ABSTRACT
Magnesium layered hydroxide (MLH) intercalated with anionic 3-(4-methoxyphenyl)propionic acid (MPP) was synthesised by a direct reaction method using magnesium oxide and MPP as precursors. A further coating of chitosan was applied on the external surface of MLH–MPP nanocomposite to form a new material, named MLH–MPP/chitosan nanocomposite. The XRD pattern showed an intense and sharp peak at basal spacing 18.9 Å, proving that MPP anions were successfully intercalated into the interlayer gallery of MLH in a monolayer arrangement. The XRD pattern of the MLH–MPP/chitosan nanocomposite shows similar peaks with the MLH–MPP nanocomposite. The result was also supported by FTIR spectra and elemental analysis. TGA/DTG spectra showed that the thermal stabilities of the guest anion in the both nanocomposites were markedly enhanced. A controlled-release study of the MPP ion from the MLH–MPP/chitosan nanocomposite showed a slower release compared to MLH–MPP nanocomposite with an initial rapid release and slow release thereafter. Meanwhile, the release behaviours of MPP ions from both nanocomposites were governed by pseudo-second order kinetics. This result highlights the potential of the nanocomposite as an encapsulated material for the controlled-release formulation of MPP anions.
1. Introduction
Recently, interest in two-dimensional (2D) nanosheets has increased tremendously because of their unique physical and chemical properties. The intercalation of 2D layered materials suggested a new opportunity to utilise hybrid materials at nanoscale dimensions, which are also known as nanocomposites.[Citation1] Layered metal hydroxide (LMH) has the general formula M2+(OH)2−x (Am−)x/m·nH2O, where M2+ is the metal cation such as Zn2+, Ni2+, Ca2+ or Mg2+ and Am− is the counter ion.[Citation2] LMH structures are made up of anions and positively charged layers, which can be expanded depending on the nature of the anions that are intercalated.[Citation3] LMHs have been frequently used as drug carriers [Citation4] and for controlled-release herbicides,[Citation5,Citation6] besides other applications such as flame retardants,[Citation7] UV screening enhancers,[Citation3] and for polymer fabrication.[Citation8]
In the last decade, research based on metal oxides, especially MgO, has become extensive. The literature shows that MgO is an adaptable oxide material that has extensive applications such as in catalysis,[Citation9] photocatalysis,[Citation10] hazardous waste treatment,[Citation11] and as an arsenic adsorbent in water treatment.[Citation12] MgO is generally known by its unique properties related to the size of the nanoparticle.[Citation13,Citation14] Due to its distinctive features, MgO can be perfectly assigned as magnesium layered hydroxide (MLH) that acts as a host for organic materials.
Currently, the agricultural sector is generating wider concern in society, and the use of pesticides has increased over time. Increased pesticide use leads to excess pesticide in the soil that creates other problems such as water pollution. Water pollution is caused by runoff of pesticides into surface water and groundwater.[Citation15] Controlled-release formulation (CRF) is the most effective way to apply pesticides while reducing environmental pollution. CRF minimises the quantity of pesticide required for the same activity; thus, it prevents the use of excess pesticides helping to reduce non-target effects. CRF also produces better crop quality and assists in increasing crop yield.[Citation1] Based on previous research, there are many active agents such as drugs,[Citation16,Citation17] pharmaceuticals,[Citation18] herbicides,[Citation5,Citation6] pesticides and plant growth regulators that have been used in CRF. All of the active agents need to be intercalated into the LMH before they can be applied as CRF.
In order to enhance the properties of LMH, a modification on the surface of the nanocomposite is one alternative. Surface modification of nanocomposites by surfactants or polymers or both can alter particle size, size distribution, particle morphology, surface chemistry, surface hydrophobicity and encapsulation efficiency of active agents.[Citation19] Coating active agents with polymer reduces their dissolution rate, thus increasing their efficiency in CRF. There are some studies concerning the coating of agrochemicals. Silva et al. studied the use of chitosan as a carrier system for the paraquat herbicide and according to that research, there is a difference in the release profiles between free and coated herbicides.[Citation20] The use of polymeric membranes as a coating also helped to control the release rate of fertilisers, thus reducing fertiliser losses and minimising environmental pollution.[Citation21] The selection of the surface coating material is important and one of the focal elements for successful coating of nanocomposites. Previous studies have used chitosan as a coating material.[Citation20,Citation22] Chitosan was chosen as a coating material based on its properties, including its biocompatibility, and most crucially its degradation products are non-toxic and non-carcinogenic. Therefore, chitosan can be readily used in many areas of agro-industry.[Citation23]
In the present work, we report and discuss the formation of a layered organic–inorganic, MLH–MPP nanocomposite by intercalation of MPP ions into MLH for CRF purposes. The MLH–MPP nanocomposite was coated with chitosan in order to increase its properties and effectiveness in CRF, and thus named as MLH–MPP/chitosan nanocomposite. MLH–MPP nanocomposite was prepared via a direct reaction method and its structure and morphology were studied by PXRD, FTIR, CHNS, ICP/OES, TGA/DTG, SEM and TEM. These instruments were also used to confirm success in the formation of the MLH–MPP/chitosan nanocomposite. Up to now, MLH has not yet received a lot of attention from researchers. Intensive research has been focused on layered hydroxide salts (LHS), especially zinc layered hydroxide.[Citation3,Citation6] Based on previous reports, no study of intercalation and controlled release of MPP from MLH has been carried out. Several concentrations of sodium nitrate solution were used in order to study the controlled release of MPP anions from both nanocomposites. Five kinetic orders were used to study the release behaviour of the MPP anion.
