ABSTRACT
The present investigation reports the biogenesis of silver nanoparticles (Ag NPs) using extracts of a medicinal plant Nothapodytes foetida. Total phenolic content (TPC) and ferric reducing antioxidant power (FRAP) assay were carried out for the microwave-assisted extract (MAE) of N. foetida using methanol as solvent and the conditions for extraction were optimised by response surface methodology (RSM). The effects of operating variables such as extraction time, temperature and ratio of sample to solvent were studied using central composite design (CCD). A mathematical model with a high determination coefficient (R2) for TPC (0.991) and FRAP (0.995) was obtained. The optimal conditions of extraction for TPC were 48.6 ºC, 23.15 min and 2.04:30 (g/mL) and for FRAP 52.31ºC, 12.32 min and 1.67: 30 (g/mL). Under these conditions, the experimental yields of TPC and FRAP were 2.426 mg gallic acid equivalents (GAE)/g dry powder and 14.985mg of FeSO4·7H2O/g of dry powder, respectively. Ag NPs were characterised using UV–Vis spectroscopy, transmission electron microscopy (TEM), X-ray diffraction (XRD) and Fourier transform infrared (FTIR) spectroscopy. The synthesised Ag NPs have also shown potent activity against the human pathogenic Staphylococcus aureus.
1. Introduction
Biosynthesis of nanoparticles (NPs) has of late been recognised as an efficient and eco-friendly means of synthesis, which is at par with other physicochemical methods [Citation1–3] aiding in the production of nanofabrications with well-defined size and morphology.[Citation4] Although microbes have been identified as potent sources for nanoparticle synthesis, the constraints for the aseptic culturing and maintenance of pure microbial colonies have paved way towards exploring the prospects of plant extracts for the synthesis of nanomaterials.[Citation5,Citation6] Of the various metal nanoparticles explored till date, silver has gained remarkable attention due to its unique optical, electrical and thermal properties that are widely applicable in the field of sensors,[Citation7] bio-labelling [Citation8] and antimicrobials.[Citation9] There are many reports on the synthesis of Ag NPs using extracts of plants such as Ipomoea carnea,[Citation10] Myrica esculenta,[Citation11] Epipremnum aureum,[Citation12] Ficus benghalensis,[Citation13] Cardiospermum halicacabum,[Citation14] Arbutus unedo,[Citation15] Syzygium aromaticum,[Citation15] Nerium oleander [Citation16] and Annona squamosa.[Citation17] The plant extracts were found to be potential sources of biomacromolecules such as phenolic compounds, terpenoids, polysaccharides, flavanoids, H+ ions, NAD+ and ascorbic acid that aid the rapid and efficient reduction of the metals to NPs. For instance, it was reported that the Vitis vinefera (raisin) extract derived sugars (∼60%), phenolics and phytochemicals help in the biosynthesis of Se NPs.[Citation18] Another new dimension to plant-mediated NP synthesis states that the plant cell organelles such as chloroplasts act as excellent biotemplates that provide a reducing and stabilising microenvironment to mediate the synthesis of Au and Ag NPs.[Citation19]
The present study reports the process optimisation for the microwave-assisted extraction of antioxidants of an endangered medicinal plant Nothapodytes foetida for the biosynthesis of Ag NPs. Further, the antibacterial activities of the biosynthesised Ag NPs were also evaluated.
2. Materials and methods
2.1. Microwave-assisted extraction and optimisation of antioxidants by response surface methodology
Microwave-assisted extraction of leaf powder was carried out in a microwave ashing system (Questron Q Ash 800, Canada) using methanol as solvent. The efficiency of extraction was enhanced by optimising the conditions of extraction by response surface methodology. A five-level, three-factor central composite design (CCD) comprising 20 experimental trials was investigated to select the appropriate independent variables such as extraction temperature (X1) ranging from 45 to 55°C, extraction time (X2) ranging from 10 to 20 min and ratio of sample to solvent (X3) from 1 to 2 g/30 mL based on its effect on the yield of antioxidants (dependent variable) in terms of total phenolic content (TPC) and ferric reducing antioxidant power (FRAP), as presented in . The experimental data were fitted to a second-order polynomial model, as given in EquationEquation (1)(1) , where
is the response function,
is the intercept,
are the coefficients of the linear, quadratic and interactive terms, respectively, and
are the levels of independent variables.
(1)
Table 1. Levels and factors of central composite experimental design used in the extraction by MAE.
