Abstract
ZnO@SiO2-TTIP was synthesised as a novel heterogeneous catalyst and characterised by FT-IR, XRD, TEM, SEM and N2 adsorption-desorption. In this catalytic system, TTIP acts as a dopant agent for ZnO@SiO2 to decreases the recombination of photogenerated electron/hole pairs and improves the catalyst activity. The catalyst was successfully utilised for the synthesis of 2-substituted benzimidazoles and benzothiazoles from 1,2-phenylenediamine and 2-aminothiophenol with wide variety of aromatic aldehydes.
1. Introduction
In general, the synthesis of core/shell structured material has the goal of obtaining a new composite material with synergetic or complementary behaviours between the core and shell materials. Many studies on the synthesis of composites, i.e. TiO2, [Citation1] Au, [Citation2] γ-Fe2O3 [Citation3] and Ag [Citation4] coated with SiO2 shells, have been reported. SiO2 is a most studied shell candidate due to its relative ease in preparation, good environmental stability and compatibility with other materials, which motivated us to prepare the core/shell structured composite of ZnO and SiO2 and expected to achieve novel properties resulting from the synergic interaction of these two chemical components.
Transition-metal catalysed organic transformations are often carried out to follow the principles of ‘Green Chemistry’ due to minimisation of waste, cleaner auxiliaries, catalysts or reagents and minimum use of energy [Citation5–8].
Nanocatalysts are important as a bridge between heterogeneous and homogeneous catalysts [Citation9]. One of the interesting properties of nanomaterials is that they have a high specific surface area of the active part, leading to an increase in contact with the reactants [Citation10]. Moreover, a higher surface area gives the nanomaterials a more active surface; they are hard to be separated. Therefore, it is attractive to design a recoverable and well-dispersed catalyst.
Recently, much effort has been devoted to study ZnO as a very promising photocatalyst for photocatalytic degradation of water pollutants, owing to its high activity, low cost and environmentally friendly feature [Citation11,Citation12].
Benzothiazoles and benzimidazoles moieties found in various natural products and belong to one of the most important classes of heterocycles with biologically and pharmaceutically activities [Citation13,Citation14].
Until now, a number of methods have been developed for the synthesis of benzimidazoles and bezothiazoles [Citation15–20].
To the best of our knowledge, most of the procedure for the synthesis of benzimidazoles and benzothiazoles suffer from one or some disadvantages such as low yields, harsh reaction conditions, time consuming process, use of expensive catalysts and boring workups. Thus presently, the development of environmentally benign, high-yielding and fast synthesis of benzimidazole and benzothiazole derivatives remains a desired goal in organic synthesis. In this work, we have elaborated core/shell structured ZnO@SiO2-TTIP via a facile chemical route for the preparation of 2-substituted benzimidazole and benzothiazole derivatives by using of H2O2 in ethanol (EtOH) media (Scheme 1).
2. Results and discussion
2.1. Catalyst characterization
A comparison between the FTIR spectra of the ZnO nanoparticles (a), ZnO@SiO2 core-shell (b) and ZnO@SiO2-TTIP (c) are shown in . The FTIR spectrum of the ZnO nanoparticles showed a peak at 495 cm−1 that is the characteristic absorption of Zn–O bond and the peaks at 3443 and 1628 cm−1 can be ascribed to the absorption of water. The band at 1069 cm−1 was assigned to the asymmetric Si–O–Si stretching vibration as well as the band appeared at 797 cm−1 was due to the Si-O-Si bending vibrations in ZnO@SiO2 and ZnO@SiO2-TTIP [Citation21]. In the case of ZnO@SiO2, some bands belonged to the stretching vibrations of C–H bonds are appeared in the range 2860–2962 cm−1 which confirms the presence of alkoxy or alkyl chains, directly bonded to the metal atoms. The bands typical of isopropoxy groups bonded to the surface of ZnO@SiO2 appeared at 1450 cm−1and 1399 cm−1. The band at 970 cm−1 attributed to the antisymmetric Ti–O–Si stretching vibration [Citation22]. The phase and crystal structure of the samples was identified by XRD. shows the XRD patterns of the ZnO (a), ZnO@SiO2 (b) and ZnO@SiO2-TTIP (c).