2. Experimental section
2.1. Materials
Magnesium oxide (MgO) and 3-(4-methoxyphenyl)propionic acid (MPP) were purchased from Acros Organics and chitosan from Sigma-Aldrich. All chemicals were of analytical grade and used without further purification. Deionised water was used in the synthesis of nanocomposites and for the preparation of solutions.
2.2. Synthesis of MLH–MPP nanocomposite
A direct reaction method was used to prepare MLH–MPP nanocomposite with MgO as a host and MPP as guest anions in an aqueous environment as previously documented elsewhere.[Citation6] MPP solutions (0.25–0.60 M) were prepared by dissolution of the required amounts of MPP in 50 mL of 99.8% methanol. First, 0.50 g of MgO was diluted with 20 mL deionised water. The resulting MgO solution was then mixed with MPP solution and the resulting solution was stirred for 2 hours. Next, the precipitate was aged in an oil bath shaker at a temperature of 70 °C for one day.[Citation6] The slurry was then centrifuged and washed with deionised water. Last, the sample was dried in an oven for approximately one day, then ground and kept in a labelled bottle.
2.3. Synthesis of MLH–MPP/chitosan nanocomposite
MLH–MPP/chitosan nanocomposite was synthesised according to the reported protocol.[Citation19,Citation24] Chitosan (0.05 g) was dissolved in 50 mL dilute acetic acid. Then, the MLH–MPP nanocomposite (0.1 g) was added into the prepared chitosan solution, with stirring for 10 hours. The synthesised nanocomposite was centrifuged and dried in an oven overnight at 60 °C. After this, samples were treated and stored in the same way as for the previous samples.
2.4. Characterisation
The XRD patterns were obtained on a Power Diffraction Bruker AXS (model D8 Advance, wavelength of 1.5406 Å) operating at a voltage of 60 kV and a current of 60 mA, using CuKα radiation. The infrared spectra were recorded on a Thermo Nicolet 6700 Fourier Transform Infrared Spectrometer using the KBr pellet technique. Thermal analyses were performed using a Perkin Elmer Pyris 1 TGA Thermo Balance at a heating rate of 20 °C min−1. The chemical compositions of the samples were analysed for Mg2+, hydrogen and carbon by ICP-OES, model Agilent (720 Axial) and CHNS, model Thermofinnigan (Flash EA 1112). Morphology was observed by using an FESEM instrument (Hitachi model SU 8020 UHR). TEM micrographs were obtained using the same instrument as for FESEM.
2.5. Release study of MPP
Several initial concentrations of sodium nitrate solution were used to study the release of MPP from the interlayer of both nanocomposites. About 0.6 mg of nanocomposite was added into a cuvette. Then, the cuvette was covered with a lid and wrapped with a parafilm. The UV–Vis instrument was set up with correct data for the analysis. The release of MPP from the interlayer of both nanocomposites was measured at a predetermined time at λmax = 219.0 nm. The release of MPP was analysed with a Perkin Elmer UV–Vis spectrophotometer Lambda 25.
3. Results and discussion
3.1. PXRD analysis
XRD was the preliminary technique used to verify the successful intercalation of herbicide into the interlayer of LMH. shows the XRD patterns of MgO, MPP, and MLH–MPP nanocomposite. MgO has five main diffraction peaks located at 36.78°, 42.76°, 62.14°, 74.54° and 78.46° which could be indexed to (111), (200), (220), (311) and (222), respectively.[Citation25] The concentration of MPP used to synthesise MLH–MPP nanocomposite was 0.25–0.60 M. The basal spacing of MLH–MPP nanocomposite was found to be 18.9 Å and 19.1 Å for 0.4 M and 0.6 M of MPP, respectively, based on three harmonics ((003), (006) and (009)). The formation of MLH–MPP nanocomposite occurred in a three step dissociation–deposition mechanism, as described elsewhere.[Citation3,Citation4,Citation26] The mechanism is described in EquationEquations (1(1) )–(Equation3
(3) ) below:
(1)
(2)
(3)
Figure 1. PXRD patterns of MgO, MPP and MLH–MPP nanocomposite at various concentrations of MPP, 0.25 M, 0.40 M and 0.60 M.
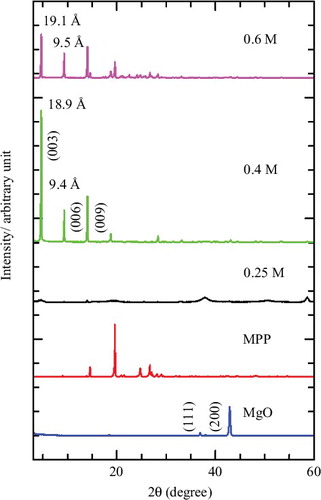
An intense and sharp peak of intercalation resulted from a successful intercalation with a high crystallinity nanocomposite.[Citation27]
MLH–MPP nanocomposite at 0.4 M was chosen for further characterisation and controlled-release study, as this concentration had the highest intensity peak at lower 2θ angle, which indicates that the inclusion of MPP into the MLH interlayer was successful. The XRD pattern for resulting nanocomposite shows there were no MgO and anion peaks at high 2θ angle. It shows that the conditions were favourable for obtaining a well-ordered and high purity layered structure with superior crystallinity. As well as undergoing a further characterisation and controlled-release study, 0.4 M MLH–MPP nanocomposite was also selected for coating with chitosan to form MLH–MPP/chitosan nanocomposite.
