ABSTRACT
Biogenic synthesis of nanoparticles offers an attractive alternate to chemical synthesis methods. Various hazard free, eco-friendly methods of synthesis of silver nanoparticles are in operation. In chemical reduction methods, the reducing agent is a chemical solution, whereas in biological ones, the collection of enzymes, especially nitrate reductase, plays this role. The highest antibacterial activity of silver nanoparticles synthesised by chemical and biological methods was found in Staphylococcus aureus and Escherichia coli. The paper aims to discuss some fundamental issues about non-biological methods and benefits about biological methods for silver nanoparticles synthesis and their antibacterial studies.
1. Introduction
The area of nanotechnology research is emerging as a cutting edge technology interdisciplinary with biology, physics, chemistry, material science and medicine. There are a huge number of physical, chemical, biological and hybrid methods available to synthesise different types of nanoparticles (NPs). Although physical and chemical methods are more popular in the synthesis of nanoparticles, the use of toxic chemicals greatly limits their biomedical applications, in particular, in clinical fields.
The main drawback with the chemical and physical methods of silver nanoparticle formation is that they are extremely costly and also involve the use of toxic, hazardous chemicals and they contain potential environmental and biological stakes.[Citation1] The way in which the silver nanoparticles blended must be taken care of by human and must be accessible at low evaluated rates for their compelling usage; hence, there is a requirement for an ecologically and financially doable approach to incorporate these nanoparticles. The hunt for such a method has led to the requirement for biogenic production of silver nanoparticles, whereby, biological methods are used to synthesise the silver nanoparticles. The developing need to grow ecologically cordial and monetarily doable innovations for material combination prompted the quest for biomimetic systems for synthesis.[Citation2] Research in bionanotechnology has shown to provide reliable, eco-friendly processes for synthesis of noble nanomaterials. Biologically synthesised silver NPs could have numerous applications in regions, for example, non-direct optics, frightfully particular covering for sunlight-based vitality retention and intercalation materials for electrical batteries, as optical receptors, catalysis in compound responses, biolabelling and as antibacterials.[Citation3] Nanotechnology mainly deals with the synthesis and stabilisation of various nanoparticles. Presently, there is a constant need to develop eco-friendly processes for the synthesis of nanoparticles. The focus for this synthesis has shifted from physical and chemical processes towards ‘green’ chemistry and bioprocesses.[Citation4] There are numerous methods for chemical synthesis of silver nanoparticles, but biogenic synthesis of nanoparticles offers a fascinating alternative to chemical synthesis. Silver nanoparticles have been the theme of researchers because of their distinctive properties (e.g. size, shape and antimicrobial properties). Mainly, there are three major sources of synthesising silver nanoparticles: bacteria, fungi and plant extracts. This biosynthesis of silver nanoparticles mostly involves oxidation reduction reactions. In the case of bacteria, the first synthesising silver nanoparticle was established using the strain Pseudomonas stutzeri AG259 strain that was isolated from silver mine.[Citation5] In plants, the real focal point of utilising plant concentrates for silver nanoparticle combination is that they are effortlessly accessible, safe and nontoxic much of the time and that they have an expansive mixed bag of metabolites that can help in the reduction of silver ions. Compared with bacteria, fungi have the capacity to produce larger amounts of nanoparticles because they are regarded as the organisms that produce nanoparticles extracellularly due to extensive secretory components, which is included in the reduction and capping of nanoparticles.[Citation6] Silver nanoparticles have received much attention as antimicrobial agents and have been shown to be an effective antimicrobial agent.[Citation7] The aim of our work is to synthesise silver nanoparticles both by chemical and biological means with well-defined size and to verify their antimicrobial activity on the series of Gram-positive and Gram-negative bacteria.
2. Material and methods
2.1. Chemicals
Silver nitrate, trisodium citrate merck (Germany), malt extract was procured from Himedia (India). Sterile distilled water was used throughout the experiments.
2.2. Bacterial cultures used
Staphylococcus aureus, Bacillus subtilis, Escherichia coli and Pseudomonas aeruginosa were procured from Microbial Type Collection (MTCC) centre, IMTECH, Chandigarh, India.
