Abstract
Tea tree oil (TTO) exhibits excellent broad-spectrum antimicrobial activity. In order to preserve it from the degradation in the presence of oxygen, light and temperature, TTO was encapsulated in liposomes (LTTOs) using the thin-membrane hydration and sonication method, and characterised by Zetasizer for size and size distribution, transmission electron microscope for morphology, zeta-potential for surface charge, entrapment efficiency and TTO release from the nanoparticles. The antimicrobial activities of phosphate-buffered saline solution containing TTO, unloaded liposomes and LTTOs suspension were determined by twofold serial broth dilution technique. The size of LTTOs was 75 nm and the encapsulation efficiency of 96.08% was obtained. LTTOs exhibited slow release of TTO and superior broad-spectrum antimicrobial effects compared with free TTO. Liposomes not only effectively encapsulated TTO to form a stable liposome suspension, but also enhanced inhibition and bactericidal effect on the TTO-tolerant strain. The liposomal systems carrying TTO may be a potential alternative for effective antimicrobial agents.
1. Introduction
Tea tree oil (TTO), the essential oil of Melaleuca alternifolia exhibits broad-spectrum antibacterial, antifungal, antiviral and antiprotozoal activity.[Citation1] Notably, the activity of TTO against antibiotic-resistant bacteria is similar in comparison with antibiotic-sensitive organisms. In addition, a range of yeasts, dermatophytes and other filamentous fungi are susceptible to TTO. Moreover, TTO vapours are capable of inhibiting bacterial and fungal growth as well as affecting sporulation.[Citation1] TTO has gained a reputation as a safe, natural and effective antiseptic in a certain concentration range which has led to its successful use in biomedical applications, such as many pharmaceutical and cosmetic products for external use.[Citation2,Citation3]
Recently, the misuse of conventional antibiotics has resulted in the emergence of many multidrug-resistant strains.[Citation4,Citation5] The resistance of the bacteria and fungi to the innumerous antimicrobial agents constitutes one of the great challenges in the treatment of infections. The use of essential oil, for example, TTO, as antimicrobial agents can overcome problems caused by the misuse of conventional antibiotics.[Citation6,Citation7] Terpinen-4-ol, the main component of TTO is responsible for most of the antimicrobial activity.[Citation8] Since the ingredient of TTO compromises the cytoplasmic membrane and it induces a loss of cellular electron dense material and coagulation of cytoplasmic constituents,[Citation9] stimulates leakage of cellular potassium ions and inhibits microbial cell respiration,[Citation10] TTO represents the in vitro antimicrobial activity against a variety of antibiotic-resistant microorganisms.[Citation1]
The antimicrobial effects of TTO depend on its effective concentrations.[Citation11] Effective concentrations of TTO following dermal topical administration are difficult to control and maintain because of TTO's poor water-solubility and instability, as it is volatile.[Citation1] It is well known that essential oils are sensitive to oxygen, light and temperature,[Citation12] and TTO is no exception. Peroxides, epoxides and endoperoxides were formed as strong sensitisers during preservation from a few days to several months, such as ascaridol, isoascaridol and 1,2,4-trihydroxy menthane. Ascaridol and isoascaridol have never been found before in fresh TTO.[Citation13,Citation14] Additionally, the barrier function of the skin and stratum corneum counteracts the drug penetration and absorption.[Citation15] To circumvent these issues, different formulations encapsulating TTO such as liposomes enhance the solubility of TTO and offer chemical and biological protection.[Citation16] Liposomes, artificially prepared vesicles composed of a lipid bilayer as cell membrane, can be used as a vehicle for administration of nutrients and pharmaceutical drugs. Liposomes encapsulate hydrophilic molecules in the aqueous internal space or lipophilic and amphiphilic molecules that become embedded in their concentric bilayers.[Citation17] Thus, it is speculated that TTO can be dissolved or finely enriched within the shell of liposomes, facilitating contact to the outermost skin layers. The evaporation of water from the lipid nanodispersion applied to the skin surface would lead to a transition of the lipid matrix and a lipid adhesive layer covering the skin surface owing to an additional occlusive effect, facilitating dermal absorption and drug expulsion. Moreover, the hydration of liposomes can temporarily open the compact structure of the horny layer, and the permeability of the barrier increases.[Citation18–20]
In a previous study, it was reported that TTO-based formulations, including colloidal bed, microemulsion, multiple emulsion and liposomal dispersion containing 5% TTO, seemed to deliver TTO to the follicles and reach the effective bactericidal concentration, which should benefit the patients with acne vulgaris.[Citation21] The liposomal formulation was found to have only 60.2% ± 1.2% encapsulation efficiency and the particle size of the liposomes was 320 ± 24.3 nm. TTO-loaded liposomal suspensions were prepared by reverse-phase evaporation vesicles method and their antimicrobial activities were evaluated.[Citation22,Citation23] Recently, the feasibility and antimicrobial efficacy of encapsulated TTO and Ag+ in combination has been investigated.[Citation23,Citation24] Encapsulating silver ions and TTO in a controlled release liposomal carrier system can improve their antimicrobial efficacy as well as reduce the effective concentration required.[Citation23] Meanwhile, silver nanoparticles encapsulated in nanoliposomes have been developed as effective antimicrobial agents.[Citation25] However, potential health risks of nano and ionic forms of silver might exist and need to be weighed in the future due to their cytotoxicity.[Citation26,Citation27] The distribution of TTO components in nanoliposomes is unclear owing to the diverse chemical and physical properties of TTO components.[Citation1] It is highly likely to affect the antimicrobial efficacy of nanoliposome-encapsulated TTO.
