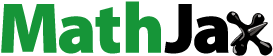
Abstract
Samarium oxychloride (SmOCl) is a promising photocatalyst for environment remediation. It is difficult to form SmOCl nanoplate by sophisticated wet chemical techniques used to prepare other layer-structured rare earth oxychloride nanoplates. Here, we report the synthesis of SmOCl nanoplates by chemical vapour deposition (CVD). Transmission electron microscopy characterization reveals that the nanoplates are single crystalline SmOCl with exposed {001} facets. Two-dimensional growth of SmOCl nanoplates is improved by adding decaborane in the atmosphere of CVD system. The largest size of SmOCl nanoplates approaches ∼5 μm. The growth process of SmOCl nanoplates is studied. This result shows that CVD is a suitable method to grow large SmOCl nanoplates.
1. Introduction
Rare earth oxychlorides (ReOCls) emerge as a new class of promising photocatalyst for environment remediation and energy conversion recently. [Citation1–3] ReOCl crystal usually stacks with tetragonal layer-structured units, in which a non-uniform charge distribution between Re2O2 slab and chlorine atom plane forms internal electric filed along the orientation perpendicular to the Re2O2 and Cl layers. This property is found to help the effective dissociation of photoinduced electron–hole pairs, leading to efficient photocatalytic performance. [Citation4] For example, BiOCl has exhibited attractive photocatalytic property comparable to the well-known TiO2 photocatalyst. [Citation1–3] Better photocatalytic efficiency is obtained by using the mixture of different rare earth oxychlorides. Comparing to pure BiOCl, a significant improvement of nitric oxide photoremoval is observed in SmOCl–BiOCl composite powders in humid environment. [Citation5] More SmOCl (∼ 80%) than BiOCl in the composite reveals the key role of SmOCl in this photoreaction process.
Controllable synthesis of rare earth oxychloride nanoplates with {001} facets remains one main task in photocatalyst field because of their facet-dependent photoactivity. For example, BiOCl nanoplates exposing (001) facet are more active for pollutant degradation than BiOCl with (010) facet. [Citation6] Common wet chemical methods used to grow ReOCl nanoplates with {001} facets, such as hydrothermal/solvothermal synthesis, anion exchange synthesis, and reversed-phase microemulsion synthesis, employ layer-structured unit of ReOCl to induce slight anisotropic growth in the solution environment. [Citation1–3] A critical factor influencing the crystal orientation of BiOCl nanoplates is the acidic environment of the solution. Exposed facet of BiOCl nanoplate changes from (001) to (010) when pH value increases from 1 to 6, because high density H+ ions act as the capping agent to keep the (001) facet in the solution. [Citation6] However, there is still no report on the formation of SmOCl nanoplate in literature, although SmOCl holds a similar layered structure as other ReOCl. How to synthesize SmOCl nanoplate is not clear up to date.
Chemical vapour deposition (CVD), a common method to grow nanomaterials via gas precursor, is usually considered to be quite distinct from the wet chemical approaches. Here, we report the synthesis of SmOCl nanoplates with exposed {001} facets by CVD. Interestingly, larger SmOCl nanoplates in the size of 3-5 μm are formed on Si/SiO2 substrate when decaborane is supplied besides samarium source. We demonstrate that the formation of SmOCl nanoplates by CVD can be improved in a gaseous ‘acidic environment’ similar to the solution environment. This work is the first report on the synthesis of SmOCl nanoplates to the best of our knowledge.
2. Experimental method
Samarium chloride hydrate powders (SmCl3⋅6H2O, ALADDIN, purity 99.99%) are used as the source in the present experiment. Cleaned small pieces of Si wafer covered with 300-nm-thick SiO2 layer are employed as the substrates. Both SmCl3⋅6H2O powders and Si/SiO2 substrate are placed in a quartz tube which is heated in a tube furnace. The source and the substrate are placed at the upstream part closing to the center of the furnace. The temperature of the tune furnace rises to 760 °C slowly and keeps 1 min at this temperature. Argon is used as the carrier gas and the quartz tube is evacuated to about 1 Torr in the heating process. Decaborane (B10H14) is used to enhance the nanoplate growth in some experiment. A small amount of decaborane are placed in a vessel separated from the entrance of the quartz tube by a valve. A heating tape is used to sublimate decaborane in the vessel when the furnace temperature approaches 760 °C.