2.3. Chemical synthesis of silver nanoparticles
The silver nanoparticles were prepared by using chemical reduction method suggested by Fang, Zhang and Mu.[Citation8] All ingredients of reacting materials were prepared in double distilled water. In typical experiment, 50 ml of 1 × 10−3 M AgNO3 was heated to boiling. To this solution, 5 ml of 1% trisodium citrate was included drop by drop. Solution was mixed vigorously during this process and heated until the colour change was noticeable (pale brown). Finally, it was removed from the heating element and stirred until cooled to room temperature ().
2.4. Biological synthesis of silver nanoparticles
The white rot fungi Pycnoporus sp (HE792771) was grown in malt extract broth containing glucose10 g/l, malt extract 5 g/l. The final pH was adjusted to 6.0 and the flasks were incubated in the orbital shaker at 200 rpm at 32 °C. After five days of incubation, the mycelium was separated by filtration and supernatant was challenged with equal amount of silver nitrate solution of 1 mM (prepared in deionised water) and incubated in shaker at 200 rpm in dark condition at 32 °C (). Simultaneously, a positive control of silver nitrate solution and deionised water, and a negative control containing only silver nitrate solution were maintained under same conditions.
2.5. UV–Vis spectroscopy analysis
Ultraviolet–visible (UV–Vis) spectral analysis was done by using ELICO SL-159 Spectrophotometer in the range of 350–470 nm. The reduction of pure silver ions synthesised by chemical and biological means was monitored by measuring the UV–Vis spectrum of the reaction mixture.
2.6. Antibacterial activity
Silver nanoparticles synthesised by chemical and biological means were tested for antimicrobial activity as suggested by Srinivasulu et al. [Citation9] taking Gram-positive and Gram-negative bacteria by the agar well diffusion method. Twenty millilitre of nutrient agar medium was poured into sterilised Petri plates. One day old bacterial culture was taken (1 × 105 CFU/ml) and was used to prepare the bacterial lawns. With the help of a sterilised borer, agar wells of 8 mm diameter were prepared. The wells were injected with 60 μl of biologically synthesised silver nanoparticle solution, 60 μl of chemically synthesised silver nanoparticle solution, 60 μl of 1 mM AgNO3 as a negative control along with 60 μl of 30 μg/ml of streptomycin as a positive control. The plates were further incubated at 37 °C for 24 h and were then examined for the presence of zones of inhibition. The inhibition zone was measured and expressed in millimetre unit.
3. Results and discussion
3.1. Chemical synthesis of silver nanoparticles
Chemical reduction method involves the reduction of AgNO3 in aqueous solution by an effective reducing agent in the presence of appropriate stabiliser, which is necessary in shielding the growth of silver particles through aggregation. During the formation of silver nanoparticles by the chemical reduction method, some of the parameters like the particle size and aggregation state of silver nanoparticles are affected by initial AgNO3 concentration, reducing agent, AgNO3 molar ratios and stabiliser concentrations.[Citation10] So many methods are suggested for the chemical synthesis of silver nanoparticle formation; chemical reduction method,[Citation11] polyol method [Citation12] and radiolytic process [Citation13] have been developed for the synthesis of silver nanoparticles. The best and most easy method of yielding nanoparticles without aggregation, high yield and low preparation cost is chemical reduction method.[Citation14]
3.2. Synthesis of silver nanoparticles by the white rot fungi Pycnoporus sp (HE792771)
In the synthesis of nanoparticles, use of fungi is a relatively recent addition to the list of micro-organisms. In comparison with fungi and bacteria, fungi have the capacity to produce larger amounts of nanoparticles because they can secrete huge amounts of proteins which directly convert to higher productivity of nanoparticles.[Citation15] The production of silver nanoparticles by fungi is said to follow the following steps: trapping of Ag+ ions at the surface of the fungal cells and the subsequent reduction of the silver ions by the enzymes present in the fungal system.[Citation16] Promising synthesis of nanoparticles appears by the use of specific enzymes secreted by fungi. This would lead to the possibility of genetically engineering micro-organisms to overexpress specific reducing molecules and capping agents and, thereby, control the size and shape of the biogenic nanoparticles.[Citation17] The main advantage of the production of nanoparticles extracellularly from fungi is that a large quantity of enzyme which is in pure state and free from cellular protein can be easy to apply for the simple downstream process. Bhainsa et al. [Citation18] has reported the extracellular biosynthesis of Ag particles in the 5–25 nm range using Aspergillus fumigates. Ahmad et al. [Citation19] reported the synthesis of fabrication of extremely stable Ag hydrosol by using Fusarium oxysporum where the particles were stabilised by the proteins excreted through the fungus. Varshney et al. [Citation20] reported synthesis of Ag nanoparticles in the range of 20–80 nm by using a novel fungi Hormoconis resinae. Vigneshwaran et al. [Citation21] reported the biomimetics of Ag nanoparticles by using Phaenerochaete chrysosporium commonly known as white rot fungus. Basavaraja et al. [Citation22] fabricated spherical and stable Ag nanoparticles in the range of 10–60 nm by using Fusarium semitectum.