The XRD pattern of MLH–MPP/chitosan is shown in . For MLH–MPP nanocomposite coated with chitosan, the XRD patterns of the resulting materials ((b)(1)) are superpositions of those of MgO with the coating material ((b)(2)) and the free polymers ((b)(3)). Based on the XRD pattern of the coated nanocomposite, peaks appearing at lower 2θ angle indicate that the successful intercalation of MPP anion between the MLH was not exchanged with chitosan. The basal spacing of the coated nanocomposite was smaller than for MLH–MPP nanocomposite, which can be attributed to slightly different orientations of MPP anion in the interlayer. This evidence shows that the addition of chitosan into the MLH–MPP nanocomposite only resulted in the adsorption of the polymer on the surface of the MLH–MPP nanocomposite.
3.2. FTIR analysis
shows the FTIR spectra for MgO, MPP and the MLH–MPP nanocomposite. The 3600–3780 cm−1 region was related to O–H stretching vibrations.[Citation25] Based on MgO spectrum, the band at 3697 cm−1 represents multi-coordinate hydroxyl groups. MgO can chemisorb H2O and CO2 from the surroundings due to its surface acid–base properties.[Citation10,Citation28,Citation29] A sharp band corresponding to the adsorption of gas-phase CO2 was visible at 2337 cm−1 and 2360 cm−1 and the band at 1455 cm−1 appears in the MgO spectrum and is attributed to CO32− absorption bands,[Citation10,Citation25] whereas the peaks at 3434 cm−1 and 1629 cm−1 are assigned to the stretching and bending vibration of the hydroxyl groups and adsorbed water.[Citation30] The peak at 429 cm−1 corresponds to the stretching vibration of Mg–O.[Citation31]
Based on the MPP spectrum, the vibration bands of C=O for carboxylic acid that bonded with aliphatic alkyl groups were centred at 1708 and 1590 cm−1. The band at 1316 cm−1 was assigned to vibration of the aromatic ring C=C bonds,[Citation6] whereas the bands at 1183 and 1038 cm−1 were due to asymmetrical and symmetrical C–O–C stretching bands, respectively.
Meanwhile, most of the bands in the FTIR spectrum for the MLH–MPP nanocomposite resemble the bands in the MPP spectra, indicating a successful intercalation of MPP into the MLH interlayer. The broad peak obtained in the range of 3200–3600 cm−1 is due to stretching vibration mode of hydroxyl groups and water molecules and reveals the existence of hydrogen bonds among the layers of nanocomposite.[Citation32] However, the bands around 1708–1640 cm−1 (C=O for carboxylic acid) have disappeared and are replaced by bands at 1445 and 1541 cm−1 assigned to carboxylate ions, CO2−. This shows that the species that intercalate into the MLH interlayers are in the form of the 3-(4-methoxyphenyl)propionate ion (CH3OC6H4CH2CH2COO−). A few of the bands are slightly shifted, caused by the intercalation and interaction between MPP anions and positively charged MgO as the host matrix.[Citation3] The rest of the vibration bands are listed in
Table 1. Fourier transform infrared spectra for MPP and MLH–MPP nanocomposite.
shows evidence that supports the successful formation of chitosan coated MLH–MPP nanocomposite. (a) and (b) shows the FTIR spectra of chitosan and MLH–MPP/chitosan nanocomposite, respectively. In both spectra, hydroxyl stretching is overlaid with the N–H stretching vibration band at the peak at 3427 cm−1.[Citation33] The stretching peak of C–H associated with the ring methane hydrogen atoms appears at 2918 cm−1.[Citation34] The peak visible at 1640 cm−1 is attributed to the N–H bending vibrational band,[Citation33] while 1379 and 1256 cm−1 peaks correspond to the stretching vibrations of C–N for chitosan and MLH–MPP/chitosan nanocomposite, respectively. The peaks visible at 1411 and 1513 cm−1 are related to the symmetrical and asymmetrical stretching vibrations of the carboxylate groups.[Citation35] Absorption bands in the range of 1000–1200 cm−1 are attributed to the stretching characteristics of –C–O– on the polysaccharide skeleton.[Citation34] The peaks appearing at 3697 cm−1 and 431 cm−1 for the MLH–MPP/chitosan nanocomposite spectrum reveal the presence of multi-coordinate hydroxyl groups of MgO and metal–oxygen bonds in the brucite-like lattice, respectively.[Citation36,Citation37] These peaks indicate that the MLH–MPP nanocomposite is present in the coated nanocomposite.
3.3. Spatial orientation of MPP
The 3D molecular size of MPP obtained using a Chem3D Ultra 8.0 is shown in (a). The x, y and z axes of MPP represent the width, thickness and length of the respective nanocomposite and were found to be 6.5, 6.5, and 12.8 Å, respectively. The proposed spatial orientation of MPP anion in interlayers of MLH ((b)) is based on the basal spacing obtained from PXRD analysis, which is 18.9 Å. The expected gallery height was 8.9 Å, which can be obtained by subtracting the thickness of MLH layer (4.8 Å) [Citation38] and 2.6 Å for each layer of zinc tetrahedron.[Citation27] Considering the charge density of the layer and anion dimension, the anions must orient themselves in a horizontal monolayer arrangement. Electrostatic interactions and hydrogen bonds exist between the oxygen atom in the carboxylate groups and the MLH layers.[Citation39] Thus, this arrangement will lead to a stronger interaction between the host layers and the anion.
