ABSTRACT
The study provides insights into the silanisation process of sepiolite nanoparticles. The nanoparticles were modified applying acidic and basic silanisation methods using Methacryloxypropyltrimethoxysilane (γ-MPS) as coupling agent. The silanisation reaction was performed in the following conditions: (1) acidic ethanol–water solution with a pH of 5 and (2) basic cyclohexane with a pH of 9. The influence of the conditions on the surface chemistry of modified particles was then investigated. To characterise the surface of sepiolite nanoparticles, analytical methods such as Fourier transform infrared spectroscopy (FTIR), thermogravimetric analysis, X-Ray diffraction (XRD), scanning electron microscopy (SEM), and zeta potential analyses were applied. In addition, the colloidal stability of the modified nanoparticles was studied using a separation analysis. According to XRD and SEM, the basal interlayer distance of pristine sepiolite is not altered by silanisation process. TGA reveals that the grafting percentage of organosilane synthesised by basic method is approximately two times of that of acidic method. The silane coverage on the surface of the sepiolite and the per cent of hydroxyl groups covalently bound to γ-MPS were calculated. Separation analysis indicates decline in dispersion stability of the modified nanoparticles in ethanol due to increment of their hydrophobicity.
1. Introduction
Sepiolite is a natural hydrated magnesium silicate clay mineral with a chemical formula of Si12O30Mg8(OH)4(H2O)4.8H2O which is structurally composed of blocks and tunnels forming fibres in c-axis.[Citation1,Citation2] Two tetrahedral silica sheets and a central octahedral sheet containing magnesium form each structural block. Its surface area is generally in the range of 120–350 m2 g−1 but depending on the deposit and the history of the mineral can reach 800 m2 g−1. The single particles of sepiolite, unlike other clays, have a needle-like morphology.[Citation3,Citation4] Due to the discontinuity in the external silica layer, a significant number of silanol (Si–OH) groups exist on the surface of sepiolite. These groups can attach to different pendant functional organosilyl groups, to form some organic characteristics to the precursor inorganic support.[Citation5]
Polymer/sepiolite nanocomposites have attracted considerable interest in recent years for exhibiting improved thermal,[Citation6,Citation7] mechanical,[Citation8,Citation9] barrier,[Citation10] optical properties [Citation11] and fire retardancy.[Citation12,Citation13] The structure and morphology of sepiolite-based nanocomposites are more controllable than other layered silicate nanocomposites because of the relatively weak interactions and small contact surfaces between the sepiolite nanorods. Consequently, the dispersion of this fibrous silicate into the polymer matrix is more uniformly than those plate-like clays.[Citation14]
Surface modification of clay makes it compatible with the hydrophobic polymer matrices.[Citation15,Citation16] Clay minerals are surface-modified through different mechanisms, i.e. adsorption, ion exchange with organic/inorganic cations and grafting with organic compounds including coupling agent or polymer.[Citation11] In silanisation, the organic molecule is covalently bonded onto the clay surface via condensation between silane and clay minerals. Research on the surface modification of sepiolite has been focused on using quaternary ammonium salts (ion exchange reaction) [Citation17] or silane coupling agents,[Citation18–Citation20] especially after pioneering work of Ruiz-Hitzky,[Citation21] but less attention has been paid to the effect of reaction conditions on the silanisation process. The silane coupling agents are commonly applied on the substrate surfaces through the acidic conditions (dissolving the silane in a mixture of water/alcohol at acidic pHs) [Citation22] or basic conditions (using an anhydrous solvent in the presence of a basic catalyst, e.g. amines).[Citation23] In our work we compared these two methods.
Therefore, the main object of this study is investigating the effects of the reaction conditions on the silanisation of sepiolite nanoparticles. γ-MPS, which contains methacrylate functional group and is commonly used with (meth)acrylate or vinyl-based matrix resins,[Citation22] was used as a silane coupling agent. Sepiolite was modified through two different methods in which the pH of silanisation solution was in the range of acidic or basic values. The modified particles were characterised using FTIR, TGA (Thermogravimetric analysis), XRD, SEM (scanning electron microscopy) and zeta potential analyses. Furthermore, the dispersion stability of modified sepiolite in ethanol was studied using a separation analyser.