3.3. UV–Vis spectroscopy analysis
In the case of chemical synthesis method, silver nanoparticles are known to exhibit a characteristic surface plasmon band that can be measured by UV–Vis spectroscopy. shows the plasmon band of silver nanoparticle suspensions, showing a typical absorbance peak for nanoparticles centred at 430 nm.
Figure 2. UV–Vis absorption spectra of silver nanoparticles synthesised by both chemical and biological means.
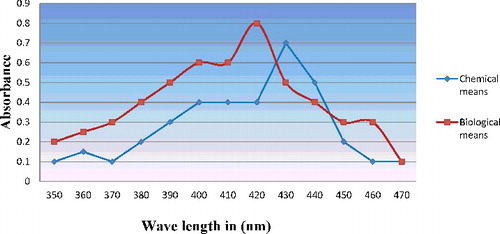
In the case of biological synthesis of silver, nanoparticles production was monitored by the change in colour () and later, it was subjected to optical measurements by UV–Vis spectrophotometer; this analysis showed an absorbance peak at 420 nm (), which was specific for the silver nanoparticles. The exact mechanism of the synthesis of silver nanoparticles was not known, but later it was hypothesised that the fungal biomass contains the nicotinamide adenine dinucleotide hydrogen (NADH) dependent nitrate reductase enzyme, when the silver ions come in contact with the cell wall of the fungal biomass; the nitrate reductase secreted by the fungus causes the reduction of silver ions into silver nanoparticles.
3.4. Antibacterial activity of chemically and biologically synthesised silver nanoparticles
Antimicrobial properties of silver are known from very ancient time. Silver has been generally utilised for curing infections, mending of wound and treating diseases. Moreover, silver nanoparticles were also used for prophylactic treatment of ophthalmia neonatorum in infants, which was, however, restricted due to its toxic effect on eyes causing blindness. Again, recent achievements in the field of technology, especially from the onset of nanotechnology, the antimicrobial effect of silver nanoparticles has regained its lost status and, thus, provides a faster wound healing along with its efficacy against bacterial resistance to antibiotics. Because of the non-harmful, safe inorganic nature, these silver nanoparticles being utilised for quite a long time and is equipped for killing around 650 micro-organisms that cause infections.[Citation23] In this study, silver nanoparticles synthesised by chemical and biological means were tested for antimicrobial activity taking Gram positive and Gram negative bacteria-like S. aureus, B. subtilis, E. coli and Klebsiella pneumonia using agar well-diffusion method (). The diameter of inhibition zones around each well with biologically synthesised Ag NPs, chemically synthesised Ag NPs and AgN03 is recorded in . The highest antibacterial activity of nanoparticles was observed in E.coli and S. aureus followed by B. subtilis and K. pneumonia in biological synthesis. In the case of chemical synthesis, the highest antibacterial activity was observed against S. aureus and E. coli followed by B. subtilis and K. pneumonia. The exact mechanism through which silver nanoparticles adopt to cause antimicrobial effect is not clearly known. Various theories came into existence on the action of silver nanoparticles on micro-organisms to cause microbial effect. Silver nanoparticles have the capacity to hold fast to the bacterial cell wall, and in the long run, enter it; in this manner, it causes structural changes in the cell film like the penetrability of the cell layer and demise of the cell. There is development of pits on the cell surface and there is collection of the nanoparticles on the cell surface.[Citation24] Morones et al. [Citation25] reported the antibacterial action of silver nanoparticles in four sorts of gram negative microbes: E. coli, Vibrio cholerae, P. aeruginosa and Salmonella typhi proposed that silver nanoparticles connect to the surface of the cell film and aggravate its capacity, infiltrate micro-organisms and release silver ions. A similar activity was determined in other groups of antibacterial activity in gram positive bacteria, such as B. subtilis, [Citation26] S. aureus [Citation27] and Enterococcus faecalis.[Citation28]
Table 1. Antibacterial activity of silver nanoparticles synthesised by chemical and biological methods.