3.4. Elemental analysis
The chemical composition of the MLH–MPP nanocomposite was analysed by using CHNS and ICP-OES. The results are shown in . As expected, the MLH–MPP nanocomposite contained both Mg and MPP anions, which indicated that MPP was successfully intercalated into the MLH interlayer. shows that 30.87% of Mg was present in pure MgO, meanwhile, 19.23% of Mg was present in the MLH–MPP nanocomposite and 42.19% of the MPP anions were successfully intercalated into the MLH interlayer. On the basis of thermal and elemental studies, the proposed chemical formula of the MLH–MPP nanocomposite was Mg(OH)1.6(CH3OC6H4CH2CH2COO−)0.4·1.09H2O.
Table 2. Analysed chemical composition of MgO and the MLH–MPP nanocomposite.
3.5. Thermal analysis
The thermal decomposition behaviour of MgO, MPP and the MLH–MPP nanocomposite was investigated using TGA-DTG studies. shows TGA and DTG profiles of MgO, MPP, MLH–MPP nanocomposite, chitosan and MLH–MPP/chitosan nanocomposite. Two weight loss steps were found during the decomposition of MgO. The endothermic peaks observed at 120 °C and 357 °C relate to an escapement of absorbed water from the air and decomposition of Mg(OH)2 to MgO nanoparticles.[Citation14,Citation40] The thermal analysis curve of the pure MPP shows single stage decomposition at 258 °C with 98.8% weight loss. Three-stage decomposition of the MLH–MPP nanocomposite can be identified at 88 °C, 373 °C and 526 °C with a weight loss of 13.7%, 51.8% and 10.7%, respectively. The first weight loss was due to the removal of water at the physisorption and the interlayer of the sample.[Citation14,Citation41] The second stage was due to dehydroxylation of the hydroxide layer and partial decomposition of MPP anions.[Citation42] The third stage, decomposition temperature up to 526 °C, is due to the complete decomposition of intercalated MPP anions.[Citation43] An increase in the decomposition temperature of MPP from 258 °C to 526 °C indicated that the intercalation of these herbicide anions into the MLH interlamellae enhanced the thermal stability of the intercalated herbicide anions compared to free herbicide.
Figure 6. TGA/DTG thermograms of (a) MLH–MPP nanocomposite, (b) MPP, (c) MgO, (d) MLH–MPP/chitosan nanocomposite and (e) chitosan.
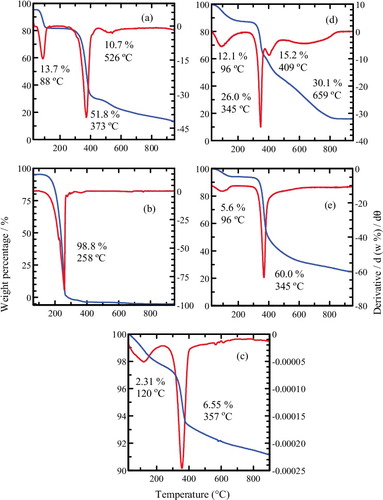
The decomposition of chitosan occurred at two different temperatures, 96 °C and 345 °C. As mentioned above, a weight loss below 100 °C was attributed to the loss of moisture. The percentage decomposition of chitosan at 345 °C was 60.0%. This is due to the dehydration of the saccharide rings and the decomposition of deacetylated units of chitosan.[Citation44] (d) shows that the thermal decomposition of MLH–MPP/chitosan progressed through four major steps of weight loss at 96 °C, 345 °C, 409 °C and 659 °C, with total weight losses of 83.4%. The total weight loss of MLH–MPP nanocomposite was 76.2%. By comparing these weight losses, it can be concluded that the LMH–MPP nanocomposite was coated with 7.2% of chitosan.[Citation19] The results of thermal analysis for all the samples are summarised in . It is shown that coating of the nanocomposite resulted in an improvement in the degradation behaviour, with an increase in the degradation temperature of the nanocomposites.
Table 3. TGA/DTG data of weight loss for MPP and MLH–MPP nanocomposite and MLH–MPP/chitosan nanocomposite.
3.6. Morphological analysis
shows the surface morphology of commercial MgO, MLH–MPP nanocomposite, chitosan and MLH–MPP/chitosan nanocomposite analysed by using FESEM. The morphology of MgO depends on its synthesis method. The SEM image of MgO shows the morphology of aggregated small plates,[Citation45] while MLH–MPP nanocomposite shows a flake-like structure. Chitosan ((c)) shows a compact and smooth surface.[Citation33] The coating of MLH–MPP nanocomposite by chitosan could be confirmed in the FESEM image, in which it is possible to clearly differentiate the coated and uncoated nanocomposites. The flake-like structure of the MLH–MPP nanocomposite was not so clear after coating with chitosan.
3.7. Transmission electron microscopy analysis
TEM micrographs of MLH–MPP nanocomposite and MLH–MPP/chitosan nanocomposite are shown in (a) and (b), respectively. The main difference between these two pictures is the presence of a lighter polymer shell.[Citation46] In (b), MLH–MPP nanocomposite exists as a dark centre surrounded by a lighter shell, which indicates the presence of chitosan in contrast to (a).