2. Experimental
2.1. Materials
Pristine sepiolite (Pangel S9) was kindly donated by Tolsa SA (Spain). The specific surface area and the density of silanol groups of the sepiolite are 320 m2 g−1 (BET method, N2 adsorption) and 2.2 groups per nm2, respectively.[Citation24] Methacryloxypropyltrimethoxysilane (γ-MPS, Dynalylan® MEMO) was kindly provided by Evonik (Germany). All other chemical reagents including dibutylamine, ethanol, cyclohexane and acetic acid (AA) were purchased from Merck (Germany). All materials were used as received.
2.2. Methods
2.2.1. Sepiolite treatment
Two different silanisation conditions were applied. In the acidic condition, 2.5 g of the silane coupling agent (γ-MPS) was dissolved in an ethanol/deionised water solution (water: ethanol = 30/70, w/w, pH of the solution was adjusted at 5 by acetic acid). The silane was allowed to be pre-hydrolysed in the solution for 1 h. Ten grams of sepiolite was then added to 150 g of the silane solution. After one day at room temperature, the suspension was centrifuged, and the supernatant solution was discarded. The modified sepiolite was washed and centrifuged several times with ethanol to remove unreacted silane. Resultant sepiolite was vacuum dried at 50 ºC for 72 h and ground using a ball mill (PM100, Retch, Germany).
In the basic reaction condition, 10 g sepiolite and 2.5 g silane were added to 150 g solution including cyclohexane and dibutylamine as the silanisation catalyst (dibutylamine: cyclohexane = 2:100, w/w). The silane–sepiolite suspension was allowed to react with stirring at 60 °C for 2 h then the rotary evaporator was utilised to remove solvent from the reaction mixture. The modified sepiolite was washed and centrifuged several times with cyclohexane to remove unreacted silane. Resultant sepiolite was dried at 50 ºC for 72 h and ground using the ball mill.
2.2.2. Characterisation
The pristine and silanised sepiolite were analysed by FTIR spectroscopy (EQUINOX 55, Bruker, Germany) at a resolution of 4 cm−1 and 32 scans in the range of 4000–400 cm−1 using KBr disc technique. TGAs of the pristine and treated sepiolites were performed (TGA-1500, Polymer Laboratories, UK) from room temperature to 600 °C at a heating rate of 10 °C min−1 under N2 atmosphere. X-ray diffraction patterns of pristine and silanised sepiolite were collected in the range of 2θ = 1.5°–60° and step size of 0.02°, using a Philips X-ray difractometer (Philips, X'pert, Netherlands) with copper target, λ = 1.54056 Å operating at a voltage of 40 kV and a current of 30 mA at the rate of 2 min−1. The d001 spacing was calculated according to the Bragg's equation: nλ = 2d sinθ. The morphology of pristine sepiolite and grafted sepiolites was monitored using field emission scanning electron microscopy on a Hitachi model s-4160 apparatus (Japan) at 15 kV with gold coating. The stability of dispersions was measured by a separation analyser (LUMireader®416.1, LUM, Germany) working with visible light and intensities of 75% and tilt of 0, for 24 h. 1 wt% of pristine and treated sepiolites was well dispersed in the ethanol solution by ultrasonication using a probe sonication apparatus (Sonoplus UW2200, Bandelin, Germany) in an ice bath for 15 min. To measure the zeta potential of nanoparticles and the effect of the surface treatments on the surface charge, a ZETA Sizer (Zeta plus, Brookhaven Instruments Corporation, Holtsville, NY USA) was utilised to investigate the zeta potential in different pHs (2, 3, 5 and 9). 0.03 wt% of pristine and treated sepiolites was well dispersed in a mixture of ethanol: water (50:50 wt/wt) by ultrasonication using the probe sonication apparatus in an ice bath for 15 min. The pH of suspensions was adjusted by acetic acid and NaOH.
