Abstract
Curcumin is an important anti-inflammatory natural compound with low bioavailability which is due to poor solubility and absorption. Solid lipid nanoparticles (SLNs) loaded with Curcumin were formulated and evaluated for physical parameters and in vitro/ex vivo permeation. Further the optimised SLN was assessed for pharmacokinetic/pharmacodynamic considerations. SLNs were formulated by emulsion-solvent evaporation technique and evaluated for physical properties and in vitro drug release. Selected SLNs were evaluated for stability and then characterised for pharmacokinetic parameters and anti-inflammatory activity with reference to a commercial formulation. Spherical SLNs were obtained in the size range of 102–156 nm with negative potential. C-SLN category has shown highest entrapment efficiency. The order of drug release was S-SLN > G-SLN > C-SLN. Selected SLN formulation C-SLN-3 has shown good stability under various conditions. C-SLN-3 has demonstrated highest drug permeation through human skin and 171.623 mg drug content permeated in 24 h. It has also shown lowest lag time 0.375 h. Similarly, it has shown maximum value for Cmax in in vivo determination and increased the bioavailability upto 68.12%. C-SLN-3 provided 90.75% edema inhibition in 6 h. Present study shows that nature of lipids and its physical-chemical properties are critical for SLN formulation and can be used for designing better drug delivery systems with optimum transdermal permeation.
1. Introduction
Curcumin, the major constituent of Curcuma longa (Family Zingiberaceae), has been reported to have anti-inflammatory,[Citation1] anti-oxidant,[Citation2,Citation3] anticarcinogenic,[Citation4] and hypocholesterolemic [Citation5] properties. Its anti-inflammatory properties have been reported to be based on its property to inhibit both lipoxygenase and cyclooxygenase enzymes. It is well tolerated in as high a dose as 2 gm/kg (peroral) in mice [Citation1]; however, photodegradation, short half-life and low bioavailability are major hurdles for the therapeutic use of Curcumin.[Citation6] Curcumin is unstable in the presence of light and basic pH and degrades within 30 min due to the presence of phenolic group and conjugated double bounds.[Citation7,Citation8] It shows poor absorption, following oral administration (up to 8 g per day) and only the traces of compound appear in blood.Citation[Citation9] It undergoes extensive first-pass metabolism.[Citation10] Several formulations have been reported for Curcumin, e.g. liposomes,[Citation11] Bionanovesicles,[Citation12] Nano-transfersomes,[Citation13] solid lipid nanoparticles (SLNs),[Citation14] transdermal film,[Citation15] microspheres,[Citation16] nanoemulsion,[Citation17] and so on.
Transdermal route can offer ease of access, avoidance of first pass and gastrointestinal disturbances; however, its use for systemic absorption is limited due to skins' barrier nature. Several techniques have been developed for enhanced permeation, e.g. iontophoresis,[Citation18] electroporation,[Citation19] ultrasound,[Citation20] ablation [CitationCitation21] or use of chemicals, e.g. alcohols,[Citation22] terpenes [CitationCitation23] and azones.[Citation24] Main mechanisms for these techniques are delipidization, hydration and disruption of the stratum corneum (SC) barrier or modifications in the thermodynamic status of drug/skin; however, these methods also imitate the skin irritation.
Lipid-based drug delivery systems form a significant category which is used to influence the absorption of poorly lipophilic or sometimes unstable compounds through different mechanisms for improved bioavailability. These drug delivery systems (DDS) are based on the concept of compatibility of excipients within the physiological system. SLNs have been considered a promising DDS particularly for incorporating a controlled-release profile into the DDS.[Citation25–28] The major features of SLNs are biocompatibility, drug targeting, drug release modulation and the scale up possibilities.[Citation29–32]
Ceramides are major lipids of SC and are critical due to its amount in skin and hydrophobicity. Another important observation for these lipids is their ability to form bilayers. Goñi and Alonso published a review on various properties of ceramides.[Citation33] Ceramide 3 or ceramide 2 (Cer 2) consists of a phytosphingosine backbone acylated with a saturated fatty acid (stearic acid).[Citation34,Citation35]
In this study, Curcumin-loaded SLNs were formulated using various lipids (glyceryl monostearate (GMS), stearic acid (SA) and ceramide 2:palmitic acid (PA)) and their physical parameters, transdermal permeation, stability and anti-inflammatory activity were evaluated. The most promising formulation was compared with an established, commercial transdermal anti-inflammatory formulation containing Diclofenac.