In this study, TTO was encapsulated into liposomes using the thin film hydration and sonication method. Sustained release of TTO from liposomes was assessed and the distribution of TTO components in nanoliposomes was analysed. The antimicrobial effects of the prepared liposomes loaded with TTO (LTTOs) on the growth of Gram-positive and Gram-negative bacteria (Staphylococcus aureus and Eschericia coli) and fungi (Candida albicans) were determined.
2. Methods
2.1. Materials
Soybean phosphatidylcholine (PC), cholesterol (Cho) and Tween 80 were purchased from Sinopharm Chemical Reagent Co., Ltd. (Shanghai, China). TTO was obtained from Nanning Innovative Pharmceutical Technology Co., Ltd. (Nanning, China). It is taken from the leaves of the Melaleuca alternifolia by steam distillation, which is native to Australia. Concentrations of the TTO components determined by gas chromatographic analysis (and the range specified by the international standard ISO 4730:1996, shown in parentheses) were as follows: 2.75% α-pinene (1%–6%); 0.53% sabinene (trace to 3.5%); 9.79% α-terpinene (5%–13%); 1.05% limonene (0.5%–4%); 2.19% p-cymene (0.5%–12%); 2.9% 1,8-cineole (0%–15%); 18.91% γ-terpinene (10%–28%); 3.11% terpinolene (1.5%–5%); 46.96% terpinen-4-ol (>30%); 2.56% α-terpineol (1.5%–8%); 0.6% aromadendrene (trace to 7%); 0.44% δ-cadinene (trace to 8%); 0.26% globulol (trace to 3.5%) and 0.29% viridiflorol (trace to 1.5%). Other agents were of analytical grade from Sinopharm Chemical Reagent Co., Ltd.
2.2. Nanoliposomes and LTTOs preparation
The unloaded liposomes (ULs) were prepared using the thin-membrane hydration method with bath-type sonicator.[Citation28] Briefly, the lipid components, 100 mg PC and 18 mg Cho were dissolved in 10 ml chloroform. The solvents were removed using a rotary evaporator at room temperature, 15 rpm, and a high vacuum, which resulted in a uniform, thin lipid membrane on the vessel wall. Traces of organic solvents were removed by storage for 12 hours in a vacuum desiccator. The deposited lipid membrane was hydrated with 10 ml of 12.0 mmol/l phosphate-buffered saline (PBS, containing 0.8% v/v Tween 80), pH 7.4, by agitation for 15 minutes at 55 °C. Simultaneously, sonication of the preparation was carried out in a bath-type sonicator with 300 W of sonication power (Branson Ultrasonics, Danbury, CT, USA) to form small unilamellar vesicles. Finally, the liposomal dispersions prepared were stored at room temperature for 3 hours in order to anneal any structural defects.
For the synthesis of LTTOs, the thin membranes (prepared as previously described) were rehydrated with 10 ml 12.0 mmol/l PBS solution, pH 7.4, containing 0.8% v/v Tween 80 and 1.2% v/v TTO. The mixture was vortexed and sonicated at 55 °C, and then incubated at room temperature for 3 hours. The LTTOs suspension was placed in cellulose dialysis bags. Free TTO was removed by washing with PBS containing 0.8% v/v Tween 80, pH 7.4. Total volume of the washed LTTOs suspension was then made up to 10 ml.
2.3. Characterisation of ULs and LTTOs
2.3.1. Vesicle diameter, size distribution and zeta-potential analysis
Liposome preparation was appropriately diluted with 12.0 mmol/l PBS solution, pH 7.4. Mean particle diameter, polydispersity index and zeta-potential of ULs and LTTOs were directly measured using Zetasizer Nano ZS90 equipment (Malvern Instruments Ltd., Worcestershire, UK). Six independent measurements were performed in each case.
2.3.2. Determination of terpinen-4-ol and 1,8-cineole
Terpinen-4-ol and 1,8-cineole in the solution was determined by gas chromatography with n-dodecane as internal standard. Gas chromatography appliances used included a Shimadzu GC-2014C System equipped with a fused-silica Stabilwax® column (60 m × 0.32 mm i.d.; film thickness 0.50 μm) and a flame ionization detector (FID) detector. Carrier gas was hydrogen at a constant voltage. The oven temperature programme was 75 °C for 4 min, then 4 °C/min until 200 °C was reached, and maintained 10 min. The detector and the injector temperature was 250 °C. The volume of the injected sample was 0.2 μl and the split ratio was 1:50.