2.1.1. Statistical analysis for central composite design
The statistical software package Minitab version 14 (Minitab Ltd., Coventry, UK) was used to analyse the experimental data. The optimal values of the critical variables were obtained by analysing surface and contour plots. The statistical analysis of the model was represented in the form of analysis of variance (ANOVA).
2.1.2. Verification of model
Optimal extraction conditions for TPC and FRAP were obtained using the predictive equations generated by response surface methodology (RSM). A verification experiment was done by carrying out the extraction at the optimised conditions in duplicate. The experimental values and model-predicted values were compared to examine the validity of the model.
2.2. Microwave-assisted extraction and determination of antioxidants by total phenolic content and ferric reducing antioxidant power assay
TPC of the extracts was determined by adding 1 mL of Folin–Ciocalteau reagent (10% v/v) to 0.5 mL of sample and incubated for 5 min at room temperature. To the mixture, 4 mL of sodium carbonate (7.5% w/v) was added, covered with aluminium foil, vortexed for 10 s and allowed to stand at room temperature for 30 min in a dark environment.[Citation20] Absorption of the sample was measured at 750 nm using a spectrophotometer against a blank solution. A calibration curve was generated using gallic acid at different concentrations of 0, 0.1, 0.3, 0.5, 0.7, 0.9 and 1.0 mg/mL (R2 = 0.99) and results were expressed in mg gallic acid equivalents (GAE).
Ferric reducing antioxidant power (FRAP) assay was performed as described by Benzie and Strain with minor modifications.[Citation21] A series of stock solutions (200, 400, 800, 1200 and 1600) mM were prepared (R2 = 0.994) using an aqueous solution of FeSO4·7H2O to obtain the calibration curve. 100 μL of the extracted samples were mixed with 3 mL of FRAP reagent (2.5 mL of 20 mM/L 2,4,6-tri (2-pyridyl)-1,3,5-triazine TPTZ solution in 40 mM/L HCl plus 2.5 mL of 25 mM/L FeCl3·6H2O and 25 mL of 0.3 M/L acetate buffer (pH 3.6)) incubated at 30°C for 10 min until the reaction ceased, and the absorbance of samples was measured against blank at 593 nm. The reducing power was determined based on the amount of reduced product of Fe(II) formed from the calibration curve.
2.3. Synthesis and characterisation of silver nanoparticles
NPs were synthesised using the extract of N. foetida obtained at the optimised conditions by adding 1 mL of the extract to 5 mL of 10 mM AgNO3 solution and the bioreduction of Ag+ in the solution was monitored using an UV–Vis spectrophotometer (Shimadzu UV-1800) from 200 to 900 nm. The extracellularly biosynthesised Ag NPs were further characterised by recording the X-ray diffraction (XRD) patterns on a Jeol DX GE-2P vertical goniometer (Japan) operated at a voltage of 30 kV and current of 20 mA with Cu Kα radiation (λ = 1.5405 Å), and the diffracted intensities were recorded from 20° to 80° 2θ angles. Transmission electron microscopic (TEM) analysis was performed using (Jeol 2100, Japan) operating at 200 kV to obtain the micrographs. Fourier transform infrared spectroscopic analysis was carried out in ranges of 400–4000 cm−1 (Perkin Elmer Inc., USA).
2.4. Determination of the antimicrobial activity of silver nanoparticles
The antimicrobial activity of Ag NPs was assessed against human pathogens such as Bacillus subtilis ATCC 6633, Pseudomonas aeruginosa ATCC 27583, Staphylococcus aureus ATCC 25923, Escherichia coli ATCC 25922 and Candida albicans ATCC 69548 using well diffusion assay. Pure cultures of bacteria were grown in Mueller-Hinton broth and Candida in Sabouraud dextrose broth at 30°C and 35°C, respectively, on a rotary shaker at 150 rpm. Each strain was swabbed uniformly onto individual agar plates, 6-mm-diameter wells were made using a cork borer and each well was loaded with different concentrations of Ag NPs (10, 30 and 50 µg/mL). The plates in triplicate were incubated at room temperature. The susceptibility of test human pathogenic microorganisms was determined by measuring the diameter of the zone of inhibition using a vernier caliper.