The diffraction peaks appearing in the vicinity of 2θ ≈ 32°, 34.5°, 36.5°, 47.9°, 56.8°, 63.0°, 66.5°, 68°, 69.5°, 73.0° and 77.0° can be indexed to (100), (002), (101), (102), (110), (103), (200), (112), (201), (004), crystal faces of wurtzite ZnO with P63mc space group and hexagonal structure (JCPDS No. 80-0075). Modification of ZnO nanoparticle with a thin layer of SiO2 () shell does not influence the crystalline structure of ZnO and consequently the characteristic peaks of ZnO nanoparticles are still in consistence with the zincite phase of ZnO. The characteristic diffraction peaks of amorphous SiO2 were not obviously detected due to the small amount of SiO2 [23]. Similar result obtained for CaCO3@SiO2 core–shell nanoparticles that no peaks of SiO2 shell in XRD spectra was observed [Citation24]. After grafting the TTIP on the surface of ZnO@SiO2, the XRD pattern does not change indicating that the ZnO@SiO2 was properly retained after the grafting of TTIP. Some similar results are reported for grafting the TTIP on the surface of silica and showed that the XRD of silica does not change after grafting process [Citation25,Citation26].
In order to investigate the particle size and morphology of ZnO@SiO2, SEM and TEM images of ZnO@SiO2 were presented in and . By SEM images some data about the morphology and size of catalyst particles were obtained (). This results show that ZnO@SiO2 nanoparticles were obtained with an average diameter of 59–91 nm.
Textural properties of ZnO@SiO2-TTIP were evaluated by N2 sorption-desorption analysis and pore size distribution was calculated by Barret-Joyner-Halenda (BJH) method. N2 Sorption-desorption isotherm is categorised to type IV (), representative of mesoporous materials with an H2 hytersis loop which indicates a narrow pore-size distribution. BET surface area of ZnO@SiO2-TTIP is 122.4 m2.g−1. Pore size distribution curve shows a maximum pore width at 2.1 nm. The larger BET surface area enhances the activity of catalyst, which is consistent with the observed results.
Figure 5. N2 adsorption-desorption isotherms of ZnO@SiO2-TTIP at 77 K. The pore size distribution curve using BJH method is shown in the inset.
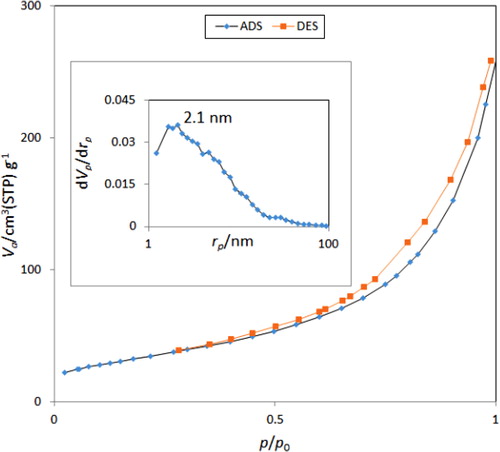
In the next step, the catalytic activity of ZnO@SiO2-TTIP on the preparation of benzimidazole and benzothiazole derivatives was studied. To optimise the reaction conditions, the effect of different amounts of ZnO@SiO2, TTIP and various solvents was investigated in a model reaction between benzaldehyde and 1,2-phenylenediamine ().