3.8. MPP herbicide release properties
The release of MPP from the interlayer of nanocomposite was performed using a release solution at several concentrations (5.0 × 10−6–8.0 × 10−4 M) of aqueous sodium nitrate (NaNO3) solution. NaNO3 was chosen as a release solution in this study because NO3− is one of the components of rain water.[Citation5] Besides, nitrates are one of the most problematic and most common potential groundwater contaminants.[Citation47] Sources of nitrate in groundwater can be divided into four categories which are natural sources, waste materials, row crop agriculture and irrigated agriculture.[Citation48] Local research in Malaysia found that nitrate could get into groundwater very quickly during the month of fertilisation and it was revealed that, on average, 15% of drinking well samples had NO3-N concentrations above the maximum acceptable level of 10 mg L−1.[Citation49] In 2004, a study was conducted by the United States Environmental Protection Agency (USEPA) showing that excessive levels of nitrate in drinking water can cause serious illness and even death. After consumption, nitrate is converted into nitrites. In general, nitrates and nitrites have the potential to cause shortness of breath, blue baby syndrome in infant, diuresis, increased starchy deposits and haemorrhaging of the spleen.[Citation50]
Release of MPP was determined at λmax = 219.0 nm. shows the release profile of MPP from the interlayer of the MLH–MPP nanocomposite into aqueous solutions containing various concentration of NaNO3. The maximum accumulated releases of MPP into the release solutions were 84% (5.0 × 10−6 M), 85% (1.0 × 10−5 M) and 86% (8.0 × 10−4 M) with equilibrium time of 530 min, 500 min and 140 min, respectively. The release of MPP from MLH–MPP/chitosan nanocomposite showed different behaviour compared to the MLH–MPP nanocomposite. Based on , the maximum accumulated release of MPP from coated nanocomposite was 10% (5.0 × 10−6 M), 14% (1.0 × 10−5 M) and 81% (8.0 × 10−4 M) and equilibrium was achieved at 1446 min, 702 min and 151 min, respectively. The release of MPP from coated nanocomposite showed a lower percentage compared to uncoated nanocomposite, which is due to an increase in degree of protection of the coated nanocomposite. This result showed that coating of MLH–MPP nanocomposite with chitosan enhanced the protection of MPP.
Figure 9. Release profile of MPP from the interlamellae of the MLH–MPP nanocomposite into aqueous solutions containing various concentrations of NaNO3.
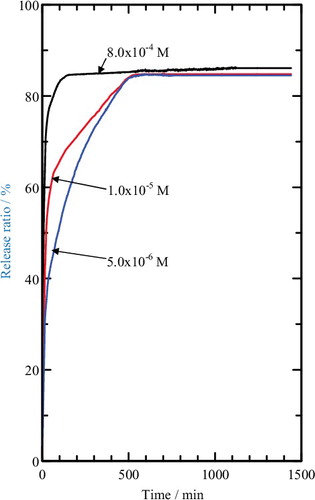
Figure 10. Release profile of MPP from the interlamellae of the MLH–MPP/chitosan nanocomposite into aqueous solutions containing various concentrations of NaNO3.
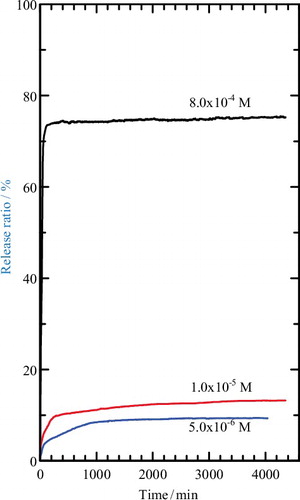
Previous work has shown that the release behaviour of dissimilar anions and hosts are quite different.[Citation19,Citation51,Citation52] The release behaviour of the nanocomposite was strongly associated with its characteristics. Some of these characteristics are the size of the guest anion, the method of synthesis, the anion affinity in the release medium, the particle size, the density and, finally, chemical interactions between the host and the guest anion.[Citation53–55]
For both and , the maximum accumulated release increased with increasing nitrate concentration. Normally, the initial release is rapid, and slows thereafter until equilibrium is achieved. This observation is caused by the presence of many sacrificial anions. Sacrificial anions act as a driving force to boost the release of MPP anions into the release solution. Ion exchange occurs when the sacrificial anion has a high affinity towards the LMH compared to the existing counter anion.[Citation56] The release rate is closely related to the electrostatic interaction between anions and the positively charged layer of layered hydroxide materials. Therefore, the initial fast release of MPP from the interlayer of nanocomposites can be related to the presence of a high density of nitrate anions, leading to a strong electrostatic interaction between nitrate anions and the positively charged layer, MLH.
3.9. Kinetic study
In this study, five different kinetic models were used to observe the release behaviour of MPP from MLH–MPP nanocomposite and MLH–MPP/chitosan nanocomposite into sodium nitrate solutions. The model used in the kinetic study of herbicide-intercalated layered hydroxides nanocomposite was zeroth order (4),[Citation57] first order (5),[Citation58] pseudo-second order (6),[Citation19] parabolic diffusion (7) [Citation59] and Fickian diffusion (8) [Citation5] model. The values refer to the percentage release of herbicide anions at time
, while
and
are the initial and final concentration of herbicide anions, respectively.
is an empirical parameter describing the release mechanism and
is a constant. The parameter correlation coefficients,
, rate constant,
, and
values are calculated from the corresponding equations.
(4)
(5)
(6)
(7)
(8)
The cumulative releases of the MPP anion were fitted to the listed kinetic models and are plotted in and . In general, there are two governing mechanisms in the release system of layered double hydroxide (LDH): anion diffusion through particles [Citation43] and dissolution of the LDH particles.[Citation16,Citation60] The best fit graph can be obtained when the resultant values are closest to 1. As shown in and , zeroth order seems to be unsuitable for describing the mechanism due to its low
value. The first order, parabolic diffusion and Fickian diffusion models also result in poor linearity. The fitting of the release data for MLH–MPP nanocomposite and MLH–MPP/chitosan nanocomposite were best achieved with the pseudo-second order model as is evident by the high
values. Pseudo-second order elucidates that the release of herbicide from the inorganic MLH interlayer is involved in the dissolution of nanocomposite as well as ion exchange between the intercalated anions in the MLH interlayer and nitrate anions in the aqueous solution.[Citation5]
Figure 11. Fitting of the data of MPP released from MLH–MPP nanocomposite into aqueous solutions containing various concentration of NaNO3; 5.0 × 10−6 M (square), 1.0 × 10−5 M (triangle) and 8.0 × 10−4 M (circle) to the (a) zeroth, (b) first, (c) pseudo-second order, (d) parabolic diffusion and (e) Fickian diffusion models.