3. Results and discussion
3.1. Experimental design, model fitting and analysis of response for optimisation of total phenolic content and ferric reducing antioxidant power
Optimisation using RSM has been proven to be a statistically important, rapid and efficient way for the determination of optimum values of process parameters with careful consideration of the sensitivity of the response on each parameter.[Citation22] In the present study, a five-level, three-variable CCD was used as the RSM tool to optimise the extraction of antioxidants. The experimental design used along with the experimental, predicted and residual results of extraction for TPC and FRAP is shown in
Table 2. Central composite experimental design and response for TPC and FRAP.
It can be inferred that, while the maximum absorption value for TPC was recorded under the 11th trial comprising the experimental conditions, for example, extraction temperature of 50°C, extraction time of 23.4 min and ratio of sample to solvent of 1.5:30 (g/mL), the absorption maximum for FRAP was recorded at a temperature of 55°C, extraction time of 10 min and ratio of sample to solvent of 2:30 (g/mL) under the 17th trial. Statistical analysis of the experimental data revealed significant (p < 0.05) linear, square and interaction effects in the variables under consideration for an optimised FRAP. However, in the case of TPC, the linear and square effects of X2 and in-interaction effects of X1 X2 were not found to be significant. These data suggested the prominent influence of factor X2 on TPC extraction.
The statistical analysis of the model by Fisher's statistical test for ANOVA is given in and . The coefficient of determination (R2) was calculated to be 0.991 for TPC and 0.995 for FRAP, implying that 99.1% and 99.5% of the experimental data were compatible with the data predicted by the model for TPC and FRAP extraction, respectively. The values of the adjusted determination coefficient, Adj R2, of 98.3% and 99.1% for TPC and FRAP, respectively, were also high, thus affirming the applicability of the model for an optimised response.
Table 3. Analysis of variance (ANOVA) of a second-order polynomial model for optimisation of TPC.
Table 4. Analysis of variance (ANOVA) of a second-order polynomial model for optimisation of FRAP.
Application of RSM yielded the following second-order polynomial regression equations, EquationEquations (2(2) ) and (Equation3
(3) ), in terms of the coded values:
(2)
(3)
The three-dimensional response surface ((a) and 1(b)) and two-dimensional contour plots generated by the model ((c) and 1(d)) were used to determine the interaction effects of the factors on the response. The convex nature of the response curves confirms an effective optimisation of the process parameters to result in significant optimisation of the TPC and FRAP extraction in a microwave-assisted system. Based on the validation of the model performed at 95% confidence level (), the optimal values were found to be obtained at conditions, for example, 48.6 ºC of extraction temperature, 23.15 min of extraction and ratio of sample to solvent of about 1.82 g sample/30 mL solvent for TPC and 52.31ºC of extraction temperature, 12.32 min of extraction and ratio of sample to solvent of about 1.67 g sample/30 mL solvent for FRAP.
Figure 1. Surface plots showing the optimised conditions for (a) TPC and (b) FRAP and contour plots showing the optimised conditions for (c) TPC and (d) FRAP.
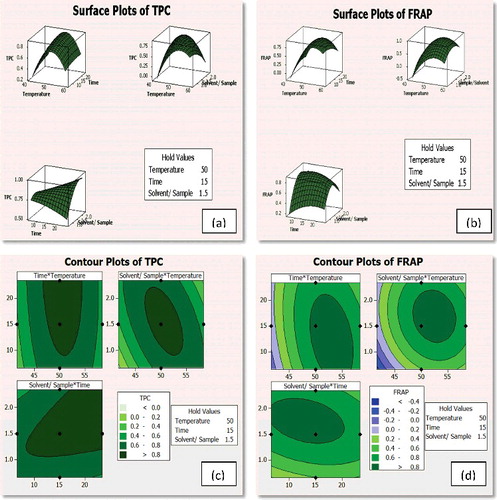
Table 5. Predicted and experimental values of the response at optimum conditions for TPC and FRAP.
The experimental yields of TPC and FRAP were 2.3 mg GAE/g dry leaf powder and 14.632 mg FeSO4·7H2O/g dry leaf powder, respectively, whereas the model-predicted yields were 2.426 mg GAE/g dry leaf powder and 14.985 mg FeSO4·7H2O/g dry leaf powder. These data indicate an appreciable correlation between the model-predicted and experimental optimum values, attesting the good fit of the model.
