Abstract
Herein, BNPs@Cur-Pd as a versatile and reusable nanocatalyst was successfully synthesized and used for C–C coupling reactions. The Suzuki, Heck and Stille coupling reactions with good to excellent yields were conducted with Pd. Also, the catalyst could be recycled and reused for several sequential runs without considerable Pd leaching or diminishing of reaction yields. This simple methodology can be based on green chemistry protocols by using the boehmite, Curcumin and PEG as support, ligand, and solvent, respectively.
1. Introduction
In recent years, transition metals have been considered as robust and useful catalysts for C(sp2)–C(sp2) coupling reactions mainly Suzuki, Heck, and Stille [Citation1–8]. Typically, the C–C coupling reactions are conducted with a Pd-phosphine catalyst and a base in various solvents that usually take hours or days to complete reaction. Achieving high conversion rates requires high volumes of catalyst and ligand, so using the expensive catalyst and ligand may lead to a sharp increase in the costs. For this reason, many researchers have studied to develop cheaper catalysts and ligands for coupling reactions.
The copious solid supports for Pd are used to provide heterogeneous catalysts that facilitate the separation, reusing and decrease of metal-contamination. For this purpose, numerous solid materials have been utilized as catalyst supports including silica, CNT, polymers, MOF, and zeolites [Citation9–16]. Recently using the boehmite nanoparticles as versatile supports to the production of the new catalyst has received great attention. Boehmite (γ-AlOOH) with oxy-hydroxide bonds has been identified as a metastable phase of aluminum oxide which can be provided in both synthetic and mineral sources [Citation17,Citation18]. In recent years, only a few studies on the synthesis of boehmite based nanocatalysts have been conducted to catalyze organic reactions [Citation19–27].
To modify the catalyst supports, there are copious selections of ligands that were typically synthesized in specific research studies. In the meantime, the use of naturally-derived ligands extracted from natural resources has been taken into consideration. Curcumin is a natural chemical compound found in turmeric spice. Turmeric is a plant root that is scientifically called “Curcuma Luna” [Citation28]. Curcumin is a diarylheptanoid in two tautomeric forms (enol and keto for acetylacetonate group) that can be an enol in organic solvents as well as a keto form in water media [Citation29]. The structural feature of curcumin can be used for organic reactions. Curcumin, as a natural ligand, can be coordinated to metal ions through the acetylacetonate functional group. Also, anti-carcinogenic, antioxidant and anti-inflammatory properties of Curcumin are very well-known [Citation30–32].
Dattatraya et al. have reported the performance of the Pd-Curcumin complex obtained from extracted natural Curcumin to catalyze Suzuki and Heck reactions. The results have shown moderate to excellent efficiency in spite of its low reusability [Citation33]. It seems that only Metal-Curcumin is not sufficient to catalyze organic reactions and the use of stable solid support is required to improve its reusability.
Therefore, in this work, the boehmite surface has been modified with extracted Curcumin to anchor Pd ions. In this regard, Boehmite Nanoparticles Curcumin-Pd (BNPs@Cur-Pd) was utilized as a versatile and reusable nanocatalyst for the carbon-carbon cross-coupling reactions. The schematic of BNPs@Cur-Pd obtained from natural curcumin is shown in Scheme 1.
2. Results and discussion
2.1. Catalyst characterization
shows the FT-IR spectra for BNPs and BNPs@Cur-Pd. In the BNPs spectrum, the two strong frequencies at 3299 and 3095 cm−1 are related to stretching vibrations of free Al–OH bonds. Also, the two frequencies at 1073 and 1161 cm−1 are due to the hydrogen bonding symmetrical bending vibrations between Al–OH groups. The absorption peaks at 752, 616 and 497 cm−1 are due to the vibration modes of the Al–O–Al [Citation17]. The two frequencies at 1384 and 1637 cm−1 are related to the NO3- impurities and the water absorbed in the crystal, respectively.