2. Materials and methods
2.1. Materials
Curcumin was the gift sample from Asoj Soft Caps, Baroda, India, whereas ceramide 2 (N-stearoyl-(2S,2R,3R)-2-amino-1,3-octadecandiol or N-stearoyl-DL-sphinganin) was the gift sample from Sederma, France, supplied by Croda Chemicals (India) Pvt. Ltd., Thane, Mumbai, India. Glyceryl monostearate (1-stearoyl-rac-glycerol), stearic acid (octadecanoic acid), palmitic acid and Tween 80 (Polysorbate 80) and ethanol were of analytical grade and were purchased from Sigma-Aldrich (New Delhi, India).
2.2. Methods
2.2.1. Preparation of nanoparticles
SLNs were formulated by modified emulsion/solvent evaporation method using the composition given in .
Table 1. Composition for SLN formulations.
Specified quantity of drug and lipid were weighed and dissolved in ethanol (10 ml). It was then heated upto 5 °C higher than melting temperature of lipid. On the other hand, Tween 80 and water were mixed together and heated upto same temperature. The aqueous phase was added to the hot oil phase and mixed with continuous stirring. Obtained dispersion was continually homogenised by mechanical agitation (Remi, New Delhi, India) for 10 min at 10,000 rpm.
The resultant dispersion was additionally sonicated above the melting point (m.p.) of oil phase at 100 W amplitude for 15 min using a probe sonicator to form nanodispersion. The nanodispersion was rapidly chilled by dipping the beaker into ice water (0 °C) to obtain nanoparticles. The SLNs were filtered through a 0.22 μm pore size membrane (Immobilon-P Membrane, Millipore Pvt. Ltd., New Delhi, India) and then dried.[Citation36]
2.2.2. Size and shape
Particle size and shape of SLN were determined by transmission electron microscopy (TEM). A drop of sample was located on a copper grid. A drop of negative stain, phosphotungstic acid (1% w/v) was added to the sample and allowed to absorb. Then it was dried and evaluated using Philips CM-10 transmission electron microscope (Acceleration voltage: 100 kV; Magnification: upto 450,000×; Cryo-attachment).
2.2.3. Zeta potential and polydispersity indices
Zeta potential and polyddispersity indices (PDI) were assessed by Zetasizer NanoZS (Malvern Instruments, UK) based on photon correlation spectroscopy. SLNs were suspended in lactate buffer (pH 5.5) and evaluated by Zetasizer.
2.2.4. Entrapment efficiency
Entrapment efficiency of the formulation was determined by estimating the drug content in a suitable aliquot of formulation after ultra filtration of a lysed sample. Total drug content of the sample was estimated by dissolving in methanol (5 ml) followed by vortexing (CM-101 PLUS, Cyclomixer, Remi, New Delhi, India). The lysed sample was diluted and analysed by high performance liquid chromatography (HPLC). Another aliquot was filtered through centrisart (MW cut-off 20 kDa) at 3500 rpm for 15 min and the drug content of the aqueous phase was determined by HPLC. The difference of both the evaluations was taken and entrapment efficiency was calculated.[Citation36]
2.2.5. Estimation of drug amount using HPLC
The drug content estimation for various procedures was done using HPLC analysis. The specifications of the HPLC system were Shimadzu LC-10AT VP pump, a SIL-10AF auto injector, an SPD-10A UV-Vis detector, and an SCL-10A VP system controller HPLC system with an analytical column of Shim-pack VP-ODS, 4.6 mm I.D. × 150 mm packed with 5 µm adsorbent particles (Shimadzu, Japan). Twenty microlitre sample was injected and eluted with acetonitrile and acetic acid (55:45, v/v/v) isocratically at a flow rate of 1.0 ml/min.[Citation36] Detection wavelength was set at 427 nm. A calibration curve was plotted between concentration and peak area.
