Abstract
Lignin, an abundant plant biopolymer, is known to possess antioxidant and UV protectant properties in its native state. Nanoparticles exhibit either improved or different properties corresponding to their bulk materials or parent polymers. In the present study, using nanoprecipitation method, dioxane lignin nanoparticles (DLNP) and alkali lignin nanoparticles (ALNP) are fabricated from two different sources of lignin, i.e., hardwood dioxane lignin (DL) extracted from subabul stems and softwood alkali lignin (AL) which is available as a commercial source. Both DLNP and ALNP were fabricated in spherical shape with mean size of 80–104 nm. Analysis of radical scavenging activity revealed that both DLNP and ALNP possess higher antioxidant activity when compared with their parent polymers DL and AL, respectively. UV protectant potential of DLNP and ALNP was validated by monitoring the survival rates of Escherichia coli upon UV-induced mortality. DLNP and ALNP were more efficient than DL and AL in protecting E. coli against UV-irradiation-induced mortality. However, upon irradiation for different time periods, DLNP offered pronounced protection for E. coli against UV when compared with ALNP. Thus, the present study demonstrates that the antioxidant and UV protection properties of DLNP can be exploited further in food, pharmaceutical and cosmetic industries.
1. Introduction
Exposure to UV radiation causes sunburns, immune suppression, premature aging, wrinkle formation and cancer to dermis and epidermis of the skin with generation of reactive oxygen species.[Citation1,Citation2] To protect our skin from such harmful effects of UV radiation, several cosmetic products are available in the market including sunscreens. In this scenario, natural compounds with antioxidant activity and photoprotective properties gained much attention in this direction.[Citation3,Citation4] Lignin is one among such natural compounds. It is a renewable second most abundant biopolymer available from plants after cellulose with characteristic antioxidant as well as UV protection properties.[Citation5–10] The antioxidant activity of lignin is known to play a crucial role to combat over chemical, biological and mechanical stresses that plants come across. Thus, the free radical scavenging ability of lignin attained promising application in various industries related to pharmaceutical, cosmetic and food processing.[Citation11] Lignin is used as an UV protectant of beet armyworm nucleopolyhedrovirus (NPV), a potential microbial agent against insect pests.[Citation12,Citation13] Further, lignin is known to (1) inhibit UV-radiation-induced mutagenicity in Euglena gracilis and (2) enhance the UV absorbance of several broad-spectrum sunscreens.[Citation14,Citation15]
Polymer nanotechnology is efficiently utilised in delivery of drugs, oligonucleotides or RNA interfering effectors. However, preparation of nanoparticles from polymers has become an important challenging component of this technology.[Citation16] Fabrication of nanoparticles from natural polymeric materials such as lignin gained prominence due to its environmental compatibility. Lignin is available in abundance as a waste material from industries like paper and pulp. Yet, its utilisation as nanoparticles is mostly limited and/or associated with patents.[Citation17] The studies of Frangville et al. [Citation18] have shown that nanoparticles synthesised from low sulphonated lignin were non-toxic towards microalgae and yeast. Thus, utilisation of lignin in various industrial formulations and consumer products such as sunscreens and paints would play an important role in reducing their potential hazardous risks on mankind. Nanoscale lignin prepared by supercritical antisolvent process was shown to possess enhanced antioxidant activity when compared to non-nanoscale lignin, due to their high surface-area-to-volume ratio with reference to their parent polymers.[Citation19]
The antioxidant and UV protectant properties of lignin are dependent on its genetic origin and method of isolation. Therefore, in the present study, dioxane lignin nanoparticles (DLNP) and alkali lignin nanoparticles (ALNP) were fabricated from two distinct sources of lignin, i.e., hardwood dioxane lignin (DL) and softwood alkali lignin (AL), isolated by different methods. Leucaena leucocephala (Lam.) de Wit. (subabul) stem cuttings were used to isolate DL using acidic DL method.[Citation20] AL, which is produced from paper and pulp industry by Kraft pulping process, was chosen for comparative analysis, due to its usage in many industrial applications. Subsequently, DLNP and ALNP were characterised and examined for their antioxidant and UV protectant properties with reference to DL and AL, respectively.
2. Materials and methods
2.1. Materials
AL and 2,2-diphenyl-1-picrylhydrazyl (DPPH) were purchased from Sigma-Aldrich, USA. Lichrosolv acetone was procured from Merck and double-distilled water was of Milli-Q grade.