Table 1. Effect of increasing amount of ZnO@SiO2-TTIP on the preparation of 2-substituted benzimidazole derivatives in various solvents.Table Footnotea
The reactions were carried out in the presence of 0, 0.025 and 0.03 g of ZnO@SiO2 and 0, 0.6 and 0.9 mmol of TTIP. As shown (entry 1), although a mixture of benzaldehyde, 1,2-phenylenediamine and 2 mmol of 30% H2O2 mixed together at room temprature, only trace amounts of corresponding product ware formed even after 8 hours and imine intermediate was the major product. By using 0.025 g of ZnO@SiO2, 0.6 mmol of TTIP and 2 mmol of H2O2 the best results regarding the reaction time and yield was obtained (entry 5). Using lower amount of catalyst resulted in lower yield (entry 4), while higher amount did not affect the reaction time and yield appreciably (entry 9). The effect of other solvents such as CH3CN, H2O, CH2Cl2 was investigated under optimum reaction conditions (entries 6–8). It was found that the nature of solvent has a considerable effect on the reaction rate and the yield. However, ethanol was found to be the best solvent and the other solvents were substantially less effective. To emphasise that the ZnO@SiO2-TTIP is superior over ZnO nanoparticles and ZnO-TTIP composite we performed the model reaction using ZnO nanoparticles (0.6 mmol, 0.048 g) and ZnO-TTIP composite (0.6 mmol, 0.48 g ZnO, 0.6 mmol, 0.2 mL TTIP). The desired product was obtained with the yields of 35% and 70% for ZnO nanoparticles and ZnO-TTIP respectively after 10 min. It is obvious that ZnO@SiO2-TTIP demonstrates better catalytic activity relative to ZnO nanoparticles, TTIP and ZnO-TTIP. Encapsulation of ZnO nanoparticles into a layer of silica shell prohibit the agglomeration problem [Citation27]. It is well documented that ZnO nanoparticles have a toxic nature [Citation28]. ZnO nanoparticles dissolve in aqueous solutions to release Zn2+ ion. The influx of Zn2+ ion could disturb the natural function of cells [Citation29]. Surface modification of nanoparticles in most cases alters the intrinsic properties of ZnO nanoparticles [Citation30] rather than reduce their toxicity. Therefore, we covered the ZnO nanoparticles with a thin layer of silica to relieve the releasing of Zn2+ ion and decrease the toxic nature of ZnO without change in the intrinsic properties of ZnO nanoparticles owing to the fact that the silica layer is benign and inert. Since the electronegativity of Si (∼1.9) is higher than that of Zn (∼1.65), the valence electron density of Zn in the Zn–O–Si bond is lower than that in the Zn–O–Zn bond [Citation31], therefore, Zn in the core of ZnO@SiO2 demonstrates more electron affinity. ZnO is a wide band gap n-type semiconductor (Eg = 3.37 eV) and suffers from two important drawbacks; first, with the large band gap of 3.37 eV; the wavelengths below 400 nm are necessary for excitation that are in the range of ultraviolet. Another problem of ZnO is the quick charge carrier recombination of photogenerated electron/hole pairs and low photocatalytic activity [Citation32,Citation33]. One of the best methods to decrease the recombination of photogenerated electron/hole pairs is to modify the surface of ZnO by metal doping into the ZnO nanoparticles. By collecting the photogenerated electrons from the conduction band of semiconductor, the dopant acts as a trap, increases the charge separation and consequently hampers the electron/hole pair recombination and finally improves the photocatalytic activity in the visible light [Citation33]. In the present work, TTIP acts as a dopant agent. Due to the smaller ionic radii of Ti4+ (42 pm) compared to Zn2+ (60 pm), the substitution of Zn2+ with Ti4+ occurs, generates the point defects and decreases the electron/hole pair recombination [Citation34]. As a result, the doping of ZnO core in ZnO@SiO2 facilitates the formation of OH radicals from H2O2.
After optimisation of the reaction conditions, synthesis of a variety of functionalised benzimidazoles was performed to explore the efficiency and the scope of the protocol. The corresponding results have been depicted in . As shown, both aldehydes bearing electron-donating and electron-withdrawing substituents gave desired benzimidazoles in excellent yields. In addition, heterocyclic aldehydes could also be used and worked well in this reaction (, entries 13 and 14). In order to further demonstrate the power and efficiency of this catalytic system, the synthesis of benzothiazoles was investigated in the presence of prepared catalyst. The experimental results showed, treatment of a variety of aldehydes with 2-aminothiophenol in the presence of catalytic amount of ZnO@SiO2-TTIP at room temperature afforded the corresponding 2-substituted benzothiazoles in (92–97%) yields (). Also, to show the efficiency of this method, a comparison of the efficiency of this method with selected previously methods is collected in . The results show that this method is comparable to some previously reported methods in terms of reaction times, temperatures and yields.