2.3.3. Determination of liposome entrapment efficiency
Percentage entrapment efficiency (% EE) was determined by using the petroleum ether extraction method as described by Jacobsohn et al. [Citation29] and Nakamura et al.[Citation30] Briefly, the unwashed liposome suspension was extracted three times with 3.0 ml of petroleum ether each time. For each extraction, the organic layers were collected into a volumetric flask. The mixture was vortexed and terpinen-4-ol in the solution was determined by gas chromatography. Finally, percentage entrapment efficiency of LTTOs was calculated with the following formulae.[Citation31](1)
2.3.4. Transmission electron microscopy
The size and morphology of the synthesised nanostructures were studied using a transmission electron microscope (TEM; JEM-2100, Tokyo, Japan). Briefly, the ULs suspension and LTTOs suspension were diluted 10-fold in 12.0 mmol/l PBS solution, and the sample was deposited on a sample grid (carbon membrane supported by a copper grid) and negatively stained with phosphotungstic acid solution 2% (w/v). All preparations were allowed to dry for 2 hours and then observed with JEM 2100 TEM operating at an accelerating voltage of 100 kV. The particle size was reported as the mean diameter of randomly selected structures.
2.3.5. In vitro release of terpinen-4-ol from LTTOs
In vitro release of terpinen-4-ol from LTTOs was determined by the dialysis method.[Citation25] 10 ml LTTOs suspension was placed in cellulose dialysis bags. The bags were suspended in 30 ml PBS containing 0.8% v/v Tween 80, pH 7.4, where terpinen-4-ol was released into the buffer by diffusion. 10 μl of buffer was removed to measure the concentration of released terpinen-4-ol using gas chromatography every 2 hours. The release of terpinen-4-ol from the dialysis bags was observed over a period of 24 hours.
2.3.6. Determination of antimicrobial effects of LTTOs
Three strains, Gram-positive bacteria (Staphylococcus aureus ATCC 6538), Gram-negative bacteria (Escherichia coli ATCC 8739) and fungi (Candida albicans ATCC 10231) were chosen for antimicrobial test. Bacterial and fungal cells were cultured for 24 hours on nutrient agar and potato dextrose agar plates at 37 °C, respectively. Colonies were resuspended in nutrient broth (bacteria) and yeast extract-peptone-dextrose medium (fungi) to achieve 5.0 × 105 cfu/ml. PBS solution (containing 0.8% v/v Tween 80 and 1.2% v/v TTO), the prepared ULs suspension and LTTOs suspension were added to the bacterial and fungal cultures by the twofold serial broth dilution method. Each organism was tested at least twice, and the culture with the PBS solution without TTO was included in each assay as an internal control. Trays (96 well) were incubated aerobically at 37 °C for 24 hours. And then, 10 μl of broth was removed from each well and the number of surviving organisms was determined by the standard pour plate method with nutrient agar (G+ and G− bacteria) and potato dextrose agar (fungi).
The inhibitory rate and killing logarithm value (KLV) against bacteria and fungi were calculated as follows:(2)
(3) where N0 is the colony number of bacteria (or fungi) in the internal control and Nt is the colony number of bacteria (or fungi) in experiment groups.
2.3.7. Data and statistical analyses
The experimental results were expressed as the mean of mutiple trials ± SD (standard deviation).
3. Results and discussion
3.1. Characterisation of nanoliposomes-encapsulated TTO
The particle size, polydispersity and zeta-potential of liposomes are summarised in (A). The size of LTTOs (75 ± 3 nm) was smaller than that of ULs (142 ± 3 nm), which was consistent with the previous report [Citation32–35] that essential oils could decrease the size of liposomes by causing higher cohesion packing among the apolar chains in the membrane vesicles [Citation33] and forcing soybean phosphatidylcholine vesicles to increase its surface curvature.[Citation36] The polydispersity index (PDI) of LTTOs was around 0.123 and considered satisfactory, indicating a narrow size distribution of LTTOs. However, the PDI of ULs was about 0.521 and unsatisfied.
Figure 1. Characterisation of ULs and LTTOs. (A) Particle size, polydispersity, zeta-potential and encapsulation efficiency of liposomes. The encapsulation efficiency of LTTOs was determined with terpinen-4-ol as the index. (B) Changes in the particle size, polydispersity and zeta-potential of liposomes during 14 weeks storage at 4 °C.Note: Hollow symbols represent ULs and solid symbols are TTO-loaded liposomes. Particle size (square symbols); polydispersity (circular symbols); zeta-potential (triangular symbols).