4. Conclusion
Chemical and physical methods of silver nanoparticle synthesis were being followed over the decades, but their formation was found to be expensive and the use of various toxic chemicals for their synthesis makes the biological synthesis the more preferred option. In spite, bacterial, fungal and plant extract sources can be used for nanosilver synthesis; this type of formation is very easy, reliable and nontoxic in nature. Different methods are suggested for nanoparticle synthesis; the chemical reduction method and biological synthesis method were widely studied due to its advantage in controlling particle size and morphology very effectively. The reducing agents in chemical reduction method are chemical solutions such as polyol, N2H4, NaBH4, sodium citrate and N,N-dimethyformamide; in the case of biological methods, collection of enzymes, predominantly, nitrate reductase plays such a role. In the case of chemical synthesis methods, a stabiliser (surfactant) is added to the first solution to prevent the agglomeration of silver nanoparticles, whereas in biological synthesis there is no need to add a stabilising agent. Environmental pollution is a disadvantage of the chemical method and the chemical reduction methods are energy-intensive. Biological synthesis methods are carried out in environmental conditions and they are safe enough, and consume no energy. Although the time required to synthesise Ag NPs is longer compared to chemical methods; in fact, the synthesis time has recently reduced with finding suitable organisms. Bio reduction of metal ions by combinations of biomolecules found in the extracts of some of the organisms is environmentally benign, but chemically, it is complex. Here, we report that the silver nanoparticles synthesised from biological means are showing better antimicrobial activity against the pathogenic bacteria comparatively with the chemical synthesis. Especially, we are focusing on fungi because they are the ideal sources in the synthesis of metal nanoparticles because of their capacity to secrete large amount of enzymes. Compared to other micro-organisms, fungal mycelial mesh can withstand flow pressure and agitation, and other conditions in bioreactors or other chambers compared to plant materials and bacteria. This green synthesis approach towards the synthesis of silver nanoparticles has gaining demand in the field of nanotechnology. These nanoparticles with biocompatibility and antimicrobial potency may be exploited for several biomedical applications including development of catheters, topical antimicrobial gel formulations, food packaging ingredients, food processing equipments and so on. In coming generations, silver nanoparticles will be used as a potential tool to combat against rapidly increasing antibiotic resistance.
Disclosure statement
No potential conflict of interest was reported by the authors.
Additional information
Funding
References
- Li X, Xu H, Zhe-Sheng C, et al. Biosynthesis of nanoparticles by microorganisms and their applications. J Nanomaterials. 2011;11:1–16.
- Kalishwaralal K, Deepak V, Ramkumarpandian S, et al. Extracellular biosynthesis of silver nanoparticles by the culture supernatant of Bacillus licheniformis. Mater Lett. 2008;62:4411–4413.
- Cao G, editor. Nanostructures and nanomaterials: synthesis, properties and applications. London: Imperial College Press; 2004.
- Vigneshwaran N, Ashtaputre NM, Varadarajan PV, et al. Biological synthesis of silver nanoparticles using the fungus Aspergillus flavus. Mater Lett. 2007;61:1413–1418.
- Haefeli C, Franklin C, Hardy K. Plasmid-determined silver resistance in Pseudomonas stutzeri isolated from a silver mine. J Bacteriol. 1984;158:389–392.
- Ahmad A, Mukherjee P, Senapati S, et al. Extracellular biosynthesis of silver nanoparticles using the fungus Fusarium oxysporum. Colloids Surf B: Biointerf. 2003;28:313–318.
- Galdiero S, Falanga A, Vitiello M, et al. Silver nanoparticles as potential antiviral agents. Molecules. 2011;16:8894–8918.