In the BNPs@Cur spectrum, the broad peak at 3416 cm−1 corresponds to the stretching vibrations of hydrogen-bonded OH groups in phenolic rings of Curcumin. The three peaks at 2852, 2924 and 2954 cm−1 indicate the stretching vibrations of sp3 C–H for CH2 alkane groups. The broadening spectrum in the range of 1600–1715 cm−1 is due to C = O enol-keto tautomer and C = C stretching vibrations. The Keto form of dicarbonyl in the Curcumin molecule shows the vibration at 1693 cm−1 while its enolic form appears at 1622 cm−1. The peak at 1454 cm−1 is related to aromatic C–C stretching vibrations as well as bending vibration of alkane C–H. Also, the peak at 1094 cm−1 indicates the Si–O vibration mode in the BNPs@Cur structure. The existence of BNPs indicative bands in the BNPs@Cur-Pd spectra may be related to the presence of free Al–OH which couldn’t react with CPTES due to some spherical hindrances.
BET adsorption analysis was conducted to observe the porosity properties of BNPs@Cur-Pd nanocatalyst. displays the nitrogen adsorption-desorption isotherms and pore size distribution of BNPs@Cur-Pd nanocatalyst. The IV-type isotherm (defined by IUPAC) in reveals the mesoporosity nature of BNPs@Cur-Pd nanocatalyst [Citation34]. displays the pore size distribution and reveals that most of the pores are in the mesoporous range with 12.24 nm maximum pore size. The BNPs@Cur-Pd with mean pore diameter 31.4 nm is contained in a specific surface area (SBET) of 1.43 m2g−1 and a specific pore volume of 0.0115 cm3g−1. The BET result for BNPs@Cur-Pd has indicated a low specific surface area that may be due to the intense agglomeration among nanoparticles.
Figure 2. N2 adsorption–desorption isotherms (a) and the corresponding pore size distributions (b) for BNPs@Cur-Pd nanocatalyst.
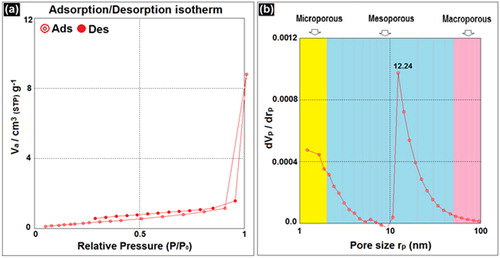
X-ray diffraction as a versatile method was used for the analysis of the phase and crystal structure of nanocatalyst powder. Hence, shows the recorded XRD patterns for BNPs@Cur and BNPs@Cur-Pd. The XRD spectrum of BNPs@Cur displays broad peaks at 2Theta = 14.1°, 28.2°, 38.4°, 45.6°, 49.3°, 55.3°, 61.2°, 64.3°, 67°, 68.3° and 72° related to diffraction lines of (020), (120), (031), (131), (051), (151), (080), (231), (002), (171), (251) and (271), respectively which can be related to orthorhombic structure of boehmite γ-AlOOH (JCPDS card 21-1307). The other peaks can be related to the curcumin phase. The broadening of diffraction peaks in the spectrum shows the lower crystallinity of BNPs@Cur due to Curcumin functionalization. Also, the XRD pattern of BNPs@Cur-Pd indicated the same diffraction lines of BNPs@Cur with the same peaks broadening. With further focus, we can see some differences in BNPs@Cur-Pd versus BNPs@Cur. These differences can be related to the small Pd NPs and the formation of Pd-curcumin complexes at the surface of BNPs@Cur. Pd species in the BNPs@Cur-Pd spectrum indicated several peaks at 2ϴ = 40°, 46.3° and 68.12° related to diffraction lines of (111), (200) and (220), respectively.