2.2.6. Drug release through synthetic membrane
In vitro drug release was estimated using synthetic cellulose acetate membrane having a MW cut-off of 12 kDa (Himedia, Mumbai, India). The synthetic membrane was stabilised in pH 5.5 buffer at 37 ± 0.5 °C for 6 h and then fixed in Franz diffusion cells (3.14 cm2). As a medium, pH 5.5 buffer was filled in the receiver section. For the experiment, suitable quantity of test formulation (1 g) was applied onto the donor side. An aliquot of 1 ml was removed at predetermined time periods and analysed by HPLC. Fresh buffer was added to maintain the volume. The drug content was estimated using assay.[Citation36]
2.2.7. Stability
For stability assessment, formulations were stored at 5 ± 3 ºC and 25 ºC ± 2 ºC/60% ± 5% relative humidity (RH), respectively, for 180 days. After this, the formulations were tested for physical parameters and in vitro drug release.[Citation36]
2.2.8. Incorporation of SLN into gel
SLNs were formulated into gels using carbopol 934. Specified quantity of carbopol 934 was dispersed in distilled water to make 1% dispersion. The dispersion was homogenised by mechanical stirrer for 30 min. Then, 0.5% v/v solution of triethanolamine was added to the dispersion for its neutralisation and kept overnight. After this, SLNs were added and drug concentration was kept at 1% w/w.
2.2.9. Viscosity
Brookfield DV III ultra V6.0 RV cone and plate rheometre (Brookfield Engineering Laboratories, Inc., Middleboro, MA) was employed for the determination of viscosity. The spindle # CPE40 was used to determine viscosity of gels at 25 ± 0.5 °C.
2.2.10. Skin permeation (ex vivo studies)
Ex vivo drug permeation was studied using full thickness human skin acquired from plastic surgery patients. For the experiment, appropriate procedure was designed and approved by institutional ethical committee. Subcutaneous tissues attached with the skin were removed by surgical scalpel and skin was washed with Ringers' solution. Then it was desiccated and stored in polyethylene bag at −20 °C, wrapped in aluminium foil.
Prior to the experiment, skin was defrosted and cleaned with Ringers' solution. It was then fixed onto the Franz cell (3.14 cm2). Buffer pH 5.5 was filled in the receiver chamber and the set-up was equilibrated overnight. Then, suitable dose of test formulation was applied onto the epidermal side of skin (1 g = commercial emulgel (CEG), Curcumin unentrapped gel (CUG); 500 mg = G-SLN-3, S-SLN-3, C-SLN-3). Adequate samples were removed at predetermined interval and analysed by HPLC. At every sampling, the buffer was supplemented with fresh buffer to maintain the volume.[Citation36,Citation37]
2.2.11. Pharmacokinetic parameters (in vivo skin permeation)
In vivo experiment was performed on Wistar albino rats. Twenty four albino rats (average weight 255 g) of 8–10 weeks age were segregated in four groups and maintained under standard laboratory conditions (temperature: 25 ± 2 °C; RH: 55 ± 5%), with free access to diet (Lipton feed, Mumbai, India) and water. For transdermal application of the test formulation, rats were sedated by combination I.V. of ketamine hydrochloride (75 mg/kg) and xylazine (5 mg/kg). Abdominal area was cleaned with water and abdominal hairs were removed. Group I received 1 g (1.16% drug) of commercial gel while other three groups received 100 mg formulation (1% w/w) of G-SLNG-3, S-SLNG-3 and C-SLNG-3 respectively. The blood samples were obtained at pre-decided time periods and plasma was isolated by centrifugation.[Citation38] Drug content was determined by HPLC assay.