2.2. Preparation of lignin nanoparticles
DLNP and ALNP were prepared from DL and AL, respectively, using nanoprecipitation method.[Citation21,Citation22] Initially, DL/AL (10 mg/ml) was dissolved in acetone and water (9:1, v/v) and filtered through 0.2-µm Supor membrane (Pall Scientifics, USA.) to remove undissolved particles. The filtrate was added rapidly (<1 min) to Milli-Q water (1:2) under constant stirring (300 rpm) using a microsyringe. After continuous stirring (10 min at 20 °C), the suspension was centrifuged at 1500 g (10 min at 20 °C) to remove aggregates. The supernatant was used for microscopic analysis to determine the size and morphology of nanoparticles. The stability of nanoparticles was expressed as their average size after 30 days storage at room temperature.
2.2.1. Yield of nanoparticles
The yield of DLNP and ALNP was determined by centrifuging (Beckman Coulter Optima L-90K Ultracentrifuge, Germany) the corresponding supernatant obtained above at 40,000 rpm for 30 min. The pellet obtained was dried in an oven at 70 °C and the yield of nanoparticles was reported as gravimetric weight of this pellet using an analytical balance (Sartorius, Germany).
2.2.2. Size and morphology
The size and morphology of DLNP and ALNP were examined under field emission scanning electron microscope (FESEM) and transmission electron microscope (TEM). For FESEM studies, a drop of DLNP/ALNP (obtained as described in 2.2) was added onto glass slide and spun for 10 sec at 4000 rpm using spin coater. The slide containing DLNP/ALNP was sputter-coated with gold for 3 min to provide electron beam conductivity. FESEM micrographs were obtained using 30 µm aperture size Carl-Ziess Ultra 55 instrument by applying electron high-tension voltage (3–5 kV). For TEM studies, a drop of nanosuspension (DLNP/ALNP) was spin-coated onto copper grid mesh substrate coated with carbon. This substrate was directly used for TEM (FEI Tecnai) analysis at an accelerating voltage of 200 kV without additional treatments. The size of DLNP/ALNP from FESEM and TEM micrographs was measured using Image J software (version 1.45). The value reported was an average of at least 150 nanoparticles from three to four independent experiments (duplicate/triplicates) performed on different days.
2.3. Physico-chemical characterisation
For physico-chemical characterisation, lyophilised (Labconco, USA) nanoparticles were used. UV spectra of DLNP and ALNP (0.0025% in Milli Q water) were recorded on an UV–Visible spectrophotometer (Shimadzu UV-1700 Pharmaspec, Japan) between 220 and 400 nm. For Fourier transform infrared (FTIR) spectra, DLNP/ALNP (5 mg) was mixed with 250 mg of potassium bromide and pellets were made. Infrared spectra of these pellets were recorded in transmittance mode on FTIR instrument (JASCO-4100 type A, USA) between 400 and 4000 cm−1. The data represented are an average of 100 scans and resolution of the spectra was maintained at 4 cm−1. For DSC, DLNP/ALNP was kept in aluminium pan and heated (10 °C/min) from 30 to 170 °C to determine their corresponding glass transition temperature (Tg). However, caution was taken to subject both DLNP and ALNP to a cycle of preheating from 30 to 170 °C (10 °C/min) which was followed by cooling (20 °C/min) to 30 °C, so as to remove any history effects pertaining to the corresponding nanoparticles. During all these steps, the N2 flow (60 ml/min) was maintained.
2.4. Antioxidant potential
Antioxidant potential of test compounds DL, AL, DLNP and ALNP was determined by monitoring the decrease in absorbance of DPPH radical at 517 nm.[Citation23] The commercial food antioxidants, butylated hydroxytoluene (BHT) and ascorbic acid (Asc), were used as reference compounds. DL, AL and BHT were dissolved in dimethyl sulfoxide (DMSO) while Asc was dissolved in water. Lyophilised DLNP and ALNP were dispersed in water. The test/reference compounds were incubated with 1.0 ml DPPH (in methanol) at a wide range of concentrations, and readings were taken after 30 min against DMSO or water, respectively. Blank was set up using methanol in lieu of the DPPH solution. IC50 values of test/reference compounds are represented as half of the maximum inhibitory concentration of DPPH radical scavenging activity.