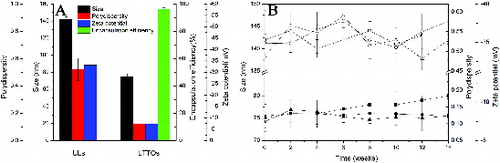
TTO is composed of terpene hydrocarbons, mainly monoterpenes, sesquiterpenes, as well as their associated alcohols and the octanol-water partition coefficients of these components are between 300 and 30,000.[Citation1] Therefore, TTO was hydrophobic and extremely unstable in PBS solution without surfactant, easy to layer, causing the dissolution of liposome membrane in the TTO layer and the failure formation of LTTOs. Lipophilic ingredients were successfully dissolved in lipid bilayer of liposomes after adding Tween 80. As a surfactant, Tween 80 was capable of forming O/W micellesFootnote and TTO was wrapped in the hydrophobic environment so as to improve the solubilisation of TTO. Moreover, the hydrophobic heads of Tween 80 were inserted into the membrane and their hydrophilic groups were extended into the aqueous phase so that Tween 80 could be embedded in the lipid bilayer. Thus, O/W micelles delivered TTO from the oil–water interface to lipid bilayer to prepare LTTOs.
Terpinen-4-ol is the main active antibacterial ingredient of TTO, so the encapsulation efficiency of LTTOs is calculated with the amount of terpinen-4-ol as an indicator. As shown in (A), the encapsulation efficiency of 96.08% was obtained for LTTOs. From the sonication method presented by Biju et al.,[Citation21] the size of the vesicles was reduced to obtain the liposomal dispersion containing 5% TTO. The particle size of the liposomes was 320 ± 24.3 nm with the encapsulation efficiency of 60.2% ± 1.2%. Flores et al. [Citation37] observed that TTO was encapsulated in nanocapsules and nanoemulsions by spontaneous emulsification and interfacial deposition of the preformed polymer methods, respectively. These nanoemulsions and polymeric nanocapsules containing TTO showed nanometric mean size (160–220 nm). The sizes of nanoparticles were so large and encapsulation efficiencies were so low that they were limited in biomedical application in terms of poor sustained antimicrobial properties. Compared with the particle size and encapsulation efficiency, the LTTOs we prepared had the smaller particle size and higher encapsulation efficiency, suggesting a better method to be used for TTO incorporation in liposomes. LTTOs have a higher specific surface area and more TTO loading capacity than those in the previous study. This will lead to favourable stability and antimicrobial activity.[Citation38]
The zeta-potential measurement of LTTOs in PBS solution was –7.45 mV and the zeta-potential of ULs was –33.14 mV. The difference of zeta-potential apparently attributed to that TTO inserted into the lipid membrane. Because the LTTOs stability and the distribution of phospholipids inside and outside of the lipid membrane in the formation process of lipid vesicle depended on several factors: the size of polar head group of phospholipids, the charge of phospholipids, TTO accumulation in the membrane.[Citation39] TTO components were weakly polar and uncharged. Therefore, the absolute value of zeta-potential of LTTOs was reduced and it was more unstable.
The size of LTTOs displayed a slow rise with time of the observation period as shown in (B). The value for the polydispersity and zeta-potential did not significantly change during the period. It was mainly related to the lower absolute value of LTTOs zeta-potential. When the absolute value of zeta-potential value is more than 30, the liposome suspension is stable; that of zeta-potential value is less than 10, the gravitational potential energy caused by Van der Waals forces among liposomes is greater than the repulsive potential energy due to electrostatic repulsion, making liposomes rapidly gather and precipitate, the system is difficult to maintain its physical stability. The lipid bilayer of two or more smaller liposomes was fused to form larger liposomes,[Citation40] leading to either change in the morphology of the membrane or rupture of the liposome membrane, resulting in the release of the liposome-encapsulated components.[Citation41,Citation42] But LTTOs did not rapidly gather, mix and precipitate because Tween 80 as the non-ionic surfactant had steric hindrance in the surface of LTTOs and formed a stable liposomal dispersion.[Citation43] The zeta-potential, polydispersity and particle size measurements carried out during 14 weeks of storage indicated that LTTOs maintained their negative charge and dispersity. Therefore, Tween 80 could enhance the entrapment efficiency and the stability of liposomes.[Citation44] This method stabilised the liposome membrane with the addition of Tween 80 to hydration medium. Furthermore, the electrostatic repulsion of surface charge of ULs was superior to that of LTTOs so as to constitute more stable dispersion. Thus, the changes in the particle size, polydispersity and zeta-potential of ULs in liposomal suspension retained in a certain boumd.