3.2. Synthesis and characterisation of silver nanoparticles
The extracts of N. foetida were used for initiating the green synthesis of Ag NPs with approximately 72% percentage conversion of AgNO3 to Ag NPs. Earlier, AgNO3-to-Ag NPs conversion rates of 66% had been reported using Azadirachta indica extract.[Citation23] Further, it is noteworthy that the percentages of conversion obtained in the present study were higher than those observed in the case of the biosynthesis of Ag NPs using an endophytic fungus of N. foetida under ambient experimental conditions.[Citation24] These data highlight the adeptness of the reducing atmosphere in the microwave-assisted extract, which aids in rapid and efficient bioreduction of AgNO3 to Ag NPs.
3.2.1. UV–Vis absorption spectroscopic analysis
The UV–Vis spectrum () of the as-synthesised Ag NPs indicates the presence of broad peaks at 420–510 nm. Similar observations have been reported in literature for the absorption spectra of Ag NPs in aqueous solutions.[Citation25] The broadening of the peak in these wavelength ranges could be attributed to the particle size, shape and its polydispersed nature.[Citation26]
3.2.2. Transmission electron microscopy analysis of silver nanoparticles
The functionality of any NP relies heavily on their chemical composition, shape, size and dispersity.[Citation27] Hence, the structural and morphological characterisation of the synthesised Ag NPs holds relevance with respect to their functionality.[Citation27] The TEM images of the as-synthesised Ag NPs () reveal the formation of spherical particles with an average size of 20–50 nm. Further, analysis of the particle size distribution using the ImageJ software revealed the preponderance of spherical particles with diameter in the range of 13–26 nm. The particle size histogram ((c)) also indicates that the majority of particles had diameters in the range of 13–17 nm. Further, the distinct lattice fringes observed in the micrograph support the crystalline nature of Ag NPs.
3.2.3. X-ray diffraction analysis of silver nanoparticles
The XRD patterns of the Ag NPs () indicate their crystalline nature. Diffraction peaks were centred at 32.23°, 38.08°, 46.25°, 55.14°, 58.08°, 67.46° and 77.3°. The maximum peak obtained at 32.23° corresponds to the 111 plane of crystalline Ag. The diffraction peaks at 2θ angles of 32.23° corresponding to Ag2O2, 33.08° and 55.14° to Ag2O and 46.25° corresponding to Ag10Si4O13 were visible. The presence of silicon and oxygen ions can be attributed to the glass holder used for the sample preparation and oxidation during successive analyses, respectively. The lattice constants were in accordance with the Joint Committee on Powder Diffraction Standards (JCPDS) database (file No. 04-0783), confirming the presence of Ag formed from extracts of N. foetida.[Citation28] Various researchers have also reported the incidence of similar XRD patterns for Ag NPs.[Citation29,Citation30]
3.2.4. Fourier transform infrared analysis of silver nanoparticles
FTIR spectroscopy was performed to identify the biomolecules that stabilise the metal NPs synthesised by the plant extract. The FTIR spectrum of Ag NPs () reveals the existence of diverse functional groups such as C–N, C–O–C, amide linkages and –COO–, with two types of vibrations (ie stretching and bending) in the wavelength from 4000 to 650 cm–1, which may be due to the phytochemicals and proteins that cap the synthesised Ag NPs.
The band at 3353.53 cm−1 represents a primary amine (N–H) stretching, whereas the bands at 2920.66 and 2856.06 cm−1 indicate the presence of an aromatic C–H stretch. The bands at 2354.66 and 1717.33 cm−1 correspond to the C=O bond and the bands at 1060.66 and 830.205 cm−1 represent C–O group and C–Cl alkyl halides. Similar peaks were observed for the synthesis of Ag NPs using Saraca asoca bark extract.[Citation31] According to the authors, these peaks indicate the involvement of carboxyl (–C=O), hydroxyl (–OH) and amine (–NH) functional groups of phytochemicals such as flavanoids and terpenoids that aid in reduction, capping and stabilisation of the Ag NPs. The prominence of amide functional groups, which indicates the involvement of proteins, is also noteworthy. The active role of proteins to aid in bioreduction and subsequently binding to metal surfaces to form a protective cap that prevents agglomeration and hence stabilising the particle size has been documented earlier.[Citation18] Therefore, it can be concluded that the synthesised NPs were surrounded by proteins and other metabolites such as terpenoids and flavonoids that have functional groups typical to alcohols, ketones, aldehydes and carboxylic acids.