- Fang J, Zhang C, Mu R. The study of deposited silver particulate films by simple method for efficient SERS. Chem Phys Lett. 2005;401:271–275.
- Srinivasulu B, Prakasham RS, Jetty A, et al. Neomycin production with free and immobilized cells of Streptomyces marinensis in an airlift reactor. Process Biochem. 2002;38:593–598.
- Song KC, Lee SM, Park TS, et al. Preparation of colloidal silver nanoparticles by chemical reduction method. Korean J Chem Eng. 2009;26:153–155.
- Chou KS, Lu YC, Lee HH. Effect of alkaline ion on the mechanism and kinetics of chemical reduction of silver. Mater Chem Phys. 2005;94:429–433.
- Lin WC, Yang MC. Novel silver/poly (vinyl alcohol) nanocomposites for surface-enhanced Raman scattering-active substrates. Macromol Rapid Commun. 2005;26:1942–1947.
- Shin HS, Yang HJ, Kim SB, et al. Mechanism of growth of colloid silver nanoparticles stabilized by polyvinyl pyrrolidone in γ-irradiated silver nitrate solution. J Colloid Interf Sci. 2004;274:89–94.
- Kim KD, Han DN, Kim HT. Optimization of experimental conditions based on the Taguchi robust design for the formation of nanosized silver nanoparticles by chemical reduction method. Chem Eng J. 2004;104:55–61.
- Mohanpuria P, Rana KN, Yadav SK. Biosynthesis of nanoparticles: technological concepts and future applications. J Nanopart Res. 2008;10:507–517.
- Mukherjee P, Ahmad A, Mandal D, et al. Fungus mediated synthesis of silver nanoparticles and their immobilization in the mycelia matrix: a novel biological approach to nanoparticle synthesis. Nano Lett. 2001;1:515–519.
- Balaji DS, Basavaraja S, Deshpande R, et al. Extracellular biosynthesis of functionalized silver nanoparticles by strains of Cladosporium cladosporioides fungus. Colloids Surf B: Biointerf. 2009;68:88–92.
- Bhainsa KC, D'Souza SF. Extracellular biosynthesis of silver nanoparticles using the fungus Aspergillus fumigates. Colloids Surf B: Biointerf. 2006;47:160–164.
- Ahmad A, Mukherjee P, Mandal D, et al. Extracellular biosynthesis of silver nanoparticles using the fungus Fusarium oxysporum composite metal particles, and the atom to metal. Colloids Surf B: Biointerf. 2003;28:313–318.
- Varshney R, Mishra AN, Bhadauria S, et al. A novel microbial route to synthesize silver nanoparticles using fungus Hormoconis resinae. Dig J Nanomater Bios. 2009;4:349–355.
- Vigneshwaran N, Kathe AA, Varadarajan PV, et al. Biomimetics of Ag nanoparticles by white rot fungus, Phaenerochaete chrysosporium. Colloids Surf B: Biointerf. 2006;53:55–59.
- Basavaraja SS, Balaji SD, Lagashetty AK, et al. Extracellular biosynthesis of silver nanoparticles using the fungus Fusarium semitectum. Mater Res Bull. 2008;43:1164–1170.
- Jeong SH, Yeo SY. The effect of filler particle size on the antibacterial properties of compounded polymer/silver fibers. J Mater Sci. 2005;40:5407–5411.
- Sondi I, Salopek-Sondi B. Silver nanoparticles as antimicrobial agent: a case study on E. coli as a model for gram-negative bacteria. J Colloid Int Sci. 2004;275:177–182.
- Morones JR, Elechiguerra JL, Camacho A, et al. The bactericidal effect of silver nanoparticles. Nanotechnology. 2005;16:2346–2353.
- Yoon KY, Byeon JH, Park JH, et al. Antimicrobial characteristics of silver aerosol nanoparticles against Bacillus subtilis bioaerosols. Environ Eng Sci. 2008;25:289–293.
- Shrivastava S, Bera T, Roy A, et al. Characterization of enhanced antibacterial effects of novel silver nanoparticles. Nanotechnology. 2007;18:103–112.
- Panacek A, Kvitek L, Prucek R, et al. Silver colloid nanoparticles: synthesis, characterization, and their antibacterial activity. J Phys Chem. 2006;110:16248–16253.