As seen in , TTO liposome suspension was stored in a well-closed container at room temperature in natural light for two weeks, the content of terpinen-4-ol and 1,8-cineole had no obvious change. But terpinen-4-ol content significantly decreased in PBS containing the same TTO concentration under the same storage conditions, while 1,8-cineole content sharply increased. This showed that liposomal encapsulating can prevent the oxidation and deterioration of terpinen-4-ol. 1,8-cineole could be a result of the oxidation of terpinen-4-ol, since 1,8-cineole levels were usually inversely proportional to the levels of terpinen-4-ol.[Citation1] TTO can degrade into strong sensitisers in the presence of oxygen, light and temperature. For example, α-terpinene, a main ingredient of TTO was found to degrade into p-cymene, ascaridol, isoascaridol and colourless crystals that likely were 1,2,4-trihydroxy menthane. These compounds could lead to skin irritation.[Citation13,Citation14] Therefore, liposomes can protect TTO from oxygen, light and temperature.
3.2. TEM analysis
ULs and LTTOs were observed by transmission electron microscopy () and LTTOs had a spheroid shape with a homogeneous size ((D)). The particle diameter of LTTOs was about 75 nm ((E)) and that of ULs was around 145 nm ((B)), which were consistent with those measured by Zetasizer Nano ZS90 equipment. Moreover, many small particles about 10 nm diameter dispersed or aggregated to form the dark areas in the surface of LTTOs. It indicated that non-uniform distribution of compositions proved the existence of TTO components in lipid bilayer membrane of liposomes (). But there were no small particles and the obvious dark areas in the surface of ULs. The boundary of ULs formed thus is continuous and smooth ((C)). The results displayed that TTO ingredients like other lipophilic molecules, such as 4-nerolidylcatechol,[Citation45] were dissolved and inserted in the liposome membrane to occupy the position of phospholipids, causing a decrease in the surface charge density of liposomes. Therefore, zeta-potential of LTTOs was lower than ULs.
Figure 4. Liposome representation of hydrophobic regions in the membrane bilayer where these clusters of TTO ingredients can be inserted: terpinen-4-ol, γ-terpinene, α-terpinene, etc. Clusters dimensions and possible locations are drawn in the relation to the bilayer dimension according to the transmission electron microscopy analysis.
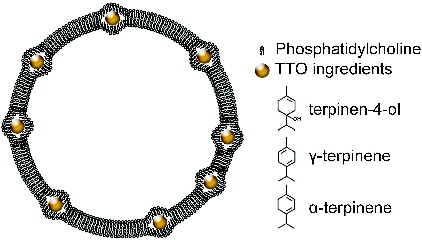
3.3. Release of terpinen-4-ol from liposomes
shows the release of terpinen-4-ol from LTTOs and PBS containing TTO. Terpinen-4-ol in hydration medium quickly diffused and completely spread after about 2 hours to reach the internal and external balance of the cellulose membrane. However, the liposome-encapsulated terpinen-4-ol released slowly and penetrated outside of the cellulose membrane. Terpinen-4-ol continuously released as nearly constant rate (about 1.0%/week) from the liposomes prepared. Therefore, LTTOs retained their functionality during the period, which was in accord with the storage stability of liposome. Owing to their high encapsulation efficiency, stability and slow release of TTO, LTTOs were tested for their antimicrobial effects.
3.4. Antimicrobial effects
The antimicrobial activity of LTTOs was assayed against three common microbial species, namely S. aureus, E. coli and C. albicans (). Minimal inhibitory concentrations of LTTOs against S. aureus, E. coli and C. albicans were 0.15%–0.3%, 0.15%–0.3% and 0.02%–0.04% of TTO terminal concentration, which was better than the antimicrobial activity of TTO formulations against the Gram-negative bacteria.[Citation38] Vuuren et al. prepared TTO-loaded liposomal suspension with the self-made oil by a reverse-phase evaporation method and the minimum inhibitory concentration reached 17–34 μg/ml.[Citation22] Minimal lethal concentrations of LTTOs (KLV ≧ 3.00) against S. aureus, E. coli and C. albicans were 0.15%–0.3%, 0.075%–0.15% and 0.005%–0.01% of TTO terminal concentration. Compared with TTO: polyvinyl alcohol (PVA30—70 kDa)-loaded liposomes,[Citation23] the value of prepared LTTOs against the Gram-positive bacteria was similar, but smaller than that against the Gram-negative bacteria and fungi. The inhibitory and lethal effects of PBS containing TTO and LTTOs suspension against fungi surpassed those against bacteria. Moreover, the antimicrobial effect of PBS containing TTO on the Gram-positive bacteria was better than that of the Gram-negative bacteria. This characteristic was in agreement with the feature of inhibiting and killing microorganisms of TTO. The antimicrobial efficacy of essential oil against the Gram-positive bacteria was superior to the Gram-negative bacteria.[Citation46] LTTOs liposomal system showed yet superior bactericidal effect against the Gram-positive bacteria than that against the Gram-negative bacteria. In addition, ULs suspension had no inhibition and killing effects for fungi and bacteria. The results indicated that TTO was the main antibacterial and antiseptic component.