3.3. Antimicrobial activity of silver nanoparticles
The biogenic Ag NPs exhibited a remarkable antimicrobial activity against four bacterial strains and yeast (). The diameters of the zone of inhibition (ZOI) at different concentrations (10, 30 and 50 µg /mL) of Ag NPs were assessed and the mean of three replicates with its standard deviation were determined. It was observed that at [Ag NP] of 30 µg /mL, the ZOI was 9.36 ± 0.23, 12.9 ± 0.1, 15.13 ± 0.32, 17.8 0 ± 0.26 and 20.16 ± 0.16 for B. subtilis, C. albicans, P. aeruginosa, E. coli and S. aureus, respectively. The ZOIs for S. aureus and E. coli at 30 µg/mL concentrations were found to be comparatively higher than the values reported by Kumar et al.[Citation32]
Similar antimicrobial activities of Ag NPs were earlier examined against B. subtilis and C. albicans by Sadhasivam et al.[Citation33] It has been reported that the antimicrobial activity of NPs is due to their positive charge creating an electrostatic attraction between the negatively charged cell membrane of microorganisms or due to the release of silver ions from Ag NPs, which act as bactericidal agents [Citation33] or due to the penetration of Ag NPs into the bacteria, thereby damaging the cell membrane.[Citation29,Citation34]
4. Conclusions
Microwave-assisted extraction at the optimised conditions obtained by CCD demonstrated to be the most effective method for extracting antioxidants from leaves of N. foetida. The extracts possessing reducing agents have proved to be extremely proficient in the biosynthesis of Ag NPs. Hence, the synthesis of Ag NPs from plant extracts obtained by MAE appears to be a competent alternative to the conventional methods with suitability of application in the emerging large-scale production. Additionally, the biosynthesis of Ag NPs using plant extract is economically viable and ecologically safe. The synthesised Ag NPs showed remarkable activity against a broad range of human pathogens, including S. aureus, E. coli, P. aeruginosa, B. subtilis and C. albicans.
Disclosure statement
No potential conflict of interest was reported by the authors.
References
- Huang J, Lin L, Sun D, et al. Bio-inspired synthesis of metal nanomaterials and applications. Chem Soc Rev. 2015;44:6330–6374.
- Ponarulselvam S, Panneerselvam C, Murugan K, et al. Synthesis of silver nanoparticles using leaves of Catharanthus roseus Linn. G. Don and their antiplasmodial activities. Asian Pac J Trop Biomed. 2012;2:574–580.
- Mary J, Mohan R, Bhat U. Biosynthesis of lead selenide quantum rods in marine Aspergillus terreus. Mater Lett. 2014;124:279–281.
- Hutchison JE. Greener nanoscience: a proactive approach to advancing applications and reducing implications of nanotechnology. ACS Nano. 2008;2:395–402.
- Shankar SS, Rai A, Ahmad A, et al. Rapid synthesis of Au, Ag, and bimetallic Au core-Ag shell nanoparticles using neem (Azadirachta indica) leaf broth. J. Colloid Interface Sci. 2004;275:496–502.
- Gardea-Torresdey JL, Parsons JG, Gomez E, et al. Formation and growth of Au nanoparticles inside live Alfalfa plants. Nano Lett. 2002;2:397–401.
- Shen L, Chen M, Hu L, et al. Growth and stabilization of silver nanoparticles on carbon dots and sensing application. Langmuir. 2013;29:16135–16140.
- Abbasi E, Milani M, Fekri Aval S, et al. Silver nanoparticles: synthesis methods, bio-applications and properties. Crit Rev Microbiol. 2016;42:173–180.
- Sharma VK, Yngard RA, Lin Y. Silver nanoparticles: green synthesis and their antimicrobial activities. Adv Colloid Interface Sci. 2009;145:83–96.
- Ganaie SU, Abbasi T, Anuradha J, et al. Biomimetic synthesis of silver nanoparticles using the amphibious weed ipomoea and their application in pollution control. J King Saud Univ - Sci. 2014;26:222–229.
- Panthari P, Balaji P, Joshi M, et al. Synthesis, characterization and evaluation of antibacterial efficacy, antioxidant potential of silver nanoparticle using Myrica nagi leaf extract. 2015;6:556–563.
- Qureshi MS. Characterisation and synthesis of silver nano particle. J Nanomater. 2012;2:1–4.
- Saxena A, Tripathi RM, Zafar F, et al. Green synthesis of silver nanoparticles using aqueous solution of Ficus benghalensis leaf extract and characterization of their antibacterial activity. Mater Lett. 2012;67:91–94.