The morphology of nanoplates is observed by a scanning electron microscope (SEM, FEI Quanta 600). The highly magnified morphology, crystal structure and the element composition are analyzed by high resolution transmission electron microscopes (HR-TEM, FEI Tecnai T20 and F30) equipped with selected area electron diffraction (SAED) and energy dispersive X-ray spectroscopy (EDAX). Atomic force microscope (AFM, DI NanoScope) is used to scan the surface of the nanoplates.
3. Results and discussion
Samarium chloride hydrate is commercially available and often used as the source to form SmOCl. Heating treatment of SmCl3⋅6H2O above 70 °C induces the loss of five H2O molecules. Pure SmCl3 cannot be obtained by heating samarium chloride hydrate at high temperature because the following reaction will occur in this case: [Citation7]
The transformation from SmCl3 to SmOCl is thermodynamically stable according to the calculated stability field diagram for Sm–O–Cl system. [Citation8] We employ this reaction to prepare SmOCl nanoplates in the present experiment.
We find that dense clusters always deposit on the substrate after heating SmCl3⋅6H2O powder for twenty minutes in a wide temperature range (< 760 °C). The general morphology of the clusters on Si/SiO2 substrate is shown in . Detailed observation reveals the irregular cluster contains some close stacked nanoplates (shown in ). The nanoplates have the thickness of less than 100 nm and the size of ∼ 0.2 μm. Such morphology seems to suggest a weak tendency of two-dimensional growth in SmOCl.
The irregular clusters in reveal too many SmOCl nuclei are formed in the growth period. Among various growth conditions, we find that the heating time at the highest heating temperature is the most important factor influencing the density of the products. When the highest temperature and the heating time are 760 °C and 1 min, isolated nanoplates are formed on the substrate. shows the nanoplates grown under the optimal condition. The nanoplates show the rounded rectangle shape and have the size of ∼ 1 μm, which is larger than that in . This result reveals if the density of SmOCl muclei is limited, isolated SmOCl nanoplates forms naturally via anisotropic two-dimensional growth in CVD environment.
Figure 2. (a) Morphology of nanoplates grown at 760 °C for 1 min, (b) TEM image of a single nanoplate, (c) high resolution TEM image, (d) SAED pattern, (e) EDAX spectrum of a nanoplate, (f) AFM image of nanoplates, (right) height plot crossing an edge of a small nanoplate.
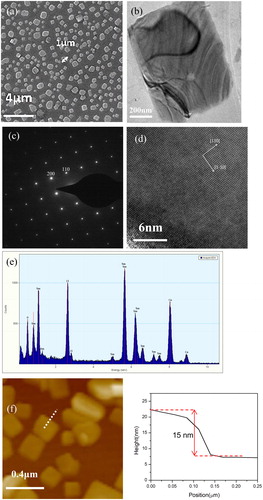
The structure of nanoplates is analyzed by TEM. shows a TEM image of a single nanoplate. The stripes in are due to the strain in thin nanoplate. shows the SAED pattern of the nanoplate, revealing the single crystalline structure of the nanoplate. The spots in are indexed to (200) and (110) planes in tetragonal structured SmOCl. High resolution TEM image of the nanoplate is shown in . (110) and (1-10) lattice planes are marked in the figure. The composition of the nanoplate is characterized by EDAX and the result is shown in . Sm, O and Cl are detected from the nanoplate. Above result reveals the nanoplate is single crystalline SmOCl with exposed {001} facets. More than twenty nanoplates are investigated by TEM and no samarium oxide or samarium chloride is found. A small SmOCl nanoplate is scanned by AFM, which gives a thickness of ∼ 15 nm (shown in ). Thicker thickness (∼ 30 nm) is measured in larger nanoplates by more AFM scanning.