2.5. Anti-UV activity test
Anti-UV activity of test compounds DL, AL, DLNP and ALNP was evaluated at a wide range of concentrations (25, 50, 100 and 150 µg) by monitoring the decrease in mortality rates (or increase in survival rates) of Escherichia coli upon UV irradiation. DL/AL was dissolved in DMSO while DLNP/ALNP was dispersed in water. All test compounds were filter sterilised (0.2 µm Supor membrane, Pall Scientifics, USA) before incubating with E. coli.
2.5.1. E. coli culture preparation
E. coli was grown overnight at 37 °C in LB broth in an orbital shaker (Orbitek, India) at 150 rpm. After 16–20 h, 100 µl of culture was inoculated into 10 ml of fresh Luria-Bertani (LB) broth and kept at 37 °C until the medium shows 0.7 optical density (OD) at 600 nm (Shimadzu UV-1700 Pharmaspec, Japan). This secondary culture was centrifuged at 5000 rpm for 10 min at 4 °C and E. coli pellet was washed twice with phosphate buffered saline (PBS). The final pellet of E. coli was suspended in 1.0 ml of PBS and serially diluted to 10−5 with the same buffer and used for UV irradiation.
2.5.2. UV irradiation
UV irradiation of E. coli (0.1 ml of 10−5 dilution) was performed in a laminar airflow chamber at a constant distance of 20 cm between UV lamp and culture (with or without test compounds) for 5 min. After UV irradiation, the culture (50 µl) was plated onto LB agar. The mortality (or survival) rate of E. coli was determined after incubating them at 37 °C for overnight. The agar plates containing E. coli without UV irradiation and test compounds are used as negative control (∼150–400 colonies). On the other hand, agar plates containing E. coli irradiated with UV in the absence of test compounds are used as a positive control (no colonies). Further, to validate the efficiency of test compounds in protecting against UV irradiation, E. coli were incubated with 150 µg of each of the test compounds under UV irradiation for different (5, 10 and 15 min) time periods, respectively. Plating was done as described above.
3. Results and discussion
3.1. Size and yield of nanoparticles
In the present study, nanoprecipitation method was employed to fabricate lignin nanoparticles. Nanoprecipitation method is a facile, less complex, less time-consuming and sensitive procedure which requires less instrumentation and non-toxic chemicals.[Citation24] The nanoparticle size and morphology were observed using FESEM and TEM. As observed in (a) and (c) and (a) and (c), DLNP and ALNP were fabricated in spherical shape with broad-range size distribution. The average sizes of DLNP and ALNP were found to be 104 ± 60 nm (FESEM)/80 ± 27 nm (TEM) and 104 ± 41 nm (FESEM)/82 ± 33 nm (TEM), respectively. Such broad-range size distribution is common when smaller polymeric nanoparticles are obtained by nanoprecipitation method. This is possibly explained as a competitive process when organic phase acetone containing lignin polymer is propagating into the surrounding aqueous medium, it tends to solidify before reaching the equilibrium size. On the other hand, if the propagation of organic phase containing lignin polymer continues further to more extent at the time of solidification, then the organic phase tends to disperse randomly leading to broad-range size distribution.[Citation25] The spherical shape of DLNP and ALNP was retained even after storage at room temperature for 30 days. However, the size of DLNP and ALNP increased marginally to 116 ± 64 (FESEM)/86 ± 29 nm (TEM) and 114 ± 52 nm (FESEM)/87 ± 28 nm (TEM), respectively, as shown in and and and . The histograms in and suggest that upon storage of DLNP or ALNP, the distribution of smaller size nanoparticles decreased while that of larger size nanoparticles increased. This marginal increase in size might be due to Ostwald ripening phenomenon which explains that nanoparticles with smaller size diffuse through solution and tends towards the surface of nanoparticles with larger size.[Citation26] However, the yield of DLNP (63%) was higher when compared with ALNP (33%). This wide difference in yield between DLNP and ALNP is possibly due to (1) low solubility of AL in acetone which resulted in the removal of its bulk material during filtration process and/or (2) formation of aggregates by ALNP during nanoprecipitation that was removed during subsequent centrifugation process.
Figure 1. FESEM micrographs with histograms of (a, c) DLNP and ALNP on the first day of preparation; (c, d) DLNP and ALNP stored at room temperature for 30 days. The results shown here are representative of three to four independent experiments performed on different days. Further details are as described in materials and methods.