3. Results and discussion
3.1. Fourier transform infrared spectroscopy
FTIR spectra of the pristine and sepiolite modified in acidic (AS) and basic (BS) conditions are shown in illustrating the characteristic IR absorption peaks of γ-MPS which confirms the grafting reaction. As can be seen, there are several absorption peaks attributed to the hydroxyl groups within pristine sepiolite. The 3424 and 1656 cm−1 bands are, respectively, attributed to the OH stretching vibration, indicating the zeolitic water in channels and bound water coordinated to magnesium in octahedral sheet.[Citation9,Citation25,Citation26] Low intensity bands at 3600–3700 cm−1 belong to the presence of OH groups in octahedral sheet and OH stretching vibration on the sepiolite's surface.[Citation25,Citation26] The deep bands at 1016–1018 cm−1 are observed in both pristine and treated sepiolites as a result of the stretching of Si–O in Si–O–Si groups of tetrahedral sheet demonstrating that the main structure is not altered by silanisation reactions.[Citation9,Citation25] The band at 1700–1720 cm−1 is attributed to the stretching of C = O revealing the presence of silane in the modified sepiolite. This band indicates successful silanisation of the particles surface. Aforementioned peak in the spectrum of AS is observed at 1700 cm−1 but in BS, it is located at 1720 cm−1. The shift to lower frequencies when surface was modified by the silane is caused by hydrogen bonding of the carbonyl group with substrate hydroxyls or adsorbed organosilanols.[Citation27–Citation29] Therefore, the bands at 1720 and 1700 cm−1 are assigned to non-hydrogen and hydrogen-bonded stretching vibration of C = O, respectively ().[Citation27]
Figure 1. FTIR spectra of pristine sepiolite (PS), modified sepiolite by acidic method (AS) and basic method (BS).
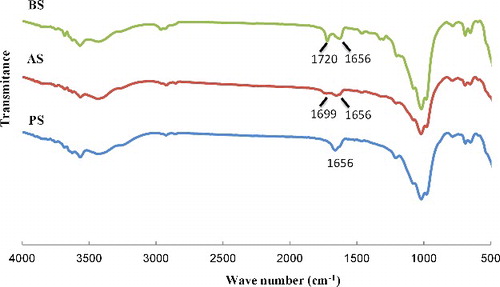
Figure 2. Molecular structure and the corresponding carbonyl FTIR peaks of monomolecular γ-MPS molecules.
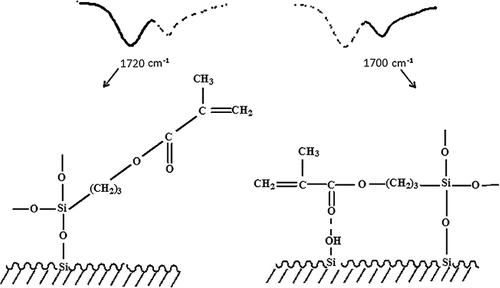
Hydrogen bonding will occur between the carbonyl and the silanol functionality of neighbouring molecules and is a function of concentration, extent of condensation and pH. The silane, which is located parallel with the surface due to hydrogen bonding,[Citation27] occupies more space comparing with the non-hydrogen bonding molecules. Taking into account that sepiolite has the same surface area in both modification methods, it can be concluded that in acidic method, the surface of sepiolite must have fewer number of silane agents.
3.2. Thermogravimetric analyses
Thermogravimetric analysis (TGA) was performed to measure the content of silanes grafted on the sepiolite surface. displays thermogravimetric curves of pristine sepiolite and organophilic sepiolites (AS and BS). The TGA curve of γ-MPS volatilisation is also shown in . The weight losses of AS and BS are about 19.2% and 21.6%, respectively, while the weight loss of the pristine sepiolite is 14%. The weight losses in the pristine sepiolite may be assigned to the removal of surface-adsorbed water, zeolitic water, coordinated water and hydroxyl group.[Citation30] Three discrete weight losses are observed in the thermogravimetric curve of pristine sepiolite (). The first weight loss up to 120 °C is related to the vaporisation of the adsorbed and zeolitic water. The elimination of coordinated water takes place in two steps.[Citation31–Citation33] Half of the coordinated water is lost between 120 and 390 °C with a 3.3% weight loss and second step of the coordinated water removal is approximately completed at 600 °C and causes a 2.7% weight loss. The total weight loss up to 600 °C is 14 wt%. As shown in , the grafted sepiolites lose lower amounts of zeolitic water than pristine sepiolite (PS): 2.8 wt% for BS and 6.8 wt% for AS, comparing with 8.3 wt% in the case of PS. Therefore, the modified sepiolite through basic process is more hydrophobic than the sepiolite silanised in acidic condition. This is due to the lower adsorption of water because of the induced hydrophobicity of sepiolite surface by the grafted organosilane and the partial replacement of zeolitic water.[Citation34] displays the TGA curve of γ-MPS which indicates that the molecule vaporises up to 200 °C. This suggests that the weight loss at ca. 200 °C may also be, in part, the result of evaporation of physically adsorbed silane.[Citation35] The extent of silane grafted on sepiolite particles can, however, be calculated considering the removal of zeolitic water (measured from the first weight loss step) and of coordinated and structural water in the sepiolite (6%). The total weight losses of AS and BS are about 19.2% and 21.6%, respectively. In the case of AS, the amount of grafted silane obtained is 6.6% and for BS is 12.8%. The results revealed that the grafting percentage in the case of BS is approximately two times of that of AS. The number of γ-MPS which reacted with silanol groups at the surface of sepiolite is calculated taking into account the molecular weight of the silane (MW = 248.35).