Table 2. Direct synthesis of 2-substituted benzimidazoles.Table Footnotea
Table 3. Direct synthesis of 2-substituted benzothiazoles.Table Footnotea
Table 4. Comparison of methods for the synthesis of benzimidazoles and benzothiazoles.
In another step, recyclability of ZnO@SiO2-TTIP was studied. For this purpose, the reaction of 1,2-phenylenediamine and benzaldehyde was selected in the optimised reaction conditions. As shown in , catalytic activity of ZnO@SiO2-TTIP was restored within the limits of the experimental errors for 7 successive recycle runs. However, the little decrease in yield was associated with the blocked pores during the course of reaction.
As discussed, doping ZnO@SiO2 with TTIP decreases the recombination of photogenerated electron/hole pairs and forms visible light photocatalytic activity; therefore, no UV-visible light is needed for irridation. We propose that in the first step a complex is formed between H2O2 and Ti-doped ZnO (ZnO@SiO2-TTIP) followed by homolytic cleavage of O-O bond of H2O2 to generate OH radicals. Generation of OH radical by coordination of H2O2 on the surface of other metal oxides is reported [Citation43]. The possible mechanism for this synthesis is shown in Scheme 2. Before addition of H2O2, aldehyde was activated by forming a coordinate bond with the Lewis acid site of a ZnO@SiO2-TTIP surface. Nucleophilic attack by 1,2-phenylenediamine on the aldehyde gives intermediate (I) which exist in equilibrium with the cyclic hydrobenzimidazoles (II). Then, the oxidation may be launched by a single electron transfer from hydroxyl to the intermediate (II) to produce hydroxide ion and a radical cation III that subsequently loses a proton to yield a radical IV which in turn is attacked by hydroxyl radical to afford product in the reaction mixture. The additional hydrogen peroxide then undergoes partial decomposition to O2 and H2O. To confirm that the reaction proceeds with formation of free radicals, a reaction between 1,2-phenylenediamine and benzaldehyde was performed in the presence of a radical scavenger, tert-butanol, affording trace amounts of product. This implies the involvement of free radicals in the reaction process.
3. Experimental
All chemicals were purchased from Merck or Fluka Chemical Companies. The known products were identified by comparison of their melting points and spectral data with those reported in the literature. Infra-red (IR) spectra were recorded on a Bruker FT-IR WQF-510 spectrophotometer in KBr with absorption in centimeters−1. 1H and 13 C NMR spectra were recorded on a Bruker (200 MHz) spectrometer in CDCl3 or DMSO as a solvent. The morphology of ZnO@SiO2 was investigated by scanning electron microscopy (SEM) using a TESCAN MIRA ∏ instrument. Transmission electron microscopy (TEM) measurements were carried out on a Philips CM120 1988. X-ray powder diffraction patterns were obtained on a SE1FERT-3003TT. BET analysis was carried out on a BELOSORP mini Π Czech.
3.1. General procedure for the preparation of ZnO@SiO2-TTIP
Typical steps were given as follows: ZnO nanoparticles were dispersed into 50 mL of EtOH and then slowly transferred into the 500 mL round bottom flask. An appropriate amount of TEOS together with 50 mL of EtOH, 75 mL H2O and 25 mL NH3.H2O (35%) was then added into the reaction flask. The mixture containing the ZnO, TEOS, solvent and NH3.H2O was stirred for 4 h. Products were collected by centrifugation, subsequently washed with EtOH for three times, and then dried in a vacuum oven at 160 °C for 2 h. Then titanium isopropoxide (TTIP) (0.6 mmol, 0.2 mL) was injected to ZnO/SiO2 nanoparticles to produce ZnO@SiO2-TTIP. Therefore, we performed the doping of ZnO@SiO2 in the reaction mixture using an in situ method. Catalytic properties were reduced if ZnO@SiO2 refluxed directly with TTIP since in this procedure we need Ti4+ to substitute with Zn2+. Therefore, in this study, TTIP was injected to reaction mixture.