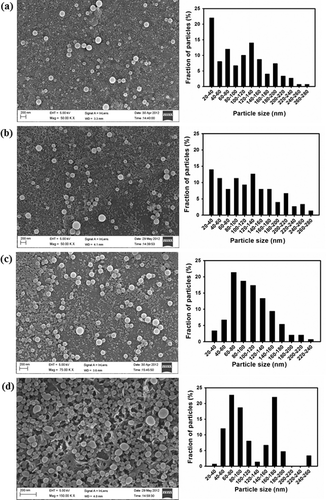
Figure 2. TEM micrographs with histograms of (a, c) DLNP and ALNP on the first day of preparation; (c, d) DLNP and ALNP stored at room temperature for 30 days. The results shown here are representative of three to four independent experiments performed on different days. Further details are as described in materials and methods.
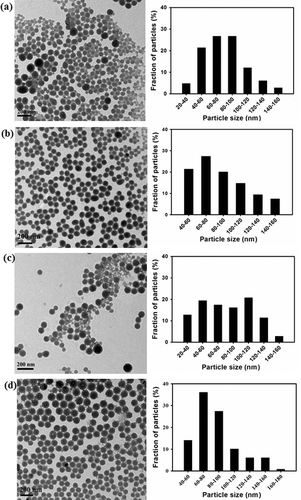
3.2. Physico-chemical characterisation
UV, FTIR and DSC data of DLNP and ALNP were analysed based on the data related to their parent polymers DL and AL, respectively.[Citation27] The UV spectra of both DLNP and ALNP showed absorption maxima at 281 nm accredited to guaiacyl (G) units and/or non-conjugated phenolic groups of lignin biomolecule ((a)). Further, the absorbance of ALNP was higher than DLNP corroborating well with its high guaiacyl content.[Citation28]
Figure 3. (a) UV, (b) FTIR and (c) DSC spectra of dioxane lignin nanoparticles (DLNP) and alkali lignin nanoparticles (ALNP). The results shown here are representative of three to four independent experiments performed on different days. Further details are as described in materials and methods.
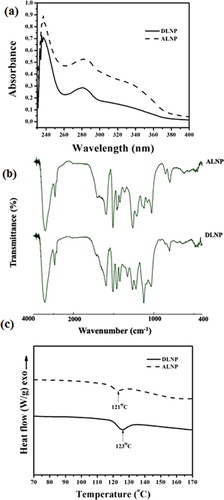
Bands detected in FTIR spectra at 1593–1605, 1505–1515 and 1422–1430 cm−1 in both DLNP and ALNP were attributed to aromatic ring vibrations of phenylpropanoid (C9) skeleton of lignin ((b)). Likewise, the band observed at 3435 cm−1 was attributed to O–H stretching, while the bands around 2935 and 2845 cm−1 were attributed to C–H stretch present in methyl, methylene and methoxyl groups in both DLNP and ALNP.[Citation29,Citation30] However, the relative intensity of methoxyl group band was higher in DLNP when compared to ALNP specifying that DLNP own more syringyl (S) units over guaiacyl (G) units. Further, bands detected in both DLNP and ALNP around 1269 and 820 cm−1 correspond to C=O stretch in guaiacyl unit and C–H out-of-plane vibrations in 2, 5 and 6 positions of guaiacyl units.
The studies of Hatakeyama et al. [Citation31] and Hatakeyama [Citation32] have shown that glass transition temperature (Tg) of lignin depends on various parameters, viz. method used for its extraction, thermal history, cross-linking nature, origin of sample, molecular weight and presence of low-molecular-weight contaminants. DSC spectra indicated that Tg of DLNP and ALNP are 123 and 121 °C, respectively ((c)). These results were in good agreement with the previous reports on Tg (100–180 °C) of lignin isolated from different sources using various extraction methods.[Citation33,Citation34] Taken together, these results suggest that both DLNP and ALNP retained structural and thermal properties of their parent polymers DL and AL, respectively.[Citation27]
3.3. Antioxidant potential
DPPH is a stable free radical and widely used to test the radical scavenging ability of lignin isolated from different sources as well as lignin model compounds.[Citation35–37] However, the radical scavenging ability of lignin was reliant on its free phenolic groups which tend to form phenoxyl radicals during scavenging process. Thus, the radical scavenging ability of lignin was delegated by its stable phenoxyl radical. Further, phenolic structures with ortho-substituents, such as methoxyl units, also stabilise these phenoxyl radicals by hindering them from propagation as well as by resonance.[Citation36,Citation37]
