Abstract
A H2O2/TiO2 P25 nanoparticle system has been examined and applied towards synthesis of a wide range of 2-substituted benzimidazole and benzothiazole derivatives from oxidative coupling of aromatic 1,2-phenylendiamine and 2-aminothiophenol with aromatic aldehydes bearing different types of substituents.
1. Introduction
Developing environmentally benign technologies that provide economical synthesis strategies is an area of research that is being vigorously pursued. Benzimidazole and benzothiazole derivatives are of wide interest because of their diverse biological activity and clinical applications. Several compounds containing the benzimidazole and benzothiazole scaffold have been used as antiparasitic,[Citation1] antimicrobial [Citation2,Citation3] (I) antitumoral,[Citation2,Citation3] (II) and antiglutamate/antipar-kinson agents [Citation4–6] (). The imidazole core is a common moiety in a large number of natural products and pharmacologically active compounds.[Citation7–11]
Many reports have been cited in the literature describing the formation of 2-arylbenzimidazoles [Citation12–23] and 2-arylbenzothiazoles.[Citation24–30] With respect of methods, many of these methods have some drawbacks such as tedious work-up procedures, long reaction times, low yields, drastic reaction conditions, co-occurrence of several side reactions and in some cases more than one step is involved in the synthesis of these compounds.
The toxic and/or hazardous properties of many solvents in conjunction with crucial environmental concerns, such as atmospheric emissions and contamination of water effluents, is restricting their use.[Citation31] Solvent-free reactions have many advantages: reduced pollution, low cost, and simplicity in process and handling.
Metal oxides play a very important role in many fields of science. In technological applications, metal oxides are used in the fabrication of microelectronic circuits, sensors, piezoelectric devices, fuel cells, coatings for the passivation of surfaces against corrosion, and design of catalysts.[Citation32]
In the emerging field of nanotechnology, the goal of preparing oxide nanostructures is to create new properties with respect to those of bulk species. At the nanoscale, metal oxides can exhibit unique physical and chemical properties due to their limited size and a high density of their corner or edge surface sites associated with the shape effect.[Citation32]
Nanocatalysis is a rapidly growing field which often employs nanoparticles (NPs) in a variety of organic and inorganic reactions.[Citation33] Among them, titanium oxide (TiO2) NPs are used in various industrial processes, bactericides,[Citation34] gas sensors,[Citation35] environmental protections [Citation36,Citation37] dielectric ceramics,[Citation38] photovoltaic solar cells,[Citation39] and absorbents.[Citation40,Citation41] In addition, there are several reactions which are catalysed by TiO2 NPs as catalyst due to its unique surface properties.[Citation42,Citation43] High activity, reusability, strong oxidising power, easy availability, nontoxicity, and long-term stability are other advantages of TiO2 NPs as a recyclable catalyst.[Citation44–46]
Diluted solution of hydrogen peroxide is a universal, ecologically clean and convenient way to handle reagents for different oxidations in the liquid phase.[Citation47,Citation48–51]
Because of the availability of a vast number of aldehydes, the condensation of phenylenediamines with aldehydes is general method for the synthesis of 2-substituted benzimidazoles. The aim of this study is to investigate the enhancement of efficiency of the nano-sized TiO2 for the synthesis of 2-arylbenzimidazole derivatives starting from 1,2-phenylenediamines and a wide variety of aryl aldehydes in the presence of H2O2 ().
2. Results and discussion
To optimise the reaction conditions, the reaction of benzaldehyde and 1,2-phenylendiamine was selected as model substrates to examine the effects of different amount of H2O2 and TiO2 NPs in solvent free at 50 °C under ambient-light conditions. The results are depicted in . The best result was obtained by carrying out the reaction with 1:1:4:0.1 1,2-phenylendiamine/aryl aldehyde/H2O2/TiO2 P25 NPs. Although acetonitrile and 1,4-dioxan were found to be effective, considering demanding environmental regulations, solvent-free reactions are desired. TiO2 P25 NPs is necessary for complete product formation because the absence of TiO2 P25 NPs resulted in an extremely sluggish reaction (, entry 1). In this case, even after 6 hours, imine derivative was obtained as only product. In contrast, the addition of a catalytic amount of TiO2 P25 NPs resulted in completion of reaction. It can be concluded that TiO2 P25 NPs activates the decomposition of H2O2.