The numbers of γ-MPS grafted on the surface of the sepiolite are 5 × 1017 and 9.7 × 1017 per square meter, respectively, for AS and BS. Considering the concentration of surface silanols on sepiolite (2.2 groups per nm2), and assuming that each γ-MPS is reacted with one silanol group, the per cent of hydroxyl groups covalently bound to γ-MPS is calculated as 44% for BS and 23% for AS. The results also solidify the assumption of parallel arrangement of the silane molecules with the sepiolite surface in the acidic condition.
3.3. X-Ray diffraction analyses
shows XRD patterns of the sepiolites before and after modification, which indicates that the crystallinity of the mineral lattice is preserved. The most intense reflection of sepiolite (110) is seen at 2θ = 8.42° (corresponding to 1.21 nm d-value). After treatment, the patterns of organo-modified samples do not show any significant difference as compared to pattern of the pristine sepiolite which means that the crystal structure of sepiolite has been persisted during the process.[Citation14,Citation36] The d-value of the sepiolite is still 1.2 nm because sepiolite is a non-swelling clay which cannot expand like other clays such as montmorillonite. This feature probably indicates that organophilisation occurs mainly through a surface modification or by partial replacement of zeolitic water, maintaining its crystalline structure.[Citation34,Citation37]
3.4. Scanning electron microscopy
The morphology of the sepiolite particles was analysed in SEM. SEM analyses () indicate that after grafting of sepiolite by two methods, sepiolite keeps its natural fibrous morphology and no clear increase in the distance between rods is observed which confirms XRD results. These observations are in accordance with XRD analysis. It seems that the silane coupling agents mainly react with the large number of silanol groups available on the surface of the sepiolite particles.
3.5. Separation analysis
displays sedimentation behaviour of pristine and treated sepiolite particles in ethanol solution. The dispersion analysis of pristine and the modified sepiolites reveal a decrease in sedimentation time for the modified sepiolites in ethanol as a solvent. Concentrations of particles in the solvent were the same. Pristine sepiolite shows the longest required time for sedimentation and BA exhibits the shortest. The transmission of any of samples did not reach 100% during 24 h. The highest transmission is seen for BS during determined time. The rate of sedimentation depends on density and surface chemistry of particles and becomes low if particles have a good interaction with solvent.[Citation38] According to XRD and SEM observation, after the modification of sepiolite, the space between rods of sepiolite has not been wider compared with pristine sepiolite which means that the nanoclay fibrrs do not separate from each other. The grafting of sepiolite, however, changes the surface chemistry of the particles and increases their hydrophobicity. In a polar solvent (here ethanol) the surface-treated particles tend to agglomerate resulting in clusters of particles which sediment at a higher rate. The dispersion stability of pristine sepiolite in ethanol is higher than both treated sepiolite which indicates that interaction between untreated particles with the solvent is stronger compared with the treated ones. Visual observation of the sedimentation behaviour () also confirms the higher stability of the dispersion containing unmodified nanoparticles.