Although there are numerous reports on CVD growth of two-dimensional layered materials, e.g., transition metal dichalcogenides, [Citation9, Citation10] synthesis of ReOCl nanoplates by CVD is rare in literature. [Citation11] We think the formation of SmOCl nanoplates follows the anisotropic growth of layer-structured SmOCl on the substrate. It is known that the transformation from samarium chloride hydrate to SmOCl occurs in a wide temperature range. [Citation12] In our experiments, the time for furnace temperature rising to 760 °C is usually 1 hour. We compare the powder weight before and after the temperature rising period. By subtracting the weight of H2O firstly, we find a large loss of SmCl3 after the temperature rising period. This phenomenon means that some powder is evapourated during the temperature rising period, resulting in a sparse distribution of samarium chloride hydrate on the substrate to form diverse SmOCl nuclei. Two-dimensional growth starting from these SmOCl nuclei occurs along the substrate surface afterwards. There are two questions on this growth model: where do SmOCl nuclei form and where do SmOCl nanoplates grow? A particular experiment is conducted to verify above growth model. Copper grid covered by a porous carbon film for TEM observation is used as the substrate for nanoplate growth. Two phenomena are checked: If the nuclei are formed in the gas phase, not only the holes but also the carbon film could act as their deposition sites, and if the nanoplates form in the atmosphere first and then deposit on the substrate randomly, some nanoplates may cross the small hole in the carbon film. The morphology of nanoplates on TEM grid is shown in . The nanoplates show similar morphology as those in . Each nanoplate lays on the carbon film entirely and no nanoplate crossing a hole is observed. Other than nanoplates, neither smaller nor bigger nuclei particle is found on the carbon film. This experiment confirms above growth model of nanoplates .
Acidic environment assisted anisotropic growth of BiOCl nanoplates in solution has been studied detailedly. Jiang et al. find that high density H+ ions can inhibit the growth perpendicular to (001) plane because of the strong binding interaction between H+ ions and terminated oxygen on the (001) BiOCl surface. [Citation6] Generally, it is difficult to produce enough H+ ions in common CVD system without additional power source, e.g., RF or high voltage. We try to improve the anisotropic growth of SmOCl by a simple way in CVD experiment. Decaborane is solid at room temperature but decomposes very easily under heat treatment. Hydrogen is one product in this pyrolysis reaction. [Citation13] Our system keeps the solid state of decaborane during the temperature-rising period and sublimes it to the atmosphere when needed. We find two-dimensional growth of SmOCl is promoted in this gaseous ‘acidic environment’. The morphology of nanoplates grown in the atmosphere with decaborane is shown in . It is obvious that the size of nanoplates in (∼ 3 μm) is larger than those in . We note such size of SmOCl nanoplates is also larger than almost all rare earth oxychloride nanoplates synthesized in solution. [Citation1–3] The largest nanoplate grown under this condition approaches the size of ∼ 5 μm. A thickness of ∼ 120 nm is measured in a nanoplate by AFM scanning (shown in ). In order to distinguish the possible effect of decaborane on nanoplate, more careful characterization on these nanoplates is carried out. First, the appearance of boride nanoplate in this experiment is ruled out by many times of elemental analysis, in which no trace of boron is detected within the range of the measurement error. Second, the structure of nanoplates is totally in accordance with SmOCl crystal by TEM investigation. A same single nanoplate is analyzed by TEM in two different zone axis. show the SAED pattern of the same nanoplate taken in [Citation001] and [101] zone axis. The corresponding HRTEM images are shown in , respectively. TEM characterization proves the nanoplate has the tetragonal SmOCl structure with exposed {001} facets. The effect of decaborane on the growth of larger SmOCl nanoplates has been confirmed by many times of experiments. A problem of adding decaborane in the growth atmosphere is uneven distribution of SmOCl nanoplates on the substrate. Larger nanoplates usually grow near the edge closing to the source but not evenly on the whole substrate.
Figure 4. (a) Morphology of nanoplates grown with decaborane addition, (b) AFM image of nanoplates, (right) height plot crossing an edge of a nanoplate, (c) and (e) SAED pattern, (d) and (f) high resolution TEM image of the same nanoplate in different zone axis.