The formation of Pd NPs is affirmed by following TEM and SEM images. displays SEM images of (a) BNPs@Cur and (b) BNPs@Cur-Pd powders in several magnifications. In , it can be seen the formation of BNPs@Cur agglomerates (500 nm–5 µm) due to the tendency to hydrogen bonding between functionalized nanoparticles. Also, it was observed some fibers in the matrix of BNPs@Cur which can be related to non-reacted crystalline Curcumin. In comparison with BNPs@Cur, the formation of small Pd NPs at the matrix of BNPs@Cur-Pd was observed in a higher magnified SEM image ().
displays the TEM images of BNPs@Cur-Pd nanocatalyst accompanied by their negative images. It can be seen that BNPs@Cur-Pd has shown needle-like nanoflakes with an average length size of 20–70 nm. The nanoflakes seem to have formed a 3D network by connecting each other, which may have occurred through Curcumin groups. In fact, Curcumin by using bifunctional phenols can form a bridge among the boehmite nanoparticles which led to the formation of a 3D network schematically shown in . Sever agglomeration between this nanostructure occurs. We cannot distinguish the morphology of this 3D network by SEM images. Also, it was observed the good distribution of Pd NPs at the surface of BNPs@Cur nanoflakes as black dots with 5–10 nm sizes.
X-ray mapping and EDX analysis were investigated for the index elements namely C, O, Si and Al in the structure of BNPs@Cur (). As shown in the mapping analysis, the uniform distribution of C and Si at BNPs@Cur matrix represents the appropriate coating of the organic functional groups of the Curcumin and CPTES at BNPs surface. The distribution of the Al element is related to the boehmite phase, which is the precursor for BNPS@Cur synthesis. The EDX spectrum indicates the percentage of index elements in BNPs@Cur (C = 9.73%, Al = 9.71%, Si = 2.46% and O = 45.61%).
shows the X-ray mapping and EDX analysis of BNPs@Cur-Pd. As shown in the mapping analysis, the uniform distribution of Pd elements at the BNPs@Cur-Pd matrix represents the appropriate formation of Pd-Curcumin and Pd NPs in the BNPs@Cur nanoflakes. The EDX spectrum indicates the percentage of index elements in BNPs@Cur-Pd (C = 30.12%, Al = 15.05%, Si = 0.66% and O = 46.67%). Also, the weight percentage of Pd in BNPs@Cur-Pd nanocatalyst was detected to be 2.23% (20.96 10−5 mol g−1).
The thermal properties of the BNPs@Cur-Pd were investigated by thermogravimetric-differential thermal analysis (TG-DTA). According to the TG curve in , four-step weight loss has occurred for BNPs@Cur-Pd. The first step includes a 9.11% mass change between 20 and 160 °C. The DTA curve indicates an endothermic peak at 80 °C, which can be related to the evaporation of solvents and volatile molecules. The second major step displayed a 28.1% mass change between 160 and 410 °C. The DTA curve indicates two exothermic peaks at 220 °C and 276 °C, which can be referred to as the oxidation and combustion processes of organic groups (Curcumin). The other weight loss steps can be related to phase changes of Pd-Si-Alumina that remained from the combustion process.
ICP-OES analysis was conducted to detect the exact amount of Pd content of BCur-Pd nanocatalyst. The analysis result revealed 3.66 × 10−5 mol g−1 (0.389%w Pd) for the palladium content of nanocatalyst.
2.2. Catalytic studies
The catalytic activity of BNPs@Cur-Pd nanocatalyst was evaluated in the carbon-carbon cross-coupling reactions including Suzuki–Miyaura, Heck and Stille reactions. In these reactions, some aryl halides reacted with phenylboronic acid, butyl acrylate, and triphenyltin chloride, respectively. In our first experiments, the Suzuki–Miyaura reaction between iodobenzene and phenylboronic acid was selected as a model reaction by using BNPs@Cur-Pd to optimize the effects of solvent, base, the amount of catalyst and temperature. shows the obtained optimization conditions for Suzuki’s reactions. In the absence of nanocatalyst, no coupling reaction has taken place in entry 1. At the beginning of the study, PEG was used as a solvent and sodium carbonate (Na2CO3) as a base by using 5–15 mg of BNPs@Cur-Pd at 80 °C (entries 2–5). The optimum results were obtained by 12 mg (0.043 × 10−5 mol g−1, 0.043 mol% Pd) of the BNPs@Cur-Pd nanocatalyst (entry 4). Increasing the amount of nanocatalyst did not show a noticeable improvement in the efficiency of the reaction (entry 6).