Table 1. Effect of increasing amount of H2O2 and TiO2 NPs on the preparation of benzimidazoles.a
At room temperature, the reaction rate was found to be very slow and was increased with increase in temperature. At 50 °C, reaction rate was found to be maximum and further increase in temperature did not show any enhancement.
In comparison to the same reaction catalysed by the commercially available TiO2 bulk, TiO2 P25 NPs increased the product yield considerably (, entry 5). It has been well documented and recognised [Citation52–54] that TiO2 NPs have a large surface-to-volume ratio compared to bulk TiO2, making it more reactive to adsorb of H2O2 compared to bulk TiO2. Moderate to lower yields were obtained with TiO2 anatase (, entry 6) and TiO2 rutile (, entry 7) tested. This is in agreement with what was previously reported [Citation55–58] that mixtures of rutile and anatase (TiO2 P25 NPs) exhibit enhanced photocatalytic activity compared to the same isolated materials. Namely a transfer of photoexcited electrons and positive holes between interconnecting anatase and rutile crystals suppresses charge recombination and leads to the activity enhancement.[Citation59]
The activity of TiO2 P25 NPs was also examined under the optimised conditions. Thus, after the first run, which gave the desired product in 96% yield, after recovery the catalyst was subjected to a second oxidation reaction from which it gave the corresponding benzimidazole in 93% yield; the average chemical yield for 5 consecutive runs was 93%, which clearly demonstrates the practical recyclability of this catalyst.
To generalise our reagent system, the applicability of the H2O2/TiO2 P25 NPs system was then examined for the synthesis of 2-substituted benzimidazoles under the optimised reaction conditions and the results are presented in . As shown, both aldehydes bearing electron-donating and electron-withdrawing substituents gave desired benzimidazoles in excellent yields. Reaction of aromatic heterocyclic aldehydes with 1,2-phenylendiamine afforded the corresponding 2-heteroaryl benzimidazoles in excellent yields (, entries 7, 8).
Table 2. Direct synthesis of various 2-arylbenzimidazoles.a
It is pleasing to observe the remarkable stability of a variety of functional groups such as nitro, hydroxyl, halides formyl, conjugated C = C double bond and ketone under the reaction conditions. This procedure is also applicable to electron-deficient or electron-rich 1,2-phenylendiamines, which produced 2-arylbenzimidazoles smoothly in excellent yields (, entries 10–12).
The direct synthesis starting from 2-aminothiophenol and aryl aldehydes should be synthetically useful and practical. 2-Aminothiophenol and a variety of aryl aldehydes can be used in this oxidative cyclisation via the formation of Schiff bases, which leads to the direct synthesis of 2-arylbenzothiazoles ().
Table 3. Direct synthesis of various 2-arylbenzothiazoles.a
The optimum molar ratio of 2-aminothiophenol/aryl aldehyde/H2O2/TiO2 P25 NPs (1:1:4:0.1) is found to be ideal for the synthesis of 2-arylbenzothiazoles in solvent-free conditions at 50 °C under ambient-light conditions, while with lesser amounts, the reaction remains incomplete.
A series of structurally diverse aryl aldehydes was then reacted with this remarkably simple procedure, and the results of these studies are collected in . As can be seen, all substrates including aromatic, and heterocyclic aldehydes were converted to the desired 2-arylbenzothiazoles, and most of the reactions proceeded nearly quantitatively. As can be seen from the data in , the chemoselectivity of this process is similar to that of the synthesis of 2-arylbenzimidazole.
The preparation of 2-(4-formylphenyl)benzimidazole and 2-(4-formylphenyl)benzothiazole from 1,4-benzenedicarboxaldehyde has been of great interest, because, the remaining formyl group can be converted to other functional groups. Therefore, the reactions of 1,4-benzenedicarboxaldehyde with 1,2-phenylenediamine and 2-aminothiophenol were also investigated. The reactions of 1,4-benzenedicarboxaldehyde in the optimised conditions led to exclusively mono-benzimidazole and mono-benzothiazole in excellent yields (, entry 6 and , entry 9).
A comparison of the efficiency of this method with selected previously methods is collected in . The results show that this method is superior to some previously reported methods in terms of yields and reaction times.
Table 4. Comparison of methods for the synthesis of 2-arylbenzimidazoles and 2-arylbenzothiazoles.
Nevertheless, this protocol has its limitations. Acetaldehyde, an aliphatic aldehyde, only provided low to moderate yield of the desired product. 2-Aminophenol did not give the desired 2-substituted benzoxazoles in the reaction ().