2.2.12. Pharmacodynamic (anti-inflammatory activity by edema inhibition)
Anti-inflammatory activity was determined by rat paw edema method and edema was induced by carrageenan in Wistar albino rats. Experimental procedure was designed and approved by institutional animal ethical committee. The formulations were evaluated against a positive control, oral Indomethacin. Commercial Diclofenac transdermal emulgel was taken as a demonstrative reference. Thirty rats were divided into five groups of six rats and fasted overnight before experiment. Initial paw volumes were measured and treatments were applied as per the following scheme. Group I received Indomethacin (10 mg/Kg; peroral), Group II received 1 g commercial gel while III, IV and V group received 100 mg formulation (G-SLNG-3/S-SLNG-3/C-SLNG-3). Carrageenan suspension (1%) was prepared 1 h before experiment and 0.1 ml was administered into the plantar side of right hind paw of rat. Paw volume readings were taken at 1, 2, 3, 4, 5 and 6 h after carrageenan injection using digital plethysmograph. The untreated paw served as negative control. Percentage edema inhibition was calculated by using formula given in data analysis.[Citation38]
2.2.13. Skin irritation studies
Skin irritation study was performed on human males whom have been properly educated about the procedure. The procedure was approved by institutional ethical committee and consent forms were signed by the participants.
Twenty-four male in four groups were used and their upper arm area was carefully scanned for any irregularities. SLNs were tested against 5% sodium lauryl sulfate (SLS) as positive control and untreated skin as negative control. SLN gels were applied onto the skin and covered with a dressing. Next day, the dressing was removed and skin was cleaned with swab. The observations were made and given a score (0, no reaction; 1, weak spotty or diffuse erythema; 2, weak but well perceptible erythema covering the total exposure area; 3, moderate erythema; 4, severe erythema with edema; 5, very severe erythema with epidermal defects (blisters, erosions, etc.)). The procedure was followed for seven days.[Citation36]
2.2.14. Data and statistical analysis
2.2.14.1. Skin permeation (ex vivo study)
Ex vivo drug permeation data was plotted as cumulative amount of drug permeated versus time and several parameters were calculated such as steady state drug flux (Jss), lag time (Tlag), permeability coefficient through the membrane (Kp), distribution parameter within the membrane (D). The plots were regressed to yield linear curve and the slope of the linear portion and its x-intercept were taken as Jss and Tlag, respectively. D and Kp were calculated using following equations:
where D = distribution parameter (cm2 h−1), h = path length and Cd = initial drug concentration.
2.2.14.2. Pharmacokinetic parameters
Several pharmacokinetic parameters such as Cmax, tmax, AUC (area under curve), MRT (mean residence time) and Ke were evaluated by plotting plasma drug concentration (μg) against time (h). Peak plasma concentration (Cmax) and time of its occurrence (tmax) were directly read from the plasma concentration time profiles. AUC0→24 was calculated using linear trapezoidal method in Graph pad Prism Version 4. Mean residence time and elimination constant were calculated using in vivo data.
2.2.14.3. Pharmacodynamic (anti-inflammatory activity)
Inhibition of carrageenan-induced edema was used for anti-inflammatory activity determination. Per cent edema inhibition was calculated by following formula:
where Tc = thickness of paw in control and Tt = thickness of paw in treatment group
The experiments for determining physical parameters and in vitro drug release were performed in triplicate and ex vivo/in vivo drug permeation and anti-inflammatory activity were analysed using six experiments' data. Data is represented as mean ± S.D and analysed by Graph pad Prism Version 4 software by employing analysis of variance or the paired t-test as required with a statistical significance of p ≤ 0.05.
3. Results
3.1. Physical characterisation
All the lipid compositions effected in the formation of SLNs which were spherical and in the size range of 102–156 nm. In each category, size decreased serially. Among all categories, largest and smallest particle size were respectively S-SLN and C-SLN categories ().
Figure 1. Transmission electron microscopic photographs of (A) G-SLN-3, (B) S-SLN-3 and (C) C-SLN-3 formulations (D82£ 10,000).
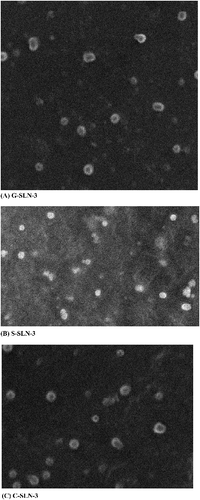
C-SLN has shown lowest PDI at 0.187 indicating consistent size distribution. Surface charge values were evaluated in form of zeta potential which was in the range from − 20 to − 38 mV. Highest Entrapment efficiency was shown by C-SLN-3 ().