3.6. Zeta potential
The surface charge on treated and pristine particles was measured by zeta potential analysis. indicates that the pristine sepiolite has pHIEP at 2.7 similar to the treated sepiolites but totally the surfaces of treated sepiolites become more negative at pHs higher than pHIEP comparing to the untreated sepiolite. The zeta potential is utilised to study the potential of solid at the shear plane to characterise its electrokinetic behaviour, because the surface potential is not directly measurable.[Citation38] Different parameters such as pH of the liquid phase or electrolyte concentration cause changes in electrokinetic results. The results reveal that the pristine sepiolite has an isoelectric point (IEP) at pH 2.7. Therefore, in pH values lower than 2.7, the surface charges become positive, while it is negatively charged above pH 2.7. The dependence of zeta potential on suspension pH for γ-MPS is shown in . When the surface of a solid is attached with a group bearing a dissociable basic molecule, pHIEP of the solid is expected to shift to basic region.[Citation25] γ-MPS does not have any acidic or basic separable part so, no significant change is observed in the IEP) of the treated and untreated particles. The BS, however, shows slightly lower zeta potential (more negative) in pHs higher than pHIEP. Higher pHs lead to an increase in the number of hydroxyl groups present in the solvent which may consequently induce polarisation of the particles surface functional groups (the carbonyl groups of silane and OH groups of the particles) resulting in the higher total negative charge in the system.
4. Conclusion
In this study, sepiolite nanorods were surface-modified with γ-MPS applying two different conditions (acidic and basic). The surface chemistry of treated particles was studied using different analytical techniques. It is concluded that basic silanisation method results in higher grafting because of the alignment of the silane coupling agent perpendicular to the surface. According to FTIR and TGA results it is hypothesised that in the acidic condition the silane coupling agent is located parallel with the surface due to hydrogen bonding. As clearly shown by XRD diffraction patterns, sepiolite remains unchanged in the crystal structure. Separation analysis indicates that modified sepiolites have lower dispersion stability in ethanol as a polar solvent than pristine sepiolite. According to zeta potential measurements, γ-MPS behaves as a nonpolar coupling agent causing no significant change in the IEP of the sepiolite.
Disclosure statement
No potential conflict of interest was reported by the authors.
References
- Ruiz-Hitzky E. Molecular access to intracrystalline tunnels of sepiolite. J Mater Chem. 2001;11:86–91.
- Lemić J, Tomašević-Čanović M, Duričić M, et al. Surface modification of sepiolite with quaternary amines. J Colloid Interface Sci. 2005;292:11–19.
- Kuang W, Facey G, Detellier C, et al. Nanostructured hybrid materials formed by sequestration of pyridine molecules in the tunnels of sepiolite. Chem Mater. 2003;15:4956–4967.
- Belzunce MJ, Mendioroz S, Haber J. Modification of sepiolite by treatment with fluorides: structural and textural changes. Clays Clay Miner. 1998;46:603–614.
- Tekin N, Dinçer A, Demirbaş Ö, et al. Adsorption of cationic polyacrylamide onto sepiolite. J Hazard Mater. 2006;134:211–219.
- Tabatabaei-Yazdi Z, Mehdipour-Ataei S. Poly (ether-imide) and related sepiolite nanocomposites: investigation of physical, thermal, and mechanical properties. Polym Adv Technol. 2015;26:308–314.
- Abbasi A, Mehdipour-Ataei S. Novel sepiolite-based poly (amide-imide) nanocomposites: preparation and properties. Polym-Plast Technol Eng. 2014;53:596–603.
- Sárossy Z, Blomfeldt T, Hedenqvist M, et al. Composite films of arabinoxylan and fibrous sepiolite: morphological, mechanical, and barrier properties. ACS Appl Mater Interfaces. 2012;4:3378–3386.
- Frydrych M, Wan C, Stengler R, et al. Structure and mechanical properties of gelatin/sepiolite nanocomposite foams. J Mater Chem. 2011;21:9103–9111.
- Cho HJ, Park S, Ha CS. Preparation and characterization of polynorbornene/sepiolite hybrid nanocomposite films. Polym Int. 2015;64:96–104.
- Turhan Y, Doǧan M, Alkan M. Characterization and some properties of poly (vinyl chloride)/sepiolite nanocomposites. Adv Polym Technol. 2013;32:E65–E82.
- Cheraghi Bidsorkhi H, Soheilmoghaddam M, Heidar Pour R, et al. Mechanical, thermal and flammability properties of ethylene-vinyl acetate (EVA)/sepiolite nanocomposites. Polym Test. 2014;37:117–122.
- Liu Y, Zhao J, Deng C, et al. Flame-retardant effect of sepiolite on an intumescent flame-retardant polypropylene system. Ind Eng Chem Res. 2011;50:2047–2054.
- Liu M, Pu M, Ma H. Preparation, structure and thermal properties of polylactide/sepiolite nanocomposites with and without organic modifiers. Compos Sci Technol. 2012;72:1508–1514.