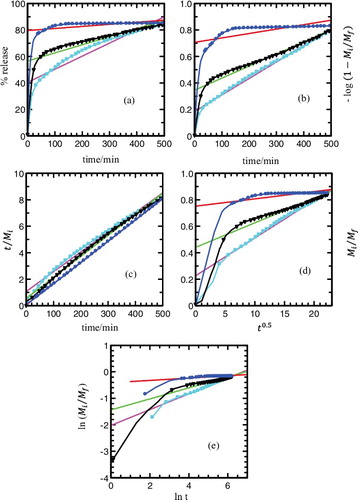
Figure 12. Fitting of the data of MPP released from MLH–MPP/chitosan nanocomposite into aqueous solutions containing various concentrations of NaNO3; 5.0 × 10−6 M (square), 1.0 × 10−5 M (triangle) and 8.0 × 10−4 (circle) to the (a) zeroth, (b) first, (c) pseudo-second order, (d) parabolic diffusion and (e) Fickian diffusion models.
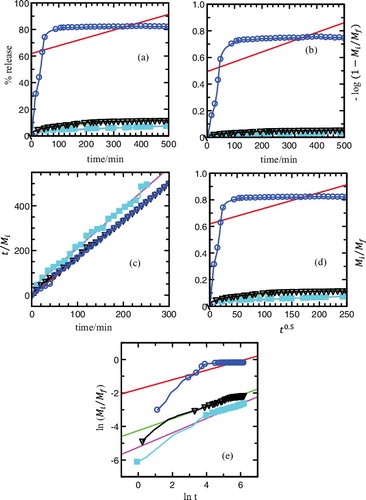
Table 4. Correlation coefficients, rate constants, half-lifes ( and constants obtained from the data of MPP release from MLH–MPP nanocomposite into NaNO3.
Table 5. Correlation coefficients, rate constants, half-lifes ( and constants obtained from the data of MPP release from MLH–MPP/chitosan nanocomposite into NaNO3.
For all the nanocomposites, is the time taken for the 50% of the MPP herbicide anions to be released from the interlayers.
values decrease as the concentration of NaNO3 increases. This is because as the concentration of nitrate increases, more nitrate anions are available to get involved in ion exchange with MPP anions, thus lowering the
value. This pattern is similar for the
values in the release of hippuric acid (HA) from hippuric acid nanocomposite (HAN) [Citation61] and the release of cloprop from cloprop–LDH nanocomposite.[Citation5] The result shows that MLH can be used as an effective inorganic matrix for herbicide anion storage. Moreover, MLH can aid controlled delivery at the appropriate time, which is a crucial aspect in the agricultural sector.
4. Conclusion
MLH–MPP nanocomposite obtained by a successful intercalation of MPP into MLH was synthesised for the first time via direct reaction using MgO as a starting material. This was proven by its XRD pattern at a basal spacing of 18.9 Å with a horizontal monolayer arrangement. MLH–MPP/chitosan nanocomposite was formed without changing the previously intercalated anion. The coating with chitosan improved the thermal stability of the MLH–MPP nanocomposite. Percentage release of MPP was affected by the concentration of aqueous nitrate solution used. The release behaviour of MPP into nitrate solution from both MLH–MPP nanocomposite and MLH–MPP/chitosan nanocomposite followed pseudo-second order kinetics. The MLH–MPP/chitosan nanocomposite exhibits slow release of MPP compared to uncoated nanocomposite. The present results demonstrate the potential application of a LMH, particularly MLH as the host for the preparation of nanocomposite compounds for controlled-release formulations of herbicides in agriculture.
Acknowledgments
The authors wish to thank UPSI for all affords and support in this research.
Disclosure statement
No potential conflict of interest was reported by the authors.
Additional information
Funding
References
- Hussein MZ, Rahman NSSA, Sarijo SH, et al. Synthesis of a monophasic nanohybrid for a controlled release formulation of two active agents simultaneously. Appl Clay Sci. 2012;58:60–66.
- Arizaga GGC, Satyanarayana KG, Wypych F. Layered hydroxide salts: synthesis, properties and potential applications. Solid State Ionics. 2007;178:1143–1162.
- Mohsin SMN, Hussein MZ, Sarijo SH, et al. Synthesis of (cinnamate–zinc layered hydroxide) intercalation compound for sunscreen application. Chem Cent J. 2013;7:1–26.
- Hussein MZ, Al Ali SH, Zainal Z, et al. Development of antiproliferative nanohybrid compound with controlled release property using ellagic acid as the active agent. Int J Nanomed. 2011;6:1373–1383.
- Hashim N, Hussein MZ, Isa IM, et al. Synthesis and controlled release of cloprop herbicides from cloprop-layered double hydroxide and cloprop–zinc-layered hydroxide nanocomposites. Open J Inorg Chem. 2014;4:1–9.
- Bashi AM, Hussein MZ, Zainal Z, et al. Synthesis and controlled release properties of 2,4-dichlorophenoxy acetate–zinc layered hydroxide nanohybrid. J Solid State Chem. 2013;203:19–24.