Scheme 2. Reagents and conditions: H2O2 (4 mmol), TiO2 P25 NPs (0.1 mmol), and solvent free, 50 °C, under ambient-light conditions.
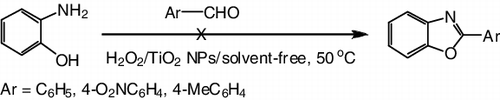
The suggested mechanism for this reaction is patterned in . We believe that the TiO2 NPs activated the aldehyde group through forming a coordinate bond with the Lewis acid site of a TiO2 surface. Nucleophilic addition by phenylene-1,2-diamine on the aldehyde produces intermediate (III) which exist in equilibrium with the cyclic hydrobenzimidazoles (IV) that is oxidised to benzimidazoles by hydrogen peroxide in the precnce of TiO2 NPs. The adsorption of H2O2 to the surface of TiO2 NPs followed by its cleavage to form the very oxidising hydroxyl radical (HO•).[Citation53] Then the oxidation may be initiated by a single electron transfer to hydroxyl radical to produce hydroxide ion and a radical cation V that subsequently loses a proton to generate a radical VI which in turn is attacked by hydroxyl radical to produce product in the reaction mixture. The additional hydrogen peroxide then undergoes partial decomposition to oxygen and water.
3. Experimental
Bulk TiO2 P25 (80% anatase, 20% rutile) as well as 1,2-phenylendiamines, 2-aminothiophenol, 2-aminophenol, and all the aldehydes employed and substrates are commercial products and were used without further purification. The nano-sized TiO2 (Aeroxide® P25, Sigma-Aldrich) has been selected for this study. The manufacturer reported primary TiO2 NP size to be 21 nm, with the specific surface area of 35–65 m2/g and the purity at least 95.5%. The particles exhibit a crystalline structure of anatase (80%) and rutile (20%).[Citation57] TiO2-anatase and TiO2-rutile NPs were purchased as a commercial product in powder form (from Sigma-Aldrich Co.) with a particle size of about 21 nm.
Melting points were determined in a capillary tube and are not corrected. 1H NMR and 13C NMR spectra were recorded on a Bruker-200 NMR spectrometer using tetramethylsilane (TMS) as internal standard.
3.1. General procedure for the synthesis of 2-substituted benzimidazoles and benzothiazoles
A mixture of 1,2-phenylenediamine (1 mmol), aryl aldehyde (1 mmol), 30% H2O2 (4 mmol, 0.4 mL) and TiO2 NPs (0.1 mmol, 0.008 g) was heated at 50 °C under ambient-light conditions. The progress of the reaction was monitored by TLC to determine the necessary reaction times (). After completion of the reaction, EtOH (15 mL) was added to the mixture, which was then heated for 5 min at 80 °C. The catalyst was removed by filtration and was reused in the next run. The filtrate was poured into ice water. The pure solid product was filtered, washed with ice-water and subsequently dried. An identical procedure was employed using 2-aminothiophenol (1 mmol), aryl aldehyde (1 mmol) in the presence of 30% H2O2 (4 mmol) and TiO2 NPs (0.1 mmol) for the synthesis of benzothiazoles ().
All products are known compounds and characterised easily by comparison with authentic samples except compound 6f (), whose spectral and analytical data are furnished. The identity and purity of the product was confirmed by 1H and 13C NMR spectroscopic analysis. Spectral data for new compound follows.
3.2. 2-(4-Formylphenyl)benzimidazole (, entry 6)
Solid, mp > 300 °C; 1H NMR (200 MHz, CDCl3): δ = 7.33–7.35 (m, 2 H), 7.68–7.79 (m, 2 H), 8.16 (d, J = 8.36 Hz, 2 H), 8.47 (d, J = 8.36 Hz, 2 H), 10.16 (s, 1 H), 13.29 (br, 1 H, NH); 13C NMR (50 MHz, CDCl3): δ = 113.3, 125.0, 129.7, 133.9, 136.3, 138.9, 151.6, 159.3, 193.3; Anal. Calcd for C14H10N2O: C, 75.65; H, 4.53; N, 12.60. Found: C, 75.5; H, 4.4; N, 12.5.