- Shekhawat MS, Manokari M, Kannan N, et al. Synthesis of silver nanoparticles using Cardiospermum halicacabum L. leaf extract and their characterization. J Phytopharm JPHYTO. 2013;2:15–20. .
- Kouvaris P, Delimitis A, Zaspalis V, et al. Green synthesis and characterization of silver nanoparticles produced using Arbutus unedo leaf extract. Mater Lett. 2012;76:18–20.
- Roni M, Murugan K, Panneerselvam C, et al. Evaluation of leaf aqueous extract and synthesized silver nanoparticles using Nerium oleander against Anopheles stephensi (Diptera: Culicidae). Parasitol Res. 2013;112:981–990.
- Vivek R, Thangam R, Muthuchelian K, et al. Green biosynthesis of silver nanoparticles from Annona squamosa leaf extract and its in vitro cytotoxic effect on MCF-7 cells. Process Biochem. 2012;47:2405–2410.
- Sharma G, Sharma AR, Bhavesh R, et al. Biomolecule-mediated synthesis of selenium nanoparticles using dried Vitis vinifera (raisin) extract. Molecules. 2014;19:2761–2770.
- Zhang YX, Zheng J, Gao G, et al. Biosynthesis of gold nanoparticles using chloroplasts. Int J Nanomed. 2011;6:2899–2906. .
- Kujala TS, Loponen JM, Klika KD, et al. Phenolics and betacyanins in red beetroot (Beta vulgaris) root: distribution and effect of cold storage on the content of total phenolics and three individual compounds. J Agric Food Chem. 2000;48:5338–5342.
- Benzie IF, Strain JJ. The ferric reducing ability of plasma (FRAP) as a measure of “antioxidant power”: the FRAP assay. Anal Biochem. 1996;239:70–76.
- Bezerra MA, Santelli RE, Oliveira EP, et al. Response surface methodology (RSM) as a tool for optimization in analytical chemistry. Talanta. 2008;76:965–977.
- Namratha N, Monica PV. Synthesis of silver nanoparticles using Azadirachta indica (Neem) extract and usage in water purification. Asian J Pharm Tech.2013;3:170–174. .
- Fathima B S, Balakrishnan RM. Biosynthesis and optimization of silver nanoparticles by endophytic fungus Fusarium solani. Mater Lett. 2014;132:428–431.
- Huang J, Li Q, Sun D, et al. Biosynthesis of silver and gold nanoparticles by novel sundried Cinnamomum camphora leaf. Nanotechnology. 2007;18:105104.
- Chandran SP, Chaudhary M, Pasricha R, et al. Synthesis of gold nanotriangles and silver nanoparticles using Aloe vera plant extract. Biotechnol Prog.2006;22:577–583.
- Noguez C. Surface plasmons on metal nanoparticles: the influence of shape and physical environment. J Phys Chem C. 2007;111:3806–3819.
- The International Centre for Diffraction Data – ICDD – a non-profit scientific organization dedicated to collecting, editing, publishing, and distributing powder diffraction data [Internet]. [cited 2015 Sep 16]. Available from: http://www.icdd.com/.
- Li W-R, Xie X-B, Shi Q-S, et al. Antibacterial activity and mechanism of silver nanoparticles on Escherichia coli. Appl Microbiol Biotechnol. 2010;85:1115–1122.
- Kim K-J, Sung WS, Suh BK, et al. Antifungal activity and mode of action of silver nano-particles on Candida albicans. Biometals. 2009;22:235–242.
- Banerjee P, Nath D. A phytochemical approach to synthesize silver nanoparticles for non-toxic biomedical application and study on their antibacterial efficacy. Nanosci Technol.2015;2:1–14.
- Kumar DA, Palanichamy V, Roopan SM. Green synthesis of silver nanoparticles using Alternanthera dentata leaf extract at room temperature and their antimicrobial activity. Spectrochim Acta A. 2014;127:168–171.
- Sadhasivam S, Shanmugam P, Yun K. Biosynthesis of silver nanoparticles by Streptomyces hygroscopicus and antimicrobial activity against medically important pathogenic microorganisms. Colloids Surf B Biointerfaces. 2010;81:358–362.
- Gopinath V, Priyadarshini S, Meera Priyadharsshini N, et al. Biogenic synthesis of antibacterial silver chloride nanoparticles using leaf extracts of Cissus quadrangularis Linn. Mater Lett. 2013;91:224–227.