3.2. General procedure for the synthesis of 2-substituted benzimidazoles and benzothiazoles
To a premixed solution of ZnO@SiO2 (0.025 g) and TTIP (0.6 mmol, 0.2 mL) in EtOH (6 mL) was added 1,2-phenylenediamine (1 mmol, 0.1 g), aryl aldehyde (1 mmol) and 30% H2O2 (2 mmol, 0.2 mL); then, the mixture was stirred at room temperature. The progress of the reaction was monitored by TLC to determine the necessary reaction times (). After completion of the reaction, the catalyst was removed by filtration and was reused in the next run. The filtrate was poured into ethyl acetate and n-hexane. The pure solid product was filtered and dried to produce 2-substituted benzimidazole derivative in excellent yield. An identical procedure was employed using 2-aminothiophenol (1 mmol, 0.1 mL), aryl aldehyde (1 mmol) in the presence of 30% H2O2 (2 mmol, 0.2 mL), ZnO@SiO2 (0.025 g) and TTIP (0.6 mmol, 0.2 mL) for the synthesis of 2-substituted benzothiazoles ().
4. Conclusion
In summary, we have synthesised ZnO@SiO2-TTIP as a novel and nanostructured heterogeneous catalyst, and fully characterised by several techniques including FTIR, XRD, SEM, TEM and N2 sorption-desorption. High BET surface area and decrease in the electron/hole pair recombination made the as-prepared catalyst highly reactive toward synthesis of 2-substituted benzimidazoles and benzothiazoles. Short reaction times, high yields, mild reaction condition and reusability of the catalyst are some advantages of this applied work.
Acknowledgments
We are thankful to the Razi University Research Council for partial support to this work.
Disclosure statement
No potential conflict of interest was reported by the authors.
Additional information
Notes on contributors
Kiumars Bahrami
Dr. Kiumars Bahrami is working as a Professor of organic chemistry in faculty of chemistry, Razi university, Kermanshah, Iran. He has many papers in national and international journals. He has also participated and presented several papers at national and international conferences. To date, he has supervised 30 M.Sc. and 10 Ph.D. students. He is developing research programs in the areas of nanostructured materials in organic syntheses.
Zahra Karami
Zahra Karami is a M.Sc. student under the guidance of Dr. Kiumars Bahrami at faculty of chemistry, Razi university, Kermanshah, Iran. She has participated in national conferences. Her interest covers application of nanocatalysts in organic reactions.
References
- Sun J, Xu K, Shi C, et al. Influence of core/shell TiO2@SiO2 nanoparticles on cement hydration. Constr Build Mater. 2017;156:114–122.
- Ryabchikova EI, Chelobanov BP, Parkhomenko RG, et al. Degradation of core-shell Au@SiO2 nanoparticles in biological media. Microporous Mesoporous Mater. 2017;248:46–53.
- An GS, Choi SW, Chae DH, et al. γ-Fe2O3@SiO2 core-shell structured nanoparticle: fabrication via surface treatment and application for plasmid DNA purification. Ceram Int. 2017;43:12888–12892.
- Alimunnisa J, Ravichandran K, Meena K. Synthesis and characterization of Ag@SiO2 core-shell nanoparticles for antibacterial and environmental applications. J Mol Liq. 2017;231:281–287.
- Karami C, Mohammadi H, Ghodrati K, et al. Cobalt manganese oxide nano catalysts as a recyclable catalyst for the synthesis of 3,4-dihydropyrimidin-2(1H)-ones-thiones. Synth React Inorg Met-Org Nano-Metal Chem. 2015;45:271–276.
- Haghnazari N, Karami C, Ghodrati K, et al. Nitration of phenols with Fe(NO3)3.9H2O in the presences of nano-SiO2 as an efficient catalyst. Int Nano Lett. 2011;1:30–33.
- Karami C, Abdollahifar M, Jahani F, et al. The preparation and characterization of flowerlike boehmite nanoparticles-SA: as new and reusable nanocatalyst for the synthesis of 2-aryl-1H-benzimidazoles. Synth React Inorg Met-Org Nano-Metal Chem. 2017;47:626–631.