Figure 6. Broad-spectrum antimicrobial and bactericidal effects of LTTOs suspension. Antimicrobial and bactericidal effects of PBS solution containing TTO, ULs and LTTOs against (A) S. aureus, (B) E. coli and (C) C. albicans.Note: IR: inhibitory rate; KLV: killing logarithm value; ULs: unloaded liposomes; LTTOs: TTO-loaded liposomes.
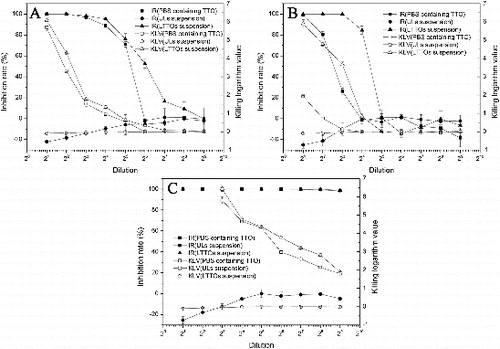
TTO and its components acted on the microbial cell membranes to form perforation,[Citation9] disrupted the distribution of normal lipid bilayer structure of the cell membrane [Citation11] and induced hydrolases degradating cell wall to stimulate cell autolysis, acting on the depolarization of normal cell membrane to cause cell death.[Citation1] However, it has proved that autolysis is a secondary event that occurs following TTO-induced cell death.[Citation9] It was speculated that the antimicrobial activity of essential oil was significantly influenced by the composition of the bacterial membrane [Citation47] and the Gram-negative bacteria had a strong resistance to TTO on account of high adventitia potential.[Citation48] In this work, the bacteriostasic and bactericidal effect of LTTOs were significantly improved in contrast with PBS containing TTO, especially for the Gram-negative bacteria. The cells of the Gram-negative bacteria possess two layers of lipid bilayer, cell membrane and specific outer membrane containing lipopolysaccharide and porins, which displays the identification of molecules into the cell, making themselves unsusceptible to PBS containing TTO. Liposomes wrapped TTO in LTTOs and negated the molecular recognition of the outer membrane, improving the lethal efficacy of TTO against the Gram-negative bacteria.
In previous studies, it has been shown that liposomes have the ability to increase the antimicrobial activities of encapsulated essential oils. Liposomes loaded with pure compounds, carvacrol and thymol, which were the major components of Origanum dictamnus L. oil, revealed a significant increase in the antimicrobial activity after encapsulation.[Citation47] Encapsulation of TTO into liposomes enhanced the solubility of TTO and overcame the volatility concerns inherent to this oil. Additionally, the structure of the liposome was similar with the protoplast of cells and both of their external layers were the lipid bilayer. PC-liposomes could interact with cells in many ways (inter-membrane transfer, contact release, absorption, fusion, phagocytosis, etc.) and strengthen the cell permeability to TTO. The interaction led to the same antibacterial effect of TTO against multifarious microorganisms which had the lipid membrane structure. Consequently, the dramatically increased antimicrobial efficacy of TTO against microbial cells could reduce the concentration of TTO necessary to achieve complete inhibition of microbial growth after the encapsulation in liposomes.
4. Conclusion
The thin-film hydration and sonication technique can produce TTO-loaded nanoliposomes to achieve high encapsulation efficiency, excellent stability and antimicrobial effects. The electrostatic repulsive force between the surfaces of liposomes and the steric effect of Tween 80 stabilised the non-uniform TTO distribution in the lipid bilayer of LTTOs to provide LTTOs with a greater ability to slow the release behaviour and guaranteed higher TTO loading efficiencies in comparison with the TTO-based formulations in the previous study. Furthermore, liposomes loaded with TTO showed a significant increase in the antimicrobial activity after encapsulation.
Note
Disclosure statement
No potential conflict of interest was reported by the authors.
Additional information
Funding
Notes
1. A micelle is an aggregate of surfactant molecules dispersed in a liquid colloid. A typical micelle in aqueous solution forms an aggregate with the hydrophilic ‘head’ regions in contact with surrounding solvent, sequestering the hydrophobic single-tail regions in the micelle centre. This phase is caused by the packing behavior of single-tail lipids in a bilayer. The difficulty filling all the volume of the interior of a bilayer, while accommodating the area per head group forced on the molecule by the hydration of the lipid head group, leads to the formation of the micelle. This type of micelle is known as a normal-phase micelle (oil-in-water micelle, that is O/W micelle).
References
- Carson CF, Hammer KA, Riley TV. Melaleuca alternifolia (tea tree) oil: a review of antimicrobial and other medicinal properties. Clin Microbiol Rev. 2006;19:50–62.
- Halcon L, Milkus K. Staphylococcus aureus and wounds: a review of tea tree oil as a promising antimicrobial. Am J Infect Control. 2004;32:402–408.