4. Conclusion
In summary, H2O2/TiO2 NPs system has been employed here as a novel, mild, and highly efficient reagent for the convenient preparation of benzimidazoles and benzothiazoles in excellent yields from 1,2-phenylenediamines and 2-aminothiophenol and a wide variety of aryl aldehydes. In addition, simple work-up procedure, low cost, environmental friendly procedure, easy availability and excellent chemoselectivity make this methodology a valid contribution to the existing processes in the field of benzimidazole and benzothiazole derivatives synthesis.
Acknowledgements
We are thankful to the Razi University Research Council for partial support to this work.
Disclosure statement
No potential conflict of interest was reported by the authors.
Additional information
Funding
References
- Navarrete-Vázquez G, Yepez-Mulia L, Hernández-Campos A, Tapia A, Hernández-Luis F, Cedillo R, González J, Martǐnez-Fernández M, Martǐnez-Grueiro M, Castillo R. Synthesis and antiparasitic activity of albendazole and mebendazole analogues. Bioorg Med Chem. 2003;11:4615–4622.
- Özden S, Atabey D, Yıldız S, Göker H. Synthesis and potent antimicrobial activity of some novel methyl or ethyl 1H-benzimidazole-5-carboxylates derivatives carrying amide or amidine groups. Bioorg Med Chem. 2005;13:1587–1597.
- Palmer, PJ, Trigg, RB, Warrington JV. Benzothiazolines as antituberculous agents. J Med Chem. 1971;14:248–251.
- Andrzejewska M, Yépez-Mulia L, Cedillo-Rivera R, Tapia A, Vilpo L, Vilpo J, Kazimierczuk Z. Synthesis, antiprotozoal and anticancer activity of substituted 2-trifluoromethyl- and 2-pentafluoroethylbenzimidazoles. Eur J Med Chem. 2002;37:973–980.
- Hall IH, Peaty NJ, Henry JR, Easmon J, Heinisch G, Purstinger G. Synthesis and biological]evaluation of some 2-substituted derivatives of benzimidazoles. Arch Pharm. (Weinheim) 1999;332:115–130.
- Gong B, Hong F, Kohm C, Bonham L, Klein P. Synthesis and SAR of 2-arylbenzoxazoles, benzothiazoles and benzimidazoles as inhibitors of lysophosphatidic acid acyltransferase-β. Bioorg Med Chem Lett. 2004;14:1455–1459.
- Greenlee WJ, Siegl PKS. Chapter 7. Anglotensln/Renln Modulators. Annu Rep Med Chem. 1992;27:59–68.
- Shilcrat SC, Mokhallalati MK, Fortunak JMD, Pridgen LN. A New regioselective synthesis of 1,2,5-trisubstituted 1H-imidazoles and its application to the development of eprosartan. J Org Chem. 1997;62:8449–8454.
- Rizzi JP, Nagel AA, Rosen T, McLean S, Seeger T. An initial three-component pharmacophore for specific serotonin-3 receptor ligands. J Med Chem. 1990;33:2721–2725.
- Shapiro G, Gomez-Lor B. Carboxylate protection for the synthesis of 4,5-disubstituted 1-methylimidazoles. J Org Chem. 1994;59:5524–5526.
- Adams LJ, Boehm JC, Kassis S, Gorycki PD, Webb EF, Hall R, Sorenson M, Lee JC, Ayrton A, Griswold DE, Gallagher TF. Pyrimidinylimidazole inhibitors of CSBP/P38 kinase demonstrating decreased inhibition of hepatic cytochrome P450 enzymes. Bioorg Med Chem Lett. 1998;8:3111–3116.
- Molander GA, Ajayi K. Oxidative condensations to form benzimidazole-substituted potassium organotrifluoroborates. Org Lett. 2012;14:4242–4245.
- Yeh C-M, Tung C-L, Sun C-M. Combinatorial liquid-Pphase synthesis of structurally diverse benzimidazole libraries. J Comb Chem. 2000;2:341–348.
- Kim Y, Kumar MR, Park N, Heo Y, Lee S. Copper-catalyzed, one-pot, three-component synthesis of benzimidazoles by condensation and C–N bond formation. J Org Chem. 2011;76:9577–9583.
- Panda SS, Malik R, Jain SC. Synthetic approaches to 2-arylbenzimidazoles: A review. Curr Org Chem. 2012;16:1905–1919.
- Coffinier D, Kaim LE, Grimaud L. New benzotriazole and benzimidazole scaffolds from Ugi−Smiles couplings of isocyanides. Org Lett. 2009;11:995–997.