Table 2. Physical characterisation of SLN and corresponding gels.
3.2. Drug release through synthetic membrane
In vitro drug release profiles for 24 h have been shown in . Highest drug release was observed in S-SLN-1 which released 93.12% drug in 24 h and minimum drug release occurred in C-SLN-3 which has shown 59.49% drug release. The drug release range for various SLN categories are 59.49%–67.14%, 76.78%–88.01% and 83.16%–93.12%, respectively, for C-SLN, G-SLN and S-SLN in that order.
3.3. Stability studies
For stability studies, SLNs were selected on the basis of drug content retained. Several changes were observed in the formulations during storage. Most significant alterations in particle size were detected in G-SLN-3 as its particle size changed from 123.1to 148.3 nm. S-SLN-3 has shown modification in entrapment efficiency from 60.94% to 43.1%. Zeta potential of all the formulations has shown slight changes on storage at 25 °C. In vitro drug release was also affected by storage at higher temperature and S-SLN and G-SLN both have shown the increment in drug release to the tunes of 13%. Among all the formulations tested, C-SLN-3 was found to be most stable formulation as it has shown almost nonsignificant changes in physical parameters and release ().
Table 3. Stability studies of selected SLN formulations.
3.4. Skin permeation (ex vivo studies)
Ex vivo drug permeation for SLN formulations was studied in comparison with Curcumin gel through full thickness human skin (). The permeation profiles were generated by plotting amount of drug permeated through unit area of skin against time.
It is to be noted that all SLN formulations have shown the drug permeation upto 24 h whereas CUG and CEG have shown drug release only upto 14 h (on x-axis of ). Moreover, highest drug permeation was detected in C-SLN-3 which has shown 171.623 μg drug permeated. Drug flux, lag time, permeability coefficient, diffusion parameter were computed on the basis of ex vivo permeation data (). C-SLNG-3 has shown highest flux (7.8356 μg/cm2/h) and lowest lag time of 0.375 h.
Table 4: Permeation parameters of the CEG, CUG and different SLN formulations.
3.5. Pharmacokinetic parameters (in vivo skin permeation)
Pharmacokinetic parameters of selecetd SLN formulations were evaluated in rats and compared with CEG (). C-SLNG-3 has shown highest Cmax value (335.8 μg/ml) and also increased tmax upto 8 h. SLN formulations in general have shown increase in tmax showing the controlled release nature of SLNs. The absolute bioavailability was enhanced from less than 1% (CEG) to 68.12% (C-SLNG-3).
3.6. Pharmacodynamic (edema inhibition)
Anti-inflammatory activity of SLN formulations was evaluated by inhibition of carrageenan-induced paw edema. All the SLN formulations have shown good edema inhibition and maximum edema inhibition was shown by C-SLNG-3 as it inhibited 90.75% edema in 6 h duration ().
Table 5. Pharmacokinetic parameters of the CEG and selected SLN formulations.
3.7. Skin irritation
None of the SLN formulations have shown any major skin irritation as shown in SLS treated group. The group treated with C-SLNG-3 showed significant enhancement in aesthetic appearance of skin.
4. Discussion
SLN is an effective lipid-based drug delivery system for delivering lipophilic drugs since they can improve the solubility of such drugs. However, physical characterisation of the formulation is also important because it can affect the stability and also their course of mechanism. In the present study, SLNs of Glyceryl monostearate, stearic acid and ceramide 2:palmitic acid loaded with Curcumin were designed for enhanced delivery of Curcumin via transdermal application.
Glyceryl monostearate, stearic acid and ceramide 2: PA were major lipid components of their respective formulations and Tween 80 was used as stabilising surfactant in all the formulation albeit at varying concentration. As shown in the results, all the compositions yielded SLNs but their sizes vary. The nanoparticle size depends on lipid content as well as surfactant ratio. It has been reported that surfactants reduce interfacial tension between lipid component and drug thereby stabilising the entire system. Stability of such systems is also favoured by finer particle size. Surfactant generates a protective layer around the SLN structure. As evidenced by the physical characterisation of SLNs by means of TEM and PCS, particle size decreased as the content of surfactant increases in the formulation.