- Kreuger MRO, Ternes CE, Mello LL, et al. The influence of the essential oil of Melaleuca alternifolia on the healing of infected dental alveoli: a histological study in rats. Revista Brasileira de Farmacognosia. 2007;17:349–355.
- Alanis AJ. Resistance to antibiotics: are we in the post-antibiotic era? Arch Med Res. 2005;36:697–705.
- Heymann DL. Resistance to anti-infective drugs and the threat to public health. Cell. 2006;124:671–675.
- Ninomiya K, Maruyama N, Inoue S, et al. The essential oil of Melaleuca alternifolia (tea tree oil) and its main component, terpinen-4-ol protect mice from experimental oral candidiasis. Biol Pharm Bull. 2011;35:861–865.
- Thomsen NA, Hammer KA, Riley TV, et al. Effect of habituation to tea tree (Melaleuca alternifolia) oil on the subsequent susceptibility of Staphylococcus spp. to antimicrobials, triclosan, tea tree oil, terpinen-4-ol and carvacrol. Int J Antimicrob Agents. 2013;41:343–351.
- Mondello F, De Bernardis F, Girolamo A, et al. In vivo activity of terpinen-4-ol, the main bioactive component of Melaleuca alternifolia Cheel (tea tree) oil against azole-susceptible and -resistant human pathogenic Candida species. BMC Infect Dis. 2006;6:158–165.
- Gustafson JE, Liew YC, Chew S, et al. Effects of tea tree oil on Escherichia coli. Lett Appl Microbiol. 1998;26:194–198.
- Cox S, Gustafson J, Mann C, et al. Tea tree oil causes K+ leakage and inhibits respiration in Escherichia coli. Lett Appl Microbiol. 1998;26:355–358.
- Cox SD, Mann CM, Markham JL, et al. The mode of antimicrobial action of the essential oil of Melaleuca alternifolia (tea tree oil). J Appl Microbiol. 2000;88:170–175.
- Martín Á, Varona S, Navarrete A, et al. Encapsulation and co-precipitation processes with supercritical fluids: applications with essential oils. Open Chem Eng J. 2010;4:31–41.
- Hausen BM. Evaluation of the main contact allergens in oxidized tea tree oil. Dermatitis. 2004;15:213–214.
- Hausen BM, Reichling J, Harkenthal M. Degradation products of monoterpenes are the sensitizing agents in tea tree oil. Am J Contact Dermatitis. 1999;10:68–77.
- Gupta M, Agrawal U, Vyas SP. Nanocarrier-based topical drug delivery for the treatment of skin diseases. Expert Opin Drug Del. 2012;9:783–804.
- Sherry M, Charcosset C, Fessi H, et al. Essential oils encapsulated in liposomes: a review. J Liposome Res. 2013;23:268–275.
- Vazquez-Gonzalez ML, Bernad R, Calpena AC, et al. Improving ex vivo skin permeation of non-steroidal anti-inflammatory drugs: Enhancing extemporaneous transformation of liposomes into planar lipid bilayers. Int J Pharm. 2014;461:427–436.
- Shiva G, Somaye M, Reza JM. Improved photostability, reduced skin permeation and irritation of isotretinoin by solid lipid nanoparticles. Acta Pharmaceutica. 2012;62:547–562.
- Kuchler S, Radowski MR, Blaschke T, et al. Nanoparticles for skin penetration enhancement – a comparison of a dendritic core-multishell-nanotransporter and solid lipid nanoparticles. Eur J Pharm Biopharm. 2009;71:243–250.
- Borgia SL, Regehly M, Sivaramakrishnan R, et al. Lipid nanoparticles for skin penetration enhancement-correlation to drug localization within the particle matrix as determined by fluorescence and parelectric spectroscopy. J Control Release. 2005;110:151–163.
- Biju SS, Ahuja A, Khar RK. Tea tree oil concentration in follicular casts after topical delivery: determination by high-performance thin layer chromatography using a perfused bovine udder model. J Pharm Sci. 2005;94:240–245.
- van Vuuren SF, du Toit LC, Parry A, et al. Encapsulation of essential oils within a polymeric liposomal formulation for enhancement of antimicrobial efficacy. Nat Prod Commun. 2010;5:1401–1408.
- Low WL, Martin C, Hill DJ, et al. Antimicrobial efficacy of liposome-encapsulated silver ions and tea tree oil against Pseudomonas aeruginosa, Staphylococcus aureus and Candida albicans. Lett Appl Microbiol. 2013;57:33–39.
- Low WL, Martin C, Hill DJ, et al. Antimicrobial efficacy of silver ions in combination with tea tree oil against Pseudomonas aeruginosa, Staphylococcus aureus and Candida albicans. Int J Antimicrob Agents. 2011;37:162–165.
- Eid KA, Azzazy HM. Sustained broad-spectrum antibacterial effects of nanoliposomes loaded with silver nanoparticles. Nanomedicine. 2014;9:1301–1310.