- Lu H, Shen H, Song Z, et al. Rod-like silicate-epoxy nanocomposites. Macromol Rapid Commun. 2005;26:1445–1450.
- Zhu L, Wool RP. Nanoclay reinforced bio-based elastomers: synthesis and characterization. Polymer. 2006;47:8106–8115.
- Yildiz A, Gür A. Adsorption of phenol and chlorophenols on pure and modified sepiolite. J Serb Chem Soc. 2007;72:467–474.
- García N, Guzmán J, Benito E, et al. Surface modification of sepiolite in aqueous gels by using methoxysilanes and its impact on the nanofiber dispersion ability. Langmuir. 2011;27:3952–3959.
- Shen W, He H, Zhu J, et al. Grafting of montmorillonite with different functional silanes via two different reaction systems. J Colloid Interface Sci. 2007;313:268–273.
- Demirbaş Ö, Alkan M, Doǧan M, et al. Electrokinetic and adsorption properties of sepiolite modified by 3-aminopropyltriethoxysilane. J Hazard Mater. 2007;149:650–656.
- Ruiz-Hitzky E, Serratosa JM. Adsorption of monovalent organic cations on sepiolite: experimental results and model calculations. Clays Clay Miner. 1998;46:340–348.
- Atai M, Pahlavan A, Moin N. Nano-porous thermally sintered nano silica as novel fillers for dental composites. Dent Mater. 2012;28:133–45.
- Antonucci J, Dickens S, Xu H, et al. Chemistry of silanes: interfaces in dental polymers and composites. J Res Natl Inst Stand Technol. 2005;110:541.
- Tartaglione G, Tabuani D, Camino G, et al. PP and PBT composites filled with sepiolite: morphology and thermal behaviour. Compos Sci Technol. 2008;68:451–460.
- Alkan M, Tekin G, Namli H. FTIR and zeta potential measurements of sepiolite treated with some organosilanes. Microporous Mesoporous Mater. 2005;84:75–83.
- Darder M, López-Blanco M, Aranda P, et al. Microfibrous chitosan-sepiolite nanocomposites. Chem Mater. 2006;18:1602–1610.
- Miller JD, Ishida H. Quantitative monomolecular coverage of inorganic particulates by methacryl-functional silanes. Surf Sci. 1984;148:601–622.
- Ishida H, Koenig JL. Fourier transform infrared spectroscopic study of the structure of silane coupling agent on E-glass fiber. J Colloid Interface Sci. 1978;64:565–576.
- Herrera NN, Letoffe J, Reymondc J, et al. Silylation of laponite clay particles with monofunctional and trifunctional vinyl alkoxysilanes. J Mater Chem. 2005;15:863–871.
- Chen H, Lu H, Zhou Y, et al. Study on thermal properties of polyurethane nanocomposites based on organo-sepiolite. Polym Degrad Stab. 2012;97:242–247.
- Nagata H. On dehydration of bound water of sepiolite. Clays Clay Miner. 1974;22:285–293.
- Ruiz R, Moral J, Pesquera C, et al. Reversible folding in sepiolite: study by thermal and textural analysis. Thermochim Acta. 1996;279:103–110.
- Frost RL, Kristóf J, Horváth E. Controlled rate thermal analysis of sepiolite. J Therm Anal Calorim. 2009;98:423–428.
- Tartaglione G, Tabuani D, Camino G. Thermal and morphological characterisation of organically modified sepiolite. Microporous Mesoporous Mater. 2008;107:161–168.
- Frost RL, Ding Z. Controlled rate thermal analysis and differential scanning calorimetry of sepiolites and palygorskites. Thermochim Acta. 2003;397:119–128.
- Acosta J, Ojeda M, Morales E, et al. Morphological, structural, and interfacial changes produced in composites on the basis of polypropylene and surface‐treated sepiolite with organic acids. III. Isothermal and nonisothermal crystallization. J Appl Polym Sci. 1986;32:4119–4126.
- Benlikaya R, Alkan M, Kaya İ. Preparation and characterization of sepiolite-poly (ethyl methacrylate) and poly (2-hydroxyethyl methacrylate) nanocomposites. Polym Compos. 2009;30:1585–1594.
- Rostami M, Mohseni M, Ranjbar Z. Investigating the effect of pH on the surface chemistry of an amino silane treated nano silica. Pigm Resin Technol. 2011;40:363–373.