Zeta potential is another physical parameter which has a critical role in stability of the formulation. It is also strongly affected by surfactant concentration. Zeta potential increase in each category could be due to increase in surfactant content and surface area due to decreased particle size. Also, Cer 2 bears a positive charge which affects the resultant zeta potential. Entrapment efficiency is dependent on the content of lipid and usually increases with increase in lipid content; however, nature of lipid plays important part in it. GMS and SA are single hydrocarbon chain containing lipids and Cer 2 has two hydrocarbon chains of asymmetrical lengths. Entrapment efficiency of SLN formulations has shown effect of lipid type. In G-SLN and S-SLN, drug content is held between the chains of two adjacent lipid molecules. These molecules have independent degrees of freedom and cause drug leakage during storage especially at elevated temperatures evidenced by entrapment efficiency and drug release after stability studies. C-SLN has both the chains in single molecule and its movement is restricted. This restricted movement helps in retaining higher drug quantity. PDIs have shown a reducing trend with increasing content of lipid which means that increased lipid content give more uniformly sized SLN.
In vitro drug release has also shown the effect of lipid structure. G-SLN and S-SLN have shown higher drug release which could be due to leaching of drug from respective SLN. However, more clear illustration of transdermal permeation is obtained from ex vivo permeation. Present study deals with the development of Curcumin-loaded SLN for transdermal delivery; however, since no transdermal formulation of Curcumin is available in the market the developed formulations were evaluated in reference to a commercial transdermal anti-inflammatory formulation. Highest drug permeation through human skin was observed in C-SLNG which has shown lowest drug release during in vitro study which means that in vitro drug release cannot always be taken as a measure of permeation. During transdermal permeation, skin's role is very critical since it is the rate limiting step. C-SLNG contains ceramide 2 as main lipid component which is the second largest component of lipid matrix of SC. It can interact with skin lipids and promote the movement of drug molecules inside skin layers which is evidenced by high values of flux and permeability coefficient. Pharmacokinetic parameters bear testimony to the results of ex vivo drug permeation. C-SLNG-3 has shown highest Cmax and AUC among all tested formulations. Based on the higher value of AUC, it can be said that Curcumin has shown good bioavailability when applied as a ceramide 2-palmitic acid SLN gel.
5. Conclusion
Three different SLN formulations were formulated and evaluated for transdermal permeation. The work ascertained that physicochemical properties of major lipid components as well as surfactant ratio govern the properties of SLN and also can predict its course of action. Ceramide:palmitic acid SLNs have shown optimum properties which could be employed for designing advanced formulations having adequate transdermal permeation.
5.1. Future perspective
Ceramide 2 is a natural lipid, belonging to the category of sphingolipids. It has earlier been used for cosmetic applications for its rejuvenative and antiaging properties. This study presents its use as a critical excipient for enhancing transdermal permeation of a natural compound, Curcumin. Ceramide 2 can be established as a novel lipid in already assorted lipid pharmaceutical excipients category.
5.2. Executive summary
Curcumin-loaded SLN were formulated using three different lipids and assessed for various properties and transdermal permeation. SLNs made up of ceramide 2:palmitic acid show desirable attributes in various evaluation procedures. The presence of ceramide 2 critically affects the properties and permeation characteristics of its SLN formulation. The optimised formulation was also found to be considerably stable and dermatologically acceptable.
Acknowledgements
The authors want to acknowledge Croda India and Sederma, France for genuine supply of ceramide 2 and Asoj Soft caps for providing Curcumin respectively. Further, we acknowledge the sophisticated analytical instrumentation facility (SAIF), AIIMS, New Delhi for TEM analysis and Advanced centre for nanotechnology (ACN), National Institute of Pharmaceutical Education & Research (NIPER) for providing facility of Zetasizer nano ZS.
Disclosure statement
The authors declare that they have no conflict of interest.
References
- Srimal RC, Dhawan BN. Pharmacology of diferuloyl methane (Curcumin), a non-steroidal anti-inflammatory agent. J Pharm Pharmacol. 1973;25:447–452.