- Yeo M, Kang M. Effects of nanometer sized silver materials on biological toxicity during zebrafish embryogenesis. Bull Kor Chem Soc. 2008;29:1179.
- Pauksch L, Hartmann S, Rohnke M, et al. Biocompatibility of silver nanoparticles and silver ions in primary human mesenchymal stem cells and osteoblasts. Acta Biomaterialia. 2014;10:439–449.
- da Silva Malheiros P, Micheletto YMS, Silveira NPd, et al. Development and characterization of phosphatidylcholine nanovesicles containing the antimicrobial peptide nisin. Food Res Int. 2010;43:1198–1203.
- Jacobsohn MK, Estahani M, Jacobsohn GM. Correlation of side chain mobility with cholesterol retention by phospholipid vesicles. Lipids. 1986;21:691–696.
- Nakamura CV, Ueda-Nakamura T, Bando E, et al. Antibacterial activity of Ocimum gratissimum L. essential oil. Memorias do Instituto Oswaldo Cruz. 1999;94:675–678.
- Ocak B, Gulumser G, Baloglu E. Microencapsulation of Melaleuca alternifolia (tea tree) oil by using simple coacervation method. J Essential Oil Res. 2011;23:58–65.
- Sinico C, De Logu A, Lai F, Valenti D, et al. Liposomal incorporation of Artemisia arborescens L. essential oil and in vitro antiviral activity. Eur J Pharm Biopharm. 2005;59:161–168.
- Valenti D, De Logu A, Loy G, et al. Liposome-incorporated Santolina insularis essential oil: preparation, characterization and in vitro antiviral activity. J Liposome Res. 2001;11:73–90.
- Detoni CB, Cabral-Albuquerque ECM, Hohlemweger SVA, et al. Essential oil from Zanthoxylum tingoassuiba loaded into multilamellar liposomes useful as antimicrobial agents. J Microencapsulation. 2009;26:684–691.
- Detoni CB, de Oliveira DM, Santo IE, et al. Evaluation of thermal-oxidative stability and antiglioma activity of Zanthoxylum tingoassuiba essential oil entrapped into multi- and unilamellar liposomes. J Liposome Res. 2012;22:1–7.
- Turina AdV, Nolan M, Zygadlo J, et al. Natural terpenes: self-assembly and membrane partitioning. Biophys Chem. 2006;122:101–113.
- Flores FC, de Lima JA, Ribeiro RF, et al. Antifungal activity of nanocapsule suspensions containing tea tree oil on the growth of Trichophyton rubrum. Mycopathologia. 2013;175:281–286.
- Biju SS, Ahuja A, Khar RK, et al. Formulation and evaluation of an effective pH balanced topical antimicrobial product containing tea tree oil. Pharmazie. 2005;60:208–211.
- Israelachvili JN. Theoretical considerations on the asymmetric distribution of charged phospholipid molecules on the inner and outer layers of curved bilayer membranes. BBA-Biomembranes. 1973;323:659–663.
- Ma Y, Wang Z, Zhao W, et al. Enhanced bactericidal potency of nanoliposomes by modification of the fusion activity between liposomes and bacterium. Int J Nanomed. 2013;8:2351–2360.
- Amstad E, Reimhult E. Nanoparticle actuated hollow drug delivery vehicles. Nanomedicine. 2012;7:145–164.
- Dhingra S, Morita M, Yoda T, et al. Dynamic morphological changes induced by GM1 and protein interactions on the surface of cell-sized liposomes. Materials. 2013;6:2522–2533.
- Joshi O, Chu L, McGuire J, et al. Adsorption and function of recombinant Factor VIII at the air-water interface in the presence of Tween 80. J Pharm Sci. 2009;98:3099–3107.
- Yang T, Cui FD, Choi MK, et al. Enhanced solubility and stability of PEGylated liposomal paclitaxel: in vitro and in vivo evaluation. Int J Pharm. 2007;338:317–326.
- Gaeti MPN, Benfica PL, Mendes LP, et al. Liposomal entrapment of 4-nerolidylcatechol: impact on phospholipid dynamics, drug stability and bioactivity. J Nanosci Nanotechnol. 2015;15:838–847.
- Andrade BFMT, Barbosa LN, da Silva Probst I, et al. Antimicrobial activity of essential oils. J Essential Oil Res. 2014;26:34–40.
- Liolios C, Gortzi O, Lalas S, et al. Liposomal incorporation of carvacrol and thymol isolated from the essential oil of Origanum dictamnus L. and in vitro antimicrobial activity. Food Chem. 2009;112:77–83.
- Longbottom CJ, Carson CF, Hammer KA, et al. Tolerance of Pseudomonas aeruginosa to Melaleuca alternifolia (tea tree) oil is associated with the outer membrane and energy-dependent cellular processes. J Antimicrob Chemother. 2004;54:386–392.