The results from suggest that both DL and AL possessed antioxidant potential. The IC50 of DL was higher (32 µg) when compared with standard food antioxidants BHT and Asc (≤10.0 µg) as well as AL (11 µg). The lower the IC50 value, the higher is its antioxidant potential. However, the IC50 of DL corroborated well with that of pyrolytic lignins (39–75 µg) and is in the acceptable range of several other potent antioxidants.[Citation38] The high DPPH scavenging ability of AL when compared with DL might be possible due to the presence of high guaiacyl content in softwood lignin. Brand-Williams et al. [Citation37] reported that guaiacol groups which are pyrolytic derivatives of guaiacyl lignin possessed more antioxidant ability consistent with the presence of ortho-methoxy substitution. Ugartando et al. [Citation11] demonstrated that lignin isolated from different sources inhibited 2,2'-azobis(2-amidinopropane) dihydrochloride (AAPH)-induced red blood haemolysis under in vitro conditions. The inhibition of haemolysis of blood was enhanced with increasing concentrations of lignin. Further, they also observed that the antioxidant capacity of lignin was decreased in the presence of carbohydrates, since their polar groups tend to form hydrogen bonds with phenolic groups of lignin. In the present study, the test compounds, viz. DL, AL, DLNP and ALNP, showed hyperbolic response while scavenging DPPH radicals with saturation at ≤150 µg. However, AL showed slightly higher antioxidant potential over DL, perhaps possibly due to low carbohydrate contamination in AL when compared with DL.[Citation27]
Figure 4. Antioxidant activity of DL, DLNP, AL, ALNP, BHT and Asc. The absorbance of DPPH was 0.9 ± 0.1 in the absence of test compounds. Results reported are mean ± SE of triplicates performed on three different days. Further details are as described in materials and methods.
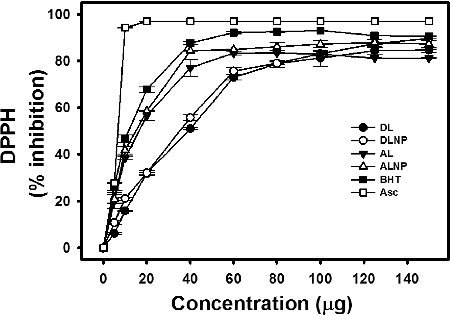
The radical scavenging ability of DLNP and ALNP was higher when compared with their parent polymers DL and AL, respectively, and showed similar trend in scavenging DPPH upon increasing their concentrations (). The IC50 of DLNP (30 µg) was marginally lower than DL (32 µg), while the IC50 of ALNP (14 µg) was marginally higher than AL (11 µg). However, in all the test concentrations that are examined, the per cent scavenging ability of DLNP and ALNP was found to be marginally high when compared with their parent polymers DL and AL, respectively. At 150 µg concentration, the test compounds showed radical scavenging ability in the following order: DLNP > ALNP > DL > AL.
3.4. UV protectant activity
Salmouny et al. [Citation13] demonstrated the vital role of lignin in protecting Spodoptera exigua embedded nucleopolyhedrovirus (SeMNPV) which is known to break down on exposure to UVA (320–400 nm) and UVB (280–320 nm) radiation. Generally, UV radiation causes intracellular oxidative damage in E. coli thereby causing their mortality. Since lignin has characteristic UV-absorbing capacity at 281 nm as evident in (a), the potential of DLNP and ALNP in protecting against UV was evaluated by monitoring the survival rates of E. coli with reference to their parent polymers DL and AL. In the absence of test compounds, E. coli showed 100% mortality when irradiated with UV light for 5 min. However, when E. coli were irradiated with UV in the presence of test compounds, their survival rate was enhanced with increase in the concentration of test compounds in a dose-dependent manner ().
Figure 5. Survival rate of E. coli: The cells were incubated with dioxane lignin (DL), alkali lignin (AL), dioxane lignin nanoparticles (DLNP) and alkali lignin nanoparticles (ALNP) at different concentrations and irradiated with UV light for 5 min. Control – no test compounds and no UV irradiation; *– in the absence of test compounds growth of E. coli was not observed after UV irradiation at 10−5 dilution. The results shown here are representative of three to four independent experiments performed on different days. Further details are as described in materials and methods.
