The isotherms obtained using the non-linear method for the adsorption of Ni(II) on CS/PRh nanocomposite was shown in .
Figure 8. Two- and three-parameters isotherms obtained using the non-linear method for the adsorption of Ni(II) on CS/PRh nanocomposite.
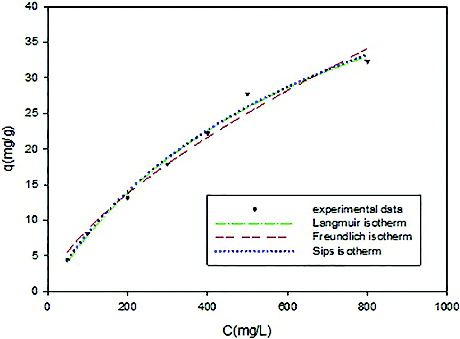
The value of R2 can give more information about the suitability of adsorption model. The high values of the correlation coefficient (R2 > 0.99) showed that the Langmuir and Sips isotherm equations can satisfactorily describe the adsorption of Ni(II) onto CS/PRh nanocomposite. The parameters of isotherms are reported in .
Table 1. Langmuir, Freundlich and Sips constants and correlation coefficients (R2) for the Ni(II) adsorption on CS/PRh nanocomposite.
3.8. Thermodynamic studies
In this section, thermodynamic aspects of the adsorption process will be considered. The experimental data obtained at different temperatures were used in calculating the thermodynamic parameters such as Gibbs free energy (ΔG), enthalpy (ΔH) and entropy (ΔS) according to the following equations
(6)
(7) where
, known as the distribution coefficient of the adsorbate, is equal to
and R is the universal gas constant. A plot of ln
as a function of 1/T yields a straight line. The ΔH and ΔS parameters were calculated from the slope and intercept of the plot, respectively.
The negative value of ΔG obtained from EquationEquation (6)(6) reflects a spontaneous (favourable) adsorption process of
, while the negative value of ΔH indicates that the adsorption reaction is exothermic and the adsorption of
is more effective at lower temperatures ().
Table 2. Thermodynamic data of Ni(II) adsorption process on CS/PRh nanocomposite.
3.9. Kinetic studies
Kinetics and equilibrium are the two major parameters for evaluating adsorption dynamics. In order to investigate the controlling mechanism of the adsorption, the pseudo-first-order and pseudo-second-order kinetics models were used to test the experimental data of the adsorption of Ni(II) ions by CS/PRh nanocomposite. The pseudo-first-order rate expression of Lagergren is given as , where
and
are the amounts of Ni(II)
adsorbed on the adsorbent at equilibrium and at time t, respectively and
is the rate constant of first-order adsorption
. The slope and intercept of plot of
vs. t were used to determine the first-order rate constant,
. The pseudo-second-order kinetic model is expressed as:
where
is the rate constant of second order adsorption. The slopes and intercepts of plots of
vs. t were used to calculate the second-order rate constant
and
().
Figure 9. Kinetic pseudo-second-order sorption kinetics of Ni(II) adsorption on CS/PRh nanocomposite.
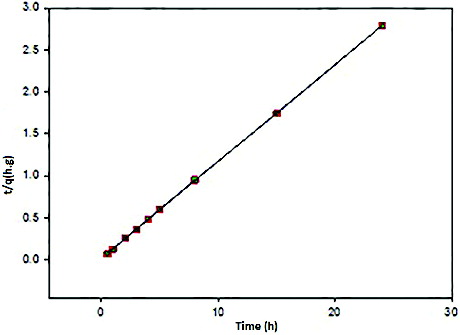
Better correlation coefficients were obtained with the pseudo-second-order kinetic model (0.999) compared with the pseudo-first-order kinetic model (0.343). Therefore the chemisorptions were the rate controlling mechanism. They were fully consistent with those drawn from adsorption isotherm analysis as mentioned above. The kinetics parameters obtained by fitting the kinetics data of Ni(II) adsorption are given in .
Table 3. Kinetic parameters for adsorption of Ni(II) on CS/PRh nanocomposite.
4. Conclusions
In this work, CS/PRh nanocomposite by in situ chemical oxidation polymerisation of rhodanine in chitosan aqueous solution was prepared and characterised. The Ni(II) adsorption behaviour on the prepared CS/PRh nanocomposite has been studied under various conditions of different solution pH values, adsorption contact times, amount of adsorbent and temperature. Optimal adsorption conditions of Ni(II) were found at pH 8, and contact time of 3 hours, with maximum adsorption capacity of and
according to the Langmuir and Sips isotherm models, respectively. The Langmuir and Sips model was found to fit well with the experimental data. Thermodynamically, the adsorption of Ni(II) is spontaneous
and exothermic
process. Kinetically, the adsorption of Ni(II) onto CS/PRh nanocomposite obeyed the pseudo-second-order model. The overall results demonstrated the potential utility of the nanocomposite for Ni(II) removal from aqueous solutions.
Acknowledgements
This study was supported by the Chemical Engineering Department of Babol University of Technology in Iran. The authors wish to thank the Director, polymer laboratory, for his kind permission to publish this paper.
No potential conflict of interest was reported by the authors.
Disclosure statement
Reference
- Katal R, Pahlavanzadeh H. Zn(II) ion removal from aqueous solution by using a polyaniline composite. J Vinyl Additive Technol. 2011;17:138–145.