- Zhang P, Kang M, Hu Y. Influence of layered zinc hydroxide nitrate on thermal properties of paraffin/intumescent flame retardant as a phase change material. J Therm Anal Calorim. 2013;112:1199–1205.
- Zimmermann A, Jaerger S, Zawadzki SF, et al. Synthetic zinc layered hydroxide salts intercalated with anionic azo dyes as fillers into high-density polyethylene composites: first insights. J Polym Res. 2013;20:1–11.
- Duan K, Thompson LG, Yao T, et al. A 1000 year history of atmospheric sulfate concentrations in southern Asia as recorded by a Himalayan ice core. Geophys Res Lett. 2007;34:1810–1813.
- Mageshwari K, Mali SS, Sathyamoorthy R, et al. Template-free synthesis of MgO nanoparticles for effective photocatalytic applications. Powder Technol. 2013;249:456–462.
- Shah MA, Qurashi A. Novel surfactant-free synthesis of MgO nanoflakes. J Alloys Compd. 2009;482:548–551.
- Tresintsi S, Simeonidis K, Katsikini M, et al. A novel approach for arsenic adsorbents regeneration using MgO. J Hazard Mater. 2014;265:217–225.
- Azhar AZA, Mohamad H, Ratnam MM, et al. Effect of MgO particle size on the microstructure, mechanical properties and wear performance of ZTA–MgO ceramic cutting inserts. Int J Refract Met Hard Mater. 2011;29:456–461.
- Vatsha B, Tetyana P, Shumbula PM, et al. Effects of precipitation temperature on nanoparticle surface area and antibacterial behaviour of Mg(OH)2 and MgO nanoparticles. J Biomater Nanobiotechnol. 2013;4:365–373.
- Laganà A, Bacaloni A, Leva ID, et al. Occurrence and determination of herbicides and their major transformation products in environmental waters. Anal Chim Acta 2002;462:187–198.
- Hasan S, Al-Ali H, Al-Qubaisi M, et al. Controlled-release formulation of antihistamine based on cetirizine zinc-layered hydroxide nanocomposites and its effect on histamine release from basophilic leukemia (RBL-2H3) cells. Int J Nanomed. 2012;7:3351–3363.
- San Román MS, Holgado MJ, Salinas B, et al. Drug release from layered double hydroxides and from their polylactic acid (PLA) nanocomposites. Appl Clay Sci. 2013;71:1–7.
- Khan AI, Lei L, Norquist AJ, et al. Intercalation and controlled release of pharmaceutically active compounds from layered double hydroxide. Chem Commun. 2001;22:2342–2347.
- Kura AU, Hussein-Al-Ali SH, Hussein MZ, et al. Preparation of Tween 80-Zn/Al-levodopa-layered double hydroxides nanocomposite for drug delivery system. Sci World J. 2014;1–10.
- Silva MDS, Cocenza DS, Grillo R, et al. Paraquat-loaded alginate/chitosan nanoparticles: preparation, characterization and soil sorption studies. J Hazard Mater. 2011;190:366–374.
- Han X, Chen S, Hu X. Controlled-release fertilizer encapsulated by starch/polyvinyl alcohol coating. Desalination 2009;240:21–26.
- Wu L, Liu M. Preparation and properties of chitosan-coated NPK compound fertilizer with controlled-release and water-retention. Carbohydr Polym. 2008;72:240–247.
- Alves NM, Mano JF. Chitosan derivatives obtained by chemical modifications for biomedical and environmental applications. Int J Biol Macromol. 2008;43:401–414.
- Kameshima Y, Sasaki H, Isobe T, et al. Synthesis of composites of sodium oleate/Mg–Al–ascorbic acid-layered double hydroxides for drug delivery applications. Int J Pharm. 2009;381:34–39.
- Li H, Li M, Qiu G, et al. Synthesis and characterization of MgO nanocrystals for biosensing applications. J Alloys Compd. 2015;632:639–644.
- Miao J, Xue M, Itoh H, et al. Hydrothermal synthesis of layered hydroxide zinc benzoate compounds and their exfoliation reactions. J Mater Chem. 2006;16:474–480.
- Cavani F, Trifiro F, Vaccari A. Hydrotalcite-type anionic clays: preparation, properties and applications. Catal Today. 1991;11:173–301.
- Selvam NCS, Kumar RT, Kennedy LJ, et al. Comparative study of microwave and conventional methods for the preparation and optical properties of novel MgO-micro and nano-structures. J Alloys Compd. 2011;509:9809–9815.
- Sutradhar N, Sinhamahapatra A, Roy B, et al. Preparation of MgO nano-rods with strong catalytic activity via hydrated basic magnesium carbonates. Mater Res Bull. 2011;46:2163–2167.
- Zhou J, Yang S, Yu J. Facile fabrication of mesoporous MgO microspheres and their enhanced adsorption performance for phosphate from aqueous solutions. Colloids Surf A Physicochem Eng Asp. 2011;379:102–108.
- Niu H, Yang Q, Tang K, et al. Large-scale synthesis of single-crystalline MgO with bone-like nanostructures. J Nanoparticle Res. 2006;8:881–888.
- Tao Q, Zhang Y, Zhang X, et al. Synthesis and characterization of layered double hydroxides with a high aspect ratio. J Solid State Chem. 2006;179:708–715.
- De S, Mohanty S, Nayak SK. Structure–property relationship of layered metal oxide phosphonate/chitosan nanohybrids for transducer in biosensing device. J Mater Eng Perform. 2015;24:114–127.