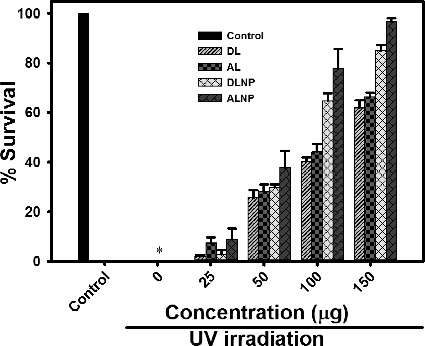
Earlier studies of Zimniewska et al. [Citation39] indicated that nanostructured lignin enhanced the UV barrier properties of hemp fabric in a dose-dependent manner. Similar results were obtained in the present study. At 25 µg concentration of DL or AL, the survival rate of E. coli was ≤10%. However, when the concentration of DL or AL was increased to 150 µg, the survival rate of E. coli was enhanced up to 67%. Similarly, in the presence of DLNP or ALNP, the survival rate of E. coli was ≤10% at 25 µg. However, the survival rate of E. coli was enhanced up to 97% with the increase in concentration of DLNP or ALNP to 150 µg (; Supplementary Figures S1– S3). The results from the present study indicate that the nanoparticles possessed significant UV protectant activity when compared with their parent polymers, and the protection ability is in the following order: ALNP > DLNP > AL > DL. However, at 150 µg concentration, DLNP and ALNP showed 25% and 30% increase in their UV protectant activity when compared with their parent polymers DL and AL, respectively. The higher UV protectant potential of AL/ALNP than DL/DLNP might be due to high guaiacyl content in AL/ALNP, which tends to absorb more UV ((a)).
To evaluate further, the UV protectant potential of DLNP and ALNP, E. coli was exposed to increasing time periods of UV light while keeping the concentration of test compounds constant (150 µg). At this concentration, the survival rates of E. coli decreased in the presence of all test compounds (DLNP, ALNP, DL and AL) with increasing time period of UV irradiation (; Supplementary Figures S4–S6). However, the decrease in survival rate was more pronounced in the presence of DL and AL when compared with their respective nanoparticles DLNP and ALNP. In the presence of ALNP, the survival rate of E. coli which was 97% after 5 min of UV irradiation decreased to ≤60% after 15 min of UV irradiation. On the other hand, in the presence of DLNP, the survival rate of E. coli which was 85% after 5 min of irradiation decreased to ≤70% after 15 min of UV irradiation.
Figure 6. Survival rate of E. coli after UV irradiation for different time periods: 5, 10 and 15 min in the presence of 150 µg concentration of each of dioxane lignin (DL), alkali lignin (AL), dioxane lignin nanoparticles (DLNP) and alkali lignin nanoparticles (ALNP). Control – no test compounds and no UV irradiation; *– in the absence of test compounds growth of E. coli was not observed after UV irradiation at 10−5 dilution. The results shown here are representative of three to four independent experiments performed on different days. Further details are as described in materials and methods.
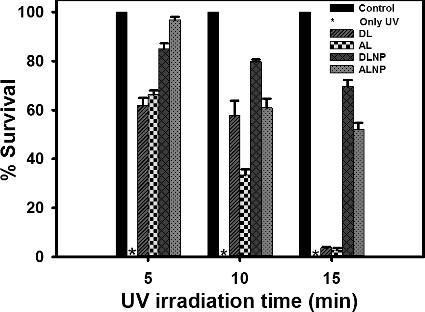
Taken together, the results suggest that both DLNP and ALNP showed improved UV protectant activity when compared with their parent polymers DL and AL. However, the decrease in survival rate of E .coli with increasing irradiation time period might be due to the decrease in UV-absorbing capacity of lignin. Since the phenolic group is responsible for UV absorbance in lignin, any disturbance in phenolic groups is reflected in the UV absorbance of lignin. Machado et al. [Citation40] described that direct photolysis causes reduction in free phenolic group content. As observed in , both DL and DLNP exhibited significant UV protection activity when compared with AL and ALNP upon increasing UV irradiation time. This result can be correlated to high guaiacyl content (more free phenolic groups) in AL/ALNP which might decrease due to direct photolysis upon exposure to UV irradiation for longer duration.
4. Conclusions
In the present study, DLNP and ALNP prepared from DL of subabul stem cuttings and commercial AL were extremely stable at room temperature. While retaining their structure and antioxidant properties, both DLNP and ALNP showed pronounced UV protectant activity when compared with their parent polymers DL and AL. However, high yield and stability of DLNP against UV irradiation for longer duration suggest that lignin nanoparticles prepared from subabul stems can be exploited further to replace chemically synthesised antioxidants and UV protectants in various industrial formulations.