- Sreejayan, Rao MNA. Curcuminoids as potent inhibitors of lipid peroxidation. J Pharm Pharmacol. 1994;46:1013–1016.
- Anto RJ, Kuttan G, Babu KVD, Rajasekharan KN, Kuttan R. Anti inflammatory activity of natural and synthetic curcuminoids. Pharm Pharmacol Commun. 1998;4:103–106.
- Huang MT, Smart RC, Wong CQ, Conney AH. Inhibitory effect of curcumin, chlorogenic acid, caffeic acid, and ferulic acid on tumor promotion in mouse skin by 12-O-tetradecanoylphorbol-13-acetate. Cancer Res. 1988;48:5941–5946.
- Rao DS, Sekhara NC. Satyanarayana MN, Srinivasan M, Effect of curcumin on serum and liver cholesterol levels in the rat. J Nutr. 1970;100:1307–1315.
- Mulik RS, Mönkkönen J, Juvonen RO, Mahadik KR, Paradkar AR. Transferrin mediated solid lipid nanoparticles containing curcumin:enhanced in vitro anticancer activity by induction of apoptosis. Int J Pharm. 2010;398:190–203.
- Wang YJ, Pan MH, Cheng AL, Lin LI, Ho YS, Hsieh CY. Stability of curcumin in buffer solutions and characterization of its degradation products. J Pharm Biomed Anal. 1997;15:1867–1876.
- Tønnesen HH, Karlsen J, Henegouwen GBv. Studies on curcumin and curcuminoids. VIII. Photochemical stability of curcumin. Z Lebensm Unters Forsch. 1986;183:116–122.
- Ravindranath V, Chandrasekhara N. Absorption and tissue distribution of curcumin in rats. Toxicology. 1980;16:259–265.
- Asai A, Miyazawa T. Occurrence of orally administered curcuminoid as glucuronide and glucuronide/sulfate conjugates in rat plasma. Life Sci. 2000;67:2785–2793.
- Preetha A, Kunnumakkara AB, Newman RA, Aggarwal BB. Bioavailability of Curcumin: problems and promises. Mol Pharm. 2007;4(6):807–818.
- Castangia I, Nácher A, Caddeo C, Valenti D, Fadda AM, Díez-Sales O, Ruiz-Saurí A, Manconi M. Fabrication of quercetin and curcumin bionanovesicles for the prevention and rapid regeneration of full-thickness skin defects on mice. Acta Biomater. 2014;10:1292–1300.
- Chaudhary H, Kohli K, Kumar V. Nano-transfersomes as a novel carrier for transdermal delivery. Int J Pharm. 2013;454:367–380.
- Tiyaboonchai W, Tungpradit W, Plianbangchang P. Formulation and characterization of curcuminoids loaded solid lipid nanoparticles. Int J Pharm. 2007;337:299–306.
- Vidyalakshmi K, Rashmi KN, Pramod Kumar TM, Siddaramaiah. Studies on formulation and in vitro evaluation of PVA/Chitosan blend films for drug delivery. J Macromol Sci Pure Appl Chem. 2004;41:1115–1122.
- Kumar V, Lewis SA, Mutalik S, Shenoy DB, Venkatesh M, Udupa N. Biodegradable microspheres of curcumin for treatment of inflammation. Indian J Physiol Pharmacol. 2002;46:209–217.
- Wang X, Jiang Y, Wang Y-W, Huang M-T, Ho C-T, Huang Q. Enhancing anti-inflammation activity of curcumin through O/W nanoemulsions. Food Chem. 2008;108:419–424.
- Batheja P, Thakur R, Michniak B. Transdermal iontophoresis. Expert Opin Drug Deliv. 2006;3:127–138.
- Denet A-R, Vanbever R, Préat V. Skin electroporation for transdermal and topical delivery. Adv Drug Deliv Rev. 2004;56:659–674.
- El-Kamel AH, Al-Fagih IM, Alsarra IA. Effect of sonophoresis and chemical enhancers on testosterone transdermal delivery from solid lipid microparticles: an in vitro study. Curr Drug Deliv. 2008;5:20–26.