- Cano R, Ramon, DJ, Yus M. Transition-metal-Free O-, S-, and N-arylation of alcohols, thiols, amides, amines, and related heterocycles. J Org Chem. 2011;76:654–660.
- Akamatsu H, Fukase K, Kusumoto S. New efficient route for solid-phase synthesis of benzimidazole derivatives. J Comb Chem. 2002;4:475–483.
- Hoesl CE, Nefzi A, Houghten RA. Parallel solid-phase synthesis of 2-imino-4-oxo-1,3,5-triazino[1,2-a]benzimidazoles via tandem aza-Wittig/heterocumulene-mediated annulation reaction. J Comb Chem. 2003;5:155–160.
- Vourloumis D, Takahashi M, Simonsen KB, Ayida BK, Barluenga S, Winters GC, Hermann T. Solid-phase synthesis of benzimidazole libraries biased for RNA targets. Tetrahedron Lett. 2003;44:2807–2811.
- Rathod SB, Lande MK, Arbad BR. Synthesis, Characterization and Catalytic Application of MoO3/CeO2-ZrO2 Solid heterogeneous catalyst for the synthesis of benzimidazole derivatives. Bull Korean Chem Soc. 2010;31:2835–2839.
- Jafarpour M, Rezaeifard A, Ghahramaninezhad M, Tabibi T. Reusable a-MoO3 nanobelts catalyzes the green and heterogeneous condensation of 1,2-diamines with carbonyl compounds. New J Chem. 2013;37:2087–2095.
- Kumar B, Smita K, Cumbal L, Debut A. Biogenic synthesis of iron oxide nanoparticles for 2-arylbenzimidazole fabrication. J Saudi Chem Soc. 2014;18:364–369.
- Zhang X, Zeng W, Yang Y, Huang H, Liang Y. Copper-catalyzed double C–S bonds formation via different paths: Synthesis of benzothiazoles from N-benzyl-2-iodoaniline and potassium sulfide. Org Lett. 2014;16:876–879.
- Kumar D, Mishra BB, Tiwari VK. Synthesis of 2-N/S/C-substituted benzothiazoles via intramolecular cyclative cleavage of benzotriazole ring. J Org Chem. 2014;79:251–266.
- Zhu Y-P, Jia F-C, Liu M-C, Wu A-X. A multipathway coupled domino strategy: Metal-free oxidative cyclization for one-pot synthesis of 2-acylbenzothiazoles from multiform substrates. Org Lett. 2012;14:4414–4417.
- Xue W-J, Guo Y-Q, Gao F-F, Li H-Z, Wu A-X. A novel self-sequence reaction Network involving a set of six reactions in one pot: the synthesis of substituted benzothiazoles from aromatic ketones and anilines. Org Lett. 2013;15:890–893.
- Hutchinson I, Jennings SA, Vishnuvajjala BR, Westwell AD, Stevens MFG. Antitumor benzothiazoles. 16. Synthesis and pharmaceutical properties of antitumor 2-(4-aminophenyl)benzothiazole amino acid prodrugs. J Med Chem. 2002;45:744–747.
- Bahrami K, Khodaei MM, Nejati A. Synthesis of 1,2-disubstituted benzimidazoles, 2-substituted benzimidazoles and 2-substituted benzothiazoles in SDS micelles. Green Chem. 2010;12:1237–1241.
- Yelwande AA, Navgire ME, Tayde DT, Arbad BR, Lande MK. SnO2/SiO2 Nanocomposite catalyzed one-pot synthesis of 2-arylbenzothiazole derivatives. Bull Korean Chem Soc. 2012;33:1856–1860.
- Tanaka F, Toda F. Solvent-free organic synthesis. Chem Rev. 2000;100:1025–1074.
- Fernandez-Garcia M, Martinez-Arias A, Hanson JC, Rodriguez JA. Nanostructured oxides in chemistry: characterization and properties. Chem Rev. 2004;104:4063–4104.
- Guo J-Z, Cui H, Zhou W, Wang W. Ag nanoparticle-catalyzed chemiluminescent reaction between luminol and hydrogen peroxide. J Photochem Photobiol A: Chem. 2008;193:89–96.
- Coleman HM, Marquis CP, Scott JA, Chin S-S, Amal R. Bactericidal effects of titanium dioxide-based photocatalysts. Chem Eng J. 2005;113:55–63.
- Guidi V, Caratta MC, Ferroni M, Martinelli G, Paglialonga L, Comini E, Sberveglieri G. Preparation of nanosized titania thick and thin films as gas-sensors. Sens Actuators B: Chem. 1999;57:197–200.