Supplemental data
Supplemental data for this article can be accessed here.
tjen_Preparation_and_characterization_of_lignin_nanoparticles..._Srinivasa_and_Kollipara.docx
Download MS Word (544 KB)Acknowledgements
This work was supported by funds from Nanotechnology project grant (No. IR/S5/IU-01/2006) to K.P.S, University of Hyderabad (UoH) from DST-Nano Mission, Govt. of India. SRY is thankful to UGC, New Delhi, India for providing RGNF (JRF & SRF). The authors thank Prof A.S. Raghavendra and Dr Saradadevi Tetali, School of life Sciences (SLS), UoH, for extending their lab facilities for the implementation of work; Prof S. Dayanada and Mr Sunil Parthasarathy, SLS, UoH, for help in UV irradiation of E. coli; Prof Tushar Jana, School of chemistry, UoH, for the help in analysing data related to DSC studies; Central Instrumentation Laboratory, UoH, for providing DSC; School of Physics, UoH, for FESEM facilities and Center for Nanotechnology (CFN), UoH, for TEM facilities.
Disclosure statement
No potential conflict of interest was reported by the authors.
Additional information
Funding
References
- Afaq F, Mukhtar H. Botanical antioxidants in the prevention of photocarcinogenesis and photoaging. Exp Dermatol. 2006;15:678–684.
- Chanchal D, Swarnlata S. Herbal photoprotective formulations and their evaluation. Open Nat Prod J. 2009;2:71–76.
- Kapur A, Sati S, Ranjan A, et al. Screening methanolic extracts of beta vulgaris roots for photoprotective activity. Int J Pharm Pharm Sci. 2012;4:124–127.
- Svobodová A, Psotová J, Walterová D. Natural phenolics in the prevention of UV-induced skin damage. A review. Biomed Pap. 2003;147:137–145.
- Dong X, Dong M, Lu Y, et al. Antimicrobial and antioxidant activities of lignin from residue of corn stover to ethanol production. Ind Crops Prod. 2011;34:1629–1634.
- García A, Toledano A, Andrés MA, et al. Study of the antioxidant capacity of Miscanthus sinensis lignins. Proc Biochem. 2010;45:935–940.
- Lu FJ, Chu LH, Gau RJ. Free radical-scavenging properties of lignin. Nutrit Cancer. 1998;30:31–38.
- Martignoni ME, Iwai PJ. Laboratory evaluation of new ultraviolet absorbers for protection of Douglas-fir Tussock moth (Lepidoptera: Lymantriidae) baculovirus. J Econ Entomol. 1985;78:982–987.
- Rozema J, Van De Staaij J, Björn LO, et al. UV-B as an environmental factor in plant life: stress and regulation. Trends Ecol Evol. 1997;12:22–28.
- Tamez-Guerra P, McGuire MR, Behle RW, et al. Sunlight persistence and rainfastness of spray-dried formulations of baculovirus isolated from Anagrapha falcifera (Lepidoptera: Noctuidae). J Econ Entomol. 2000;93:210–218.
- Ugartondo V, Mitjans M, Vinardell MP. Comparative antioxidant and cytotoxic effects of lignins from different sources. Bioresour Technol. 2008;99:6683–6687.
- Salamouny SE, Herz A, Huber J. Suitability of three lignin products as UV protectants to baculovirus.. Bull Ent Soc Egypt Econ Ser. 2002;28:103–111.
- Salamouny SE, Shapiro M, Ling KS, et al. Black tea and lignin as ultraviolet protectants for the beet armyworm nucleopolyhedrovirus. J Entomol Sci. 2009;44:50–58.
- Belicová A, Krajčovič J, Križková L, et al. Anti-u.v. activity of lignin biopolymers on Euglena gracilis. World J Microbiol Biotechnol. 2000;16:91–93.
- Qian Y, Qiu X, Zhu S. Lignin: a nature-inspired sun blocker for broad-spectrum sunscreens. Green Chem. 2015;17:320–324.
- Vauthier C, Bouchemal K. Methods for the preparation and manufacture of Polymeric Nanoparticles. Pharm Res. 2009;26:1025–1058.
- Popa VI, Cǎpraru AM, Grama S, et al. Nanoparticles based on modified lignins with biocide properties. Cellulose Chem Technol. 2011;45:221–226.
- Frangville C, Rutkevicius M, Richter AP, et al. Fabrication of environmentally biodegradable lignin nanoparticles. Chemphyschem. 2012;13:4235–4243.