- Lee JW, Gadiraju P, Park J-H, Allen MG, Prausnitz MR. Microsecond thermal ablation of skin for transdermal drug delivery. J Control Release. 2011;154:58–68.
- Babu RJ, Singh M, Kanikkannan N. Fatty alcohols and fatty acids. In: Smith EW, Maibach HI, editors. Percutaneous penetration enhancers. Boca Raton, FL: CRC Press; Taylor & Francis Group; 2005. p. 137–158.
- Liu C-H, Chang F-Y, Hung D-K. Terpene microemulsions for transdermal curcumin delivery: effects of terpenes and cosurfactants. Colloids Surf B Biointerfaces. 2011;82:63–70.
- Phillips CA, Michniak BB. Transdermal delivery of drugs with differing lipophilicities using azone analogs as dermal penetration enhancers. J Pharm Sci. 1995;84:1427–1433.
- Jasper AW, Schultz NE, Truhlar DG. Analytic potential energy functions for simulating aluminum nanoparticles. J Phys Chem B. 2005;109:3915–3920
- Reithmeier H, Hermann J, Göpferich A. Development and characterization of lipid microparticles as a drug carrier for somatostatin. Int J Pharm. 2001;218:133–143.
- Morel S, Ugazio E, Cavalli R, Gasco MR. Thymopentin in solid lipid nanoparticles. Int J Pharm. 1996;132:259–261.
- Schwarz C, Mehnert W, Lucks JS, Müller RH. Solid lipid nanoparticles (SLN) for controlled drug delivery. I. Production, characterization and sterilization. J Control Rel. 1994;30:83–96.
- Nemmar A, Holme JA, Rosas I, Schwarze PE, Alfaro-Moreno E. Recent advances in particulate matter and nanoparticle toxicology: a review of the in vivo and in vitro studies. Biomed Res Int. 2013;2013:22.
- Varshosaz J, Ghaffari S, Mirshojaei SF, Jafarian A, Atyabi F, Kobarfard F, Azarmi S. Biodistribution of Amikacin solid lipid nanoparticles after pulmonary delivery. Biomed Res Int. 2013;2013:8.
- Falzarano MS, Passarelli C, Bassi E, Fabris M, Perrone D, Sabatelli P, Maraldi NM, Donà S, Selvatici R, Bonaldo P, Sparnacci K, Laus M, Braghetta P, Rimessi P, Ferlini A. Biodistribution and molecular studies on orally administered nanoparticle-AON complexes encapsulated with alginate aiming at inducing dystrophin rescue in MDX mice. Biomed Res Int. 2013;2013:13.
- Gaur PK, Mishra S, Purohit S. Development of aceclofenac nanovesicular system using biomaterial for transdermal delivery: physical characterization, ex vivo, in vivo, and anti-inflammatory studies. J Biomater Sci Polym Ed. 2013;24:2126–2141.
- Goñi FM, Alonso A. Biophysics of sphingolipids I. Membrane properties of sphingosine, ceramides and other simple sphingolipids. Biochim Biophys Acta. 2006;1758:1902–1921.
- Wartewig S, Neubert RHH. Properties of ceramides and their impact on the stratum corneum structure: a review. Skin Pharmacol Physiol. 2007;20:220–229.
- Gaur PK, Mishra S. Diclofenac loaded nanoparticles fabricated with biomaterial (ceramide 2) for transdermal delivery. Sci Adv Mater. 2014;6:736–745.
- Gaur PK, Purohit S, Mishra S. Solid lipid nanoparticles of Guggul lipid as drug carrier for transdermal drug delivery. Biomed Res Int. 2013;2013:10.
- Manconi M, Caddeo C, Sinico C, Valenti D, Mostallino MC, Biggio G, Fadda AM. Ex vivo skin delivery of diclofenac by transcutol containing liposomes and suggested mechanism of vesicle-skin interaction. Eur J Pharm Biopharm, 2011;78:27–35.
- Gaur PK, Purohit S, Kumar Y, Mishra S, Bhandari A. Ceramide-2 nanovesicles for effective transdermal delivery: development: characterization and pharmacokinetic evaluation. Drug Dev Ind Pharm. 2014;40:568–576.