- Balasubramanian G, Dionysiou DD, Suidan MT, Baudin I, Lainé J-M. Evaluating the activities of immobilized TiO2 powder films for the photocatalytic degradation of organic contaminants in water. Appl Catal B: Environ. 2004;47:73–84.
- Ao CH, Lee SC. Indoor air purification by photocatalyst TiO2 immobilized on an activated carbon filter installed in an air cleaner. Chem Eng Sci. 2005;60:103–109.
- Titov AA, Krokhin VA, Zhulanov NK, Melnikov DL. Preparation of chemically active powders of niobium and titanium oxides and synthesis of dielectric ceramic materials. J Mater Process Technol. 1995;55:249–253.
- Huisman CL, Goossens A, Schoonman J. Preparation of a nanostructured composite of titanium dioxide and polythiophene: a new route towards 3D heterojunction solar cells. Synth Met. 2003;138:237–241.
- Pena ME, Korfiatis GP, Patel M, Lippincott L, Meng X. Adsorption of As (V) and As (III) by nanocrystalline titanium dioxide. Water Res. 2005;39:2327–2337.
- Esumi K. Adsorption and adsolubilization of surfactants on titanium dioxides with functional groups. Colloids Surf A: Physicochem Eng Asp. 2001;176:25–34.
- Huang H, Leung DYC. Complete oxidation of formaldehyde at room temperature using TiO2 supported metallic Pd nanoparticles. ACS Catal. 2011;1:348–354.
- Hosseini-Sarvari M. TiO2 as a new and reusable catalyst for one-pot three-component syntheses of α-aminophosphonates in solvent-free conditions. Tetrahedron. 2008;64:5459–5466.
- Yamazaki S. Selective synthesis of sulfoxides and sulfones by methyltrioxorhenium-catalyzed oxidation of sulfides with hydrogen peroxide. Bull Chem Soc Jpn. 1996;69:2955–2959.
- Reich HJ, Chow F, Peake SL. Seleninic acids as catalysts for oxidations of olefins and sulfides using hydrogen peroxide. Synthesis. 1978;299–441.
- Bortolini O, Furia FD, Modena G, Seraglia R. Theoretical determination of molecular structure and conformation. 14. Is bicyclo[6.2.0]decapentaene aromatic or antiaromatic? J Org Chem. 1985;50:2684–2688.
- Strukul G, editor. Catalytic oxidations with hydrogen peroxide as oxidant. Dordrecht: Taylor & Francis; 1992.
- Bahrami K. Selective oxidation of sulfides to sulfoxides and sulfones using hydrogen peroxide (H2O2) in the presence of zirconium tetrachloride. Tetrahedron Lett. 2006;47:2009–2012.
- Khodaei MM, Bahrami K, Khedri M. The efficient chemoselective MoO3-catalyzed oxidation of sulfides to sulfoxides and sulfone with H2O2. Can J Chem. 2007;85:7–11.
- Khodaei MM, Bahrami K, Karimi A. H2O2/Tf2O System: An efficient oxidizing reagent for selective oxidation of sulfanes. Synthesis. 2008;1682–1684.
- Noyori R, Aoki M, Sato K. Green oxidation with aqueous hydrogen peroxide. Chem Commun. 2003;1977–1986.
- Sanchez LD, Taxt-Lamolle SFM, Hole EO, Krivokapic A, Sagstuen E, Haugen HJ. TiO2 suspension exposed to H2O2 in ambient light or darkness: degradation of methylene blue and EPR evidence for radicaloxygen species. Appl Catal B: Environmental. 2013;142–143:662–667.
- Lousad CM, Johansson AJ, Brinck T, Jonsson M. Mechanism of H2O2 Decomposition on transition metal oxide surfaces. J Phys Chem C. 2012;116:9533–9543.
- Khin MM, Nair, AS, Babu VJ, Murugan R, Ramakrishna S. A review on nanomaterials for environmental remediation. Energy Environ Sci. 2012;5:8075–8109.
- Li L, Salvador, PA, Rohrer GS. Photocatalysts with internal electric fields. Nanoscale. 2014:6:24–42.
- Lei S, Duan, W. Highly active mixed-phase TiO2 photocatalysts fabricated at low temperature and the correlation between phase composition and photocatalytic activity. J Environ Sci. 2008;20:1263–1267.