- Lu Q, Zhu M, Zu Y, et al. Comparative antioxidant activity of nanoscale lignin prepared by a supercritical antisolvent (SAS) process with non-nanoscale lignin. Food Chem. 2012;135:63–67.
- Fukushima RS, Hatfield RD. Extraction and isolation of lignin for utilization as a standard to determine lignin concentration using the acetyl bromide spectrophotometric method. J Agric Food Chem. 2001;49:3133–3139.
- Fessi H, Piusieux F, Devissaguet JP, et al. Nanocapsule formation by interfacial polymer deposition following solvent displacement. Int J Pharm. 1989;55:R1–R4.
- Legrand P, Lesieur S, Bochot A, et al. Influence of polymer behaviour in organic solution on the production of polylactide nanoparticles by nanoprecipitation. Int J Pharm. 2007;344:33–43.
- Kumar S, Kumar D, Manjusha SK, et al. Antioxidant and free radical scavenging potential of Citrullus colocynthis (L.) Schrad. methanolic fruit extract. Acta Pharm. 2008;58:215–220.
- Schubert S, Delaney JJT, Schubert US. Nanoprecipitation and nanoformulation of polymers: from history to powerful possibilities beyond poly(lactic acid). Soft Matter. 2011;7:1581–1588.
- Lee J, Hwang S, Lee D, et al. Formation of poly(ethylene glycol)-poly(ϵ-caprolactone) nanoparticles via nanoprecipitation. Macromol Res. 2009;17:72–78.
- Elek N, Hoffman R, Raviv U, et al. Novaluron nanoparticles: formation and potential use in controlling agricultural insect pests. Colloid Surf A. 2010;372:66–72.
- Yearla SR, Padmasree K. Leucaena leucocephala (Lam.) de Wit., ‘subabul’ stem lignin: isolation, structural characterization and thermal properties. Indian J Exp Biol. Forthcoming 2015.
- Lin SY. Ultraviolet spectrophotometry. In: Lin SY, Dence CW, editors. Methods in lignin chemistry. Heidelberg: Springer-Verlag; 1992. p. 215–232.
- Faix O. Fourier transform infrared spectroscopy. In: Lin SY, Dence CW, editors. Methods in lignin chemistry. Heidelberg: Springer-Verlag; 1992. p. 233–241.
- She D, Xu F, Geng Z, et al. Physicochemical characterization of extracted lignin from sweet sorghum stem. Ind Crops Prod. 2010;32:21–28.
- Hatakeyama T, Nakamura K, Hatakeyama H. Studies on heat capacity of cellulose and lignin by differential scanning calorimetry. Polymer. 1982;23:1801–1804.
- Hatakeyama H. Thermal analysis. In: Lin SY, Dence, CW, editors. Methods in lignin chemistry. Heidelberg: Springer-Verlag; 1992. p. 200–214.
- Buranov AU, Ross KA, Mazza G. Isolation and characterization of lignins extracted from flax shives using pressurized aqueous ethanol. Bioresour Technol. 2010;101:7446–7455.
- Tejado A, Peña C, Labidi J, et al. Physico-chemical characterization of lignins from different sources for use in phenol–formaldehyde resin synthesis. Bioresour Technol. 2007;98:1655–1663.
- Dizhbite T, Telysheva G, Jurkjane V, et al. Characterization of the radical scavenging activity of lignins – natural antioxidants. Bioresour Technol. 2004;95:309–317.
- Pan X, Kadla JF, Ehara K, et al. Organosolv ethanol lignin from hybrid poplar as a radical scavenger: relationship between lignin structure, extraction conditions, and antioxidant activity. J Agric Food Chem. 2006;54:5806–5813.
- Brand-Williams W, Cuvelier ME, Berset C. Use of a free radical method to evaluate antioxidant activity. LWT Food Sci Technol. 1995;28:25–30.
- Nsimba RY, West N, Boateng AA. Structure and radical scavenging activity relationships of pyrolytic lignins. J Agric Food Chem. 2012;60:12525–12530.
- Zimniewska M, Kozłowski R, Batog J. Nanolignin modified linen fabric as a multifunctional product. Mol Cryst Liq Cryst. 2008;484:43/[409]–50/[416].
- Machado AEH, Furuyama AM, Falone SZ, et al. Photocatalytic degradation of lignin and lignin models, using titanium dioxide: the role of the hydroxyl radical. Chemosphere. 2000;40:115–124.