- Karami C, Ahmadian H, Nouri M, et al. A novel method for synthesis of cobalt manganese oxide nano catalysts as a recyclable catalyst for the synthesis of some bis (indolyl) methane derivatives. Catal Commun. 2012;27:92–96.
- Shylesh S, Schünemann V, Thiel WR. Magnetically separable nanocatalysts: bridges between homogeneous and heterogeneous catalysis. Angew Chem Int Ed. 2010;49:3428–3459.
- Pal N, Bhaumik A. Mesoporous materials: versatile supports in heterogeneous catalysis for liquid phase catalytic transformations. RSC Adv. 2015;5:24363–24391.
- Goudarzi M, Mousavi-Kamazani M, Salavati-Niasari M. Zinc oxide nanoparticles: solvent-free synthesis, characterization and application as heterogeneous nanocatalyst for photodegradation of dye from aqueous phase. J Mater Sci Mater Electron. 2017;28:8423–8428.
- Da Silva GT, Carvalho KT, Lopes OF, et al. Synthesis of ZnO nanoparticles assisted by N sources and their application in the photodegradation of organic contaminants. ChemCatChem. 2017;9:3795–3804.
- Thakkar SS, Thakor P, Ray A, et al. Benzothiazole analogues: synthesis, characterization, MO calculations with PM6 and DFT, in silico studies and in vitro antimalarial as DHFR inhibitors and antimicrobial activities. Bioorganic Med Chem Lett. 2017;25:5396–5406.
- Keri RS, Patil MR, Patil SA, et al. A comprehensive review in current developments of benzothiazole-based molecules in medicinal chemistry. Eur J Med Chem. 2015;89:207–251.
- Hornberger KR, Adjabeng GM, Dickson HD, et al. A mild, one-pot synthesis of disubstituted benzimidazoles from 2-nitroanilines. Tetrahedron Lett. 2006;47:5359–5361.
- Wang R, Lu XX, Yu XQ, et al. Acid-catalyzed solvent-free synthesis of 2-arylbenzimidazoles under microwave irradiation. J Mol Catal A Chem. 2007;266:198–201.
- Surpur MP, Singh PR, Patil SB, et al. One‐pot synthesis of benzimidazoles from o‐nitroanilines under microwaves via a reductive cyclization. Synth Commun. 2007;37:1375–1379.
- Mohammadi H, Karami C, Bahri M, et al. BiOCl/FeOCl/SiO2 nano composite as an efficacy novel catalyst toward synthesis of 2-aryl-1H-benzimidazoles in mild aerobic condition. Inorg Nano-Met Chem. 2017;47:1334–1341.
- Taher MA, Karami C, Sheikh Arabi M, et al. Efficient FeCl3/SiO2 as heterogeneous nanocatalysis for the synthesis of benzimidazoles under mild conditions. Int Nano Lett. 2016;6:85–90.
- Ahmadian H, Veisi H, Karami C, et al. Cobalt manganese oxide nanoparticles as recyclable catalyst for efficient synthesis of 2-aryl-1-arylmethyl-1H-1,3-benzimidazoles under solvent-free conditions. Appl Organometal Chem. 2015;29:266–269.
- Liden E, Bergström L, Persson M, et al. Surface modification and dispersion of silicon nitride and silicon carbide powders. J Eur Ceram Soc. 1991;7:361–368.
- Pérez Y, Del Hierro I, Zazo L, et al. The catalytic performance of metal complexes immobilized on SBA-15 in the ring opening polymerization of ε-caprolactone with different metals (Ti, Al, Zn and Mg) and immobilization procedures. Dalton Trans. 2015;44:4088–4101.
- Zhai J, Tao X, Pu Y, et al. Core/shell structured ZnO/SiO2 nanoparticles: Preparation, characterization and photocatalytic property. Appl Surf Sci. 2010;257:393–397.
- Zhang S, Li X. Synthesis and characterization of CaCO3@SiO2 core–shell nanoparticles. Powder Technol. 2004;141:75–79.
- Cruz P, Pérez Y, Del Hierro I. Titanium alkoxides immobilized on magnetic mesoporous silica nanoparticles and their characterization by solid state voltammetry techniques: application in ring opening polymerization. Microporous Mesoporous Mater. 2017;240:227–235.