Table 1. Optimization of the reaction conditions for Suzuki coupling reaction in the presence of BNPs@Cur-Pd as a nanocatalyst.Table Footnotea
Afterward, the evaluation was conducted to change solvent effects for various solvents such as DMF, DMSO, and H2O instead of PEG (entries 6–8). The results revealed that using the other solvents did not lead to an improvement in reaction efficiency. Also, the aprotic solvents (DMF, DMSO) showed better results versus the protic solvents (H2O) which can be due to the nature of curcumin as a ligand in BNPs@Cur-Pd that is a hydrophobic molecule that can be solved freely in the aprotic solvents and this nature can enhance more interactions between solvent and catalyst. Also, the role of PEG solvent as a phase transfer catalyst can promote the basicity of Na2CO3 as well as the increased solubility of other precursors. Then, the optimization was performed on the effect of several bases (KOH, Et3N, and NaOEt) in the coupling reactions (entries 9–11). The obtained results revealed that sodium carbonate was still the best among the other bases to obtain a high yield of product. Finally, the temperature effect was evaluated at 25 °C, 60 and 80 °C and it was found that the Suzuki reaction proceeded effectively at 80 °C.
Afterwards, the other biaryl derivatives were synthesized from different aryl iodides (, entries 1, 3, 5 and 9) and bromides (, entries 2, 4, 6, 7, 8 and 10) under the optimal reaction conditions (12 mg BNPs@Cur-Pd, Na2CO3 as a base, PEG as a solvent, Temp. 80 °C). The results of the Suzuki reaction are shown in .
Table 2. Suzuki coupling reactions of aryl halides with aryl boronic acid catalyzed by BNPs@Cur-Pd nanocatalyst.Table Footnotea
As expected, the reaction times for aryl iodides were less than aryl bromides. The coupling reactions of electron-withdrawing and electron donor substituted aryl halides have been successfully conducted with good to excellent yields in short reaction times (). In (entries 4 and 10) the nitro groups as an electron withdrawing functional groups, unlike electron donor types have affected on coupling reactions in shorter times and higher efficiency. The comparison between entries 6 and 8 in indicated the effect of para or meta situations on coupling reaction. The methoxy group as an electron donor showed a negative effect when it was located in the para position through a strong resonance effect. Therefore, the electron-donating effect decreased when methoxy groups were located in the meta position.
In another attempt, the coupling of aryl halides with 3,4-difluoro phenylboronic acid was conducted under optimized reaction conditions. According to the less reactivity of 3,4-difluoro phenylboronic acid versus unfunctionalized phenylboronic acid, the iodobenzene and 4-nitrobromobenzene in the reaction with 3,4-difluoro phenylboronic acid indicated reasonable yields for their corresponding cross-coupling products (, entries 9–10). Therefore, these results indicated the effectiveness of this methodology for a wide range of aryl halides and aryl boronic acid derivatives. Scheme 2 shows schematically the possible mechanism of the Suzuki coupling reaction by BNPs@Cur-Pd nanocatalyst.
The Stille coupling reaction was studied to illustrate the broad application of BNPs@Cur-Pd as a nanocatalyst. With this aim in mind, the reaction between iodobenzene (1 mmol) and triphenyltin chloride (0.5 mmol) was selected as a model reaction to optimize the effects of the amount of catalyst, base, solvent, and temperature. shows the obtained optimization conditions for the Stille reaction.
Table 3. Optimization of the reaction conditions for Stille coupling using BNPs@Cur-Pd as a nanocatalyst.Table Footnotea
In the absence of nanocatalyst, no coupling reaction has occurred in entry 1. In the beginning, PEG was used as a solvent and Na2CO3 as a base by using of 5–15 mg of BNPs@Cur-Pd at 80 °C (entries 2–5). The optimum results were obtained by 