- Wu D, Chang PR, Ma X. Preparation and properties of layered double hydroxide–carboxymethylcellulose sodium/glycerol plasticized starch nanocomposites. Carbohydr Polym. 2011;86:877–882.
- Rosca C, Popa MI, Lisa G, et al. Interaction of chitosan with natural or synthetic anionic polyelectrolytes. 1. The chitosan–carboxymethylcellulose complex. Carbohydr Polym. 2005;62:35–41.
- Darder M, Blanco-Lopez M, Aranda P, et al. Bio-nanocomposites based on layered double hydroxides. Chem Mater. 2005;17:1969–1977.
- Wang D-Y, Leuteritz A, Kutlu B, et al. Preparation and investigation of the combustion behavior of polypropylene/organomodified MgAl-LDH micro-nanocomposite. J Alloys Compd. 2011;509:3497–3501.
- Marangoni R, Ramos LP, Wypych F. New multifunctional materials obtained by the intercalation of anionic dyes into layered zinc hydroxide nitrate followed by dispersion into poly(vinyl alcohol) (PVA). J Colloid Interface Sci. 2009;330:303–309.
- Bruce WD, O'Hare D. Inorganic materials. New York: Wiley; 1993.
- Jung HS, Lee J-K, Kim J-Y, et al. Crystallization behaviors of nanosized MgO particles from magnesium alkoxides. J Colloid Interface Sci. 2003;259:127–132.
- Štengl V, Bakardjieva S, Mařı´ková M, et al. Magnesium oxide nanoparticles prepared by ultrasound enhanced hydrolysis of Mg-alkoxides. Mater Lett. 2003;57:3998–4003.
- Schouten N, van der Ham LGJ, Euverink G-JW, et al. Optimization of layered double hydroxide stability and adsorption capacity for anionic surfactants. Adsorption. 2007;13:523–532.
- Latip AFA, Hussein MZ, Stanslas J, et al. Release behavior and toxicity profiles towards A549 cell lines of ciprofloxacin from its layered zinc hydroxide intercalation compound. Chem Cent J. 2013;7:119–129.
- Qian L, Zhang H. Green synthesis of chitosan-based nanofibers and their applications. Green Chem. 2010;12:1207–1214.
- Sharma M, Jeevanandam P. Synthesis of magnesium oxide particles with stacks of plates morphology. J Alloys Compd. 2011;509:7881–7885.
- Li B, He J, Evans DG, et al. Enteric-coated layered double hydroxides as a controlled release drug delivery system. Int J Pharm. 2004;287:89–95.
- Keeney D, Olson RA. Sources of nitrate to ground water. Crit Rev Environ Sci Technol. 1986;16:257–304.
- Canter LW. Nitrates in groundwater. Boca Raton (FL): CRC Press; 1996.
- Ahmad AR, Zulkefli M, Ahmed M, et al. Environmental impact of agricultural inorganic pollution on groundwater resources of the Kelantan Plain, Malaysia. In: Aminuddin BY, Sharma ML, Willett IR, editors. Agricultural impacts on groundwater quality. Canberra: Australian Centre for International Agricultural Research; 1996. p. 8–21.
- Waite M. Sustainable water resources in the built environment. London: IWA Publishing; 2010. p. 13–26.
- Hussein MZ, Zainal Z, Yahaya AH, et al. Controlled release of a plant growth regulator, a-naphthaleneacetate from the lamella of Zn–Al-layered double hydroxide nanocomposite. J Control Release 2002;82:417–427.
- Sopena F, Cabreria A, Maqueda C, et al. Ethylcellulose formulations for controlled release of the herbicide alachlor in a sandy soil. J Agric Food Chem. 2007;55:8200–8205.
- Yang J, Han Y-S, Park M, et al. New inorganic-based drug delivery system of indole-3-acetic acid-layered metal hydroxide nanohybrids with controlled release rate. Chem Mater. 2007;19:2679–2685.
- Khan AI, Ragavan A, Fong B, et al. Recent developments in the use of layered double hydroxides as host materials for the storage and triggered release of functional anions. Ind Eng Chem Res. 2009;48:10196–10205.
- Sarijo SH, Hussein MZ, Yahaya AH, et al. Synthesis of phenoxyherbicides-intercalated layered double hydroxide nanohybrids and their controlled release property. Curr Nanosci. 2010;6:199–205.
- Hussein MZ, Ghotbi MY, Yahaya AH, et al. Synthesis and characterization of (zinc-layered-gallate) nanohybrid using structural memory effect. Mater Chem Phys. 2009;113:491–496.
- Costa P, Lobo JMS. Modeling and comparison of dissolution profiles. Eur J Pharm Sci. 2001;13:123–133.
- Qiu H, Lv L, Pan B, et al. Critical review in adsorption kinetic models. J Zhejiang Univ Sci A. 2009;10:716–724.
- Saifullah B, Hussein MZ, Hussein-al-ali SH, et al. Sustained release formulation of an anti-tuberculosis drug based on para-amino salicylic acid-zinc layered hydroxide nanocomposite. Chem Cent J. 2013;7:1365–1375.
- Panda HS, Srivastava R, Bahadur D. In-vitro release kinetics and stability of anticardiovascular drugs-intercalated layered double hydroxide nanohybrids. J Phys Chem B. 2009;113:15090–15100.
- Hussein SH, Al Ali, Al-Qubaisi M, et al. Comparative study of Mg/Al- and Zn/Al-layered double hydroxide-perindopril erbumine nanocomposites for inhibition of angiotensin-converting enzyme. Int J Nanomed. 2012;7:4251–4262.