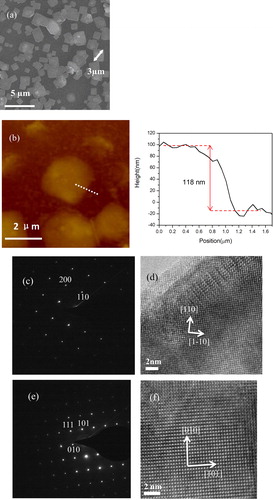
We believe the reason for improved two-dimensional growth of SmOCl is H+ ions decomposed from decaborane. Scheme 1 demonstrates the formation of SmOCl nanoplates with and without decaborane. Our method can produce H+ ions timely and ‘in situ’, which is similar to the acidic solution environment for BiOCl nanpolate growth. [Citation6] We find the time to supply decaborane is the key factor to improve the growth of the SmOCl nanoplates. The optimal time for decaborane addition is several minutes earlier than the time of the highest temperature (760 °C). If decaborane is added too early, even if more decaborane is supplied, no benefit effect is observed. This phenomenon indicates that the sublimation and pyrolysis of decaborane should occur in the same period of SmOCl nanoplate growth. According to above growth model, SmOCl nuclei are formed on the substrate during the heating period. In an atmosphere containing enough decaborane at ∼760 °C, SmOCl nuclei adsorb dense decaborane molecules on their surface. The decomposition of decaborane molecules adsorbed on the {100} facets may generate hydrogen ions at the adsorption sites, which play similar role on anisotropic growth as H+ ions in solution.
4. Conclusions
In summary, we have demonstrated a simple technique to grow SmOCl nanoplates. We show that the growth of SmOCl nanoplates in the size of ∼ 3 μm can be achieved by addition of decaborane in the growth atmosphere. TEM characterization reveals all nanoplates are single crystal SmOCl and no trace of B is detected from the nanoplates. Our work demonstrates that H+ ions decomposed from decaborane is beneficial to form large SmOCl nanoplates in CVD experiment.
Acknowledgements
This research was supported by National Natural Science Foundation of China under Grant No. 61774007.
Disclosure statement
No potential conflict of interest was reported by the authors.
References
- Li J, Yu Y, Zhang L. Bismuth oxyhalide nanomaterials: layered structures meet photocatalysis. Nanoscale. 2014;6:8473–8488.
- Cheng H, Huang B, Dai Y. Engineering BiOX (X = Cl, Br, I) nanostructures for highly efficient photocatalytic applications. Nanoscale. 2014;6:2009–2026.
- Ye L, Su Y, Jin X, et al. Recent advances in BiOX (X = Cl, Br and I) photocatalysts: synthesis, modification, facet effects and mechanisms. Environ. Sci.: Nano. 2014;1:90–112.
- Ye L, Jin X, Leng Y, et al. Synthesis of black ultrathin BiOCl nanosheets for efficient photocatalytic H2 production under visible light irradiation. J Power Sources.. 2015;293:409–415.,
- Okumura H, Adachi K, Yamasue E, et al. New LnOCl (Ln = Sm, Nd) photocatalyst and novel cocatalytic effect on BiOCl in humid environment. Chem Commun (Camb)..). 2017;53:8854
- Jiang J, Zhao K, Xiao X, et al. Synthesis and Facet-Dependent Photoreactivity of BiOCl Single-Crystalline Nanosheets. J Am Chem Soc.. 2012;134:4473–4476., pp
- Koch CW, Cunningham BB. The Vapour Phase Hydrolysis of the Rare Earth Halides. II. Heat and Free Energy of the Reactions: SmCl3(s) + H2O(g) = SmOCl(s) + 2HCl(g) and GdCl3(s) + H2O(g) = GdOCl(s) + 2HCl(g). J Am Chem Soc.. 1953;75:796–797. pp
- Jacob KT, Dixit A, Rajput A. Stability field diagrams for Ln–O–Cl systems. Bull Mater Sci.. 2016;39:603–611.
- Yu J, Li J, Zhang W, et al. Synthesis of high quality two-dimensional materials via chemical vapour deposition. Chem Sci.. 2015; 6:6705.
- Jana MK, Rao CNR. Two-dimensional inorganic analogues of graphene: transition metal dichalcogenides. Phil Trans R Soc A. . 2016;374:20150318.
- Peng H, Chan CK, Meister S, et al. Shape Evolution of Layer-Structured Bismuth Oxychloride Nanostructures via Low-Temperature Chemical Vapour Transport. Chem Mater.. 2009; 21: 247–252.
- Chen X, Zhao H, Wu Y, et al. Methods and Conditions of Dehydration of Hydrated Metal Halides. Chinese Journal of Chemical Education. 2017; 38:12–18.
- Brewer JR, Deo N, Morris Wang Y, et al. Lanthanum Hexaboride Nanoobelisks. Chem Mater.. 2007; 19:6379–6381.