- Ohno T, Sarukawa K, Tokieda K, Matsumura M. Morphology of a TiO2 photocatalyst (Degussa, P-25) consisting of anatase and rutile crystalline phases. J Catal. 2001;203:82–86.
- Ohtani B, Prieto-Mahaney OO, Li D, Abe R. What is Degussa (Evonik) P25? Crystalline composition analysis, reconstruction from isolated pure particles and photocatalytic activity test. J Photochem Photobiol A. 2010;216:179–182.
- Hurum, DC, Agrios, AG, Gray, KA, Rajh, T, Thurnauer, MC. Explaining the enhanced photocatalytic activity of Degussa P25 mixed-phase TiO2 using EPR. J Phys Chem B. 2003;107:4545–4549.
- Ben-Alloum A, Bougrin K, Soufiaoui M. Synthèse chimiosélective des benzimidazoles sur silice traitée par le chlorure du thionyle. Tetrahedron Lett. 2003;44:5935–5937.
- Ryabukhin SV, Plaskon AS, Volochnyuk DM, Tolmachev AA. Synthesis of fused imidazoles and benzothiazoles from (hetero)aromatic ortho-diamines or ortho-aminothiophenol and aldehydes promoted by chlorotrimethylsilane. Synthesis. 2006;3715–3726.
- Sharghi H, Aberi M, Doroodmand MM. Reusable cobalt (III)-salen complex supported on activated carbon as an efficient heterogeneous catalyst for synthesis of 2arylbenzimidazole derivatives. Adv Synth Catal. 2008;350:2380–2390.
- Bougrin K, Loupy A, Soufiaoui, M. Trois nouvelles voies de synthèse des dérivés 1,3-azoliques sous micro-ondes. Tetrahedron. 1998;54:8055–8064.
- Trivedi R, De SK, Gibbs RA. A convenient one-pot synthesis of 2-substituted benzimidazoles. J Mol Catal A: Chem. 2006;245:8–11.
- Bahrami K, Khodaei MM, Naali F. Mild and highly efficient method for the synthesis of 2-arylbenzimidazoles and 2-arylbenzothiazoles. J Org Chem. 2008;73:6835–6837.
- Eynde JJV, Delfosse F, Lor P, Haverbeke YV. 2,3-Dichloro-5,6-dicyano-1,4-benzoquinone, a mild catalyst for the formation of carbon-nitrogen bonds. Tetrahedron. 1995;51:5813–5818.
- Wattenberg LW, Page MA, Leong JL. Induction of increased benzpyrene hydroxylase activity by 2-phenylbenzothiazoles and related compounds. Cancer Res. 1968;28:2539–2544.
- Kodomari M, Tamaru Y, Aoyama T. Solvent-free synthesis of 2-aryl and 2-alkylbenzothiazoles on silica gel under microwave irradiation. Synth Commun. 2004;34:3029–3036.
- Paul S, Gupta M, Gupta R. Microwave-induced solvent-free synthesis of 2-arylbenzothiazoles using p–TsOH. Synth Commun. 2002;32:3541–3547.
- Boger DE. A convenient preparation of 2-substituted benzothiazoles. J Org Chem. 1978;43:2296–2297.
- Das B, Holla H, Srinivas Y. Efficient (bromodimethyl)sulfonium bromide mediated synthesis of benzimidazoles. Tetrahedron Lett. 2007;48:61–64.
- Chen Y-X, Qian L-F, Zhang W, Han B. Efficient aerobic oxidative synthesis of 2-substituted benzoxazoles, benzothiazoles, and benzimidazoles catalyzed by 4-methoxy-TEMPO. Angew Chem Int Ed. 2008;47:9330–9333.
- Gogoi P, Konwar D. An efficient and one-pot synthesis of imidazolines and benzimidazoles via anaerobic oxidation of carbon–nitrogen bonds in water. Tetrahedron Lett. 2006;47:79–82.
- Hein DW, Alheim RJ, Leavitt JJ. The use of polyphosphoric acid in the synthesis of 2-aryl- and 2-alkyl-substituted benzimidazoles, benzoxazoles and benzothiazoles. J Am Chem Soc. 1957;79:427–429.
- Ben-Alloum A, Bakkas S, Souflaoui M. Nouvelle voie de synthèse des 2-arylbenzothiazoles transfert d'electrons activé par micro-ondes. Tetrahedron Lett. 1997;38:6395–6592.