- Borba CE, Guirardello R, Silva EA, Veit MT, Tavares CRG. Removal of nickel(II) ions from aqueous solution by biosorption in a fixed bed column: experimental and theoretical breakthrough curves. Biochem Eng J. 2006;30:184–191.
- Song J, Oh H, Kong H, Jang J. Polyrhodanine modified anodic aluminum oxide membrane for heavy metal ions removal. J Hazard Mater. 2011;187:311–317.
- Ghorbani M, Eisazadeh H. Removal of COD, color, anions and heavy metals from cotton textile wastewater, by using polyaniline and polypyrrole nanocomposites coated on rice husk ash. Compos Part B. 2013;45:1–7
- Kousalya GN, Muniyappan RG, Sairam SC, Meenakshi S. Synthesis of nano- hydroxyapatite chitin/chitosan hybrid biocomposites for the removal of Fe(III). Carbohydr Polym. 2010;82:549–599.
- Song J, Kong H, Jang J. Adsorption of heavy metal ions from aqueous solution by polyrhodanine-encapsulated magnetic nanoparticles. J Colloid Interface Sci. 2011;359:505–511.
- Yan H, Yang L, Yang Z, Yang H, Li A, Cheng R. Preparation of chitosan/poly (acrylic acid) magnetic composite microspheres and applications in the removal of copper (II) ions from aqueous solutions. J Hazard Mater. 2012;229–230:371–380.
- Salam MA, Makki MSI, Abdelaal MYA. Preparation and characterization of multi-walled carbon nanotubes/chitosan nanocomposite and its application for the removal of heavy metals from aqueous solution. J Alloys Compd. 2011;509:2582–2587.
- Suc NV, Ly H. Lead (II) removal from aqueous solution by chitosan flake modified with citric acid via crosslinking with glutaraldehyde. J Chem Technol Biotechnol. 2013;88:1641–1649.
- Barros CF, Sousa W, Cavalcante M, Carvalho V, Dias S. Removal of copper, nickel and zinc ions from aqueous solution by chitosan-8-hydroxyquinoline beads. CLEAN-Soil, Air, Water. 2008;36:292–298.
- Paipitak K , Pornpra T, Mongkontalang P, Techitdheer W, Pecharapa W. Characterization of PVA-chitosan nanofibers prepared by electrospinning. Procedia Eng. 2011;8:101–105.
- Spasova M, Manolova N, Paneva D, Rashkov I. Preparation of chitosan-containing nanofibres by electrospinning of chitosan/poly(ethylene oxide) blend solutions. E-Polym. 2004;56:1–12.
- Li W, Jang DM, An SY, Kim D, Hong SK, Kim H. Polyaniline-chitosan nanocomposite: High performance hydrogen sensor from new principle. Sensor Actuat B Chem. 2011;160:1020–1025.
- Li Y, Li G, Peng H, Chen K. Facile synthesis of electroactive polypyrrole–chitosan composite nanospheres with controllable diameters. Polym Int. 2011;60:647–651.
- Hu Y, Jiang X, Ding Y, Ge H, Yuan Y, Yang C. Synthesis and characterization of chitosan–poly(acrylic acid) nanoparticles. Biomaterials. 2002;23:3193–3201.
- Fernandes SCM, Oliveira L, Freire CSR, Silvestre AJD, Neto CP, Gandini A, Desbriéres J. Novel transparent nanocomposite films based on chitosan and bacterial cellulose. Green Chem. 2009;11:2023–2029.
- Kong H, Jang J. Synthesis and antimicrobial properties of novel silver/polyrhodanine nanofibers. Biomacromolecules. 2008;9:2677–2681.
- Yu D, Wang W, Wu J. Preparation of conductive wool fabrics and adsorption behavior of Pd (II) ions on chitosan in the pre-treatment. Synth Met. 2011;161:124–131.
- Salmah H, Faisal A, Kamarudin H, Hanafi I. Mechanical and thermal properties of chitosan‐filled polypropylene composites: the effect of acrylic acid. J Vinyl Additive Technol. 2011;17:125–131.
- Kong H, Song J, Jang J. One-step fabrication of magnetic c-Fe2O3/polyrhodanine nanoparticles using in situ chemical oxidation polymerization and their antibacterial properties. Chem Commun. 2010;46:6735–6737.
- Ramya R, Sankar P, Anbalagan S, Sudha PN. Adsorption of Cu(II) and Ni(II) ions from metal solution using crosslinked chitosan-g-acrylonitrile copolymer. Int J Environ Sci. 2011;6:1326–1338.
- Kumar M, Tripathi BP, Shahi VK. Crosslinked chitosan/polyvinyl alcohol blend beads for removal and recovery of Cd(II) from wastewater. J Hazard Mater. 2009;172:1041–1048.
- Foo KY, Hameed BH. Insights into the modeling of adsorption isotherm systems. Chem Eng J. 2010;156:2–10.
- Cho DW, Jeon BH, Chon CM, Kim Y, Schwartz FW, Lee ES, Song H. A novel chitosan/clay/magnetite composite for adsorption of Cu(II) and As(V). Chem Eng J. 2012;200:654–662.
- Thinh NN, Hanh PTB, Ha LTT, Anh LN, Hoang TV, Hoang VD, Dang LH, Khoi NV, Lam TD. Magnetic chitosan nanoparticles for removal of Cr(VI) from aqueous solution. Mater Sci Eng C. 2013;33:1214–1218.