- Cruz P, Pérez Y, Del Hierro I, et al. ε-Caprolactone polymerization using titanium complexes immobilized onto silica based materials functionalized with ionic liquids: insights into steric, electronic and support effects. RSC Adv. 2016;6:19723–19733.
- Grigorie AC, Muntean C, Vlase T, et al. ZnO-SiO2 based nanocomposites prepared by a modified sol-gel method. Mater Chem Phys. 2017;186:399–406.
- Chia SL, Leong DT. Reducing ZnO nanoparticles toxicity through silica coating. Heliyon. 2016;2:e00177.
- Setyawati MI, Tay CY, Leong DT. Mechanistic investigation of the biological effects of SiO2, TiO2, and ZnO nanoparticles on intestinal cells. Small. 2015;11:3458–3468.
- Liqiang J, Baiqi W, Baifu X, et al. Investigations on the surface modification of ZnO nanoparticle photocatalyst by depositing Pd. J Solid State Chem. 2004;177:4221–4227.
- Cannas C, Casu M, Lai A, et al. XRD, TEM and 29Si MAS NMR study of sol-gel ZnO-SiO2 nanocomposites. J Mater Chem. 1999;9:1765–1769.
- Mohamed R, Barakat M. Enhancement of photocatalytic activity of ZnO/SiO2 by nanosized Pt for photocatalytic degradation of phenol in wastewater. Int J Photoenergy. 2012;2012:1–8.
- Zhang X, Qin J, Xue Y, et al. Effect of aspect ratio and surface defects on the photocatalytic activity of ZnO nanorods. Sci Rep. 2014;4:4596
- Güntner AT, Pineau NJ, Chie D, et al. Selective sensing of isoprene by Ti-doped ZnO for breath diagnostics. J Mater Chem B. 2016;4:5358–5366.
- Mobinikhaledi A, Zendehdel M, Goudarzi F, et al. Nano-Ni (II)/Y zeolite catalyzed synthesis of 2-aryl-and 2-alkyl benzimidazoles under solvent-free conditions. Synth React Inorg Met Org Chem. 2016;46:1526–1531.
- Jafarpour M, Rezaeifard A, Ghahramaninezhad M, et al. Reusable α-MoO3 nanobelts catalyzes the green and heterogeneous condensation of 1,2-diamines with carbonyl compounds. New J Chem. 2013;37:2087–2095.
- Kumar B, Smita K, Cumbal L, et al. Biogenic synthesis of iron oxide nanoparticles for 2-arylbenzimidazole fabrication. J Saudi Chem Soc. 2014;18:364–369.
- Fazlinia A, Sheikh S. Preparation of 2-arylbenzimidazole derivatives using CuO nanoparticles/H2O2 system. Inorg Nano-Met Chem. 2018;48:126–130.
- Wen X, El Bakali J, Deprez-Poulain R, Deprez B. Efficient propylphosphonic anhydride (® T3P) mediated synthesis of benzothiazoles, benzoxazoles and benzimidazoles. Tetrahedron Lett. 2012;53:2440–2443.
- Karimian A, Mohammadzadeh Kakhki R, Kargar Beidokhti H. Magnetic Co‐doped NiFe2O4 nanocomposite: a heterogeneous and recyclable catalyst for the one‐pot synthesis of benzimidazoles, benzoxazoles and benzothiazoles under solvent‐free conditions. J Chin Chem Soc. 2017;64:1316–1325.
- Digwal CS, Yadav U, Sakla AP, et al. VOSO4 catalyzed highly efficient synthesis of benzimidazoles, benzothiazoles, and quinoxalines. Tetrahedron Lett. 2016;57:4012–4016.
- Kodomari M, Tamaru Y, Aoyama T. Solvent‐free synthesis of 2‐aryl and 2‐alkylbenzothiazoles on silica gel under microwave irradiation. Synth Commun. 2004;34:3029–3036.
- Lousada CM, Johansson AJ, Brinck T, et al. Mechanism of H2O2 decomposition on transition metal oxide surfaces. J Phys Chem C. 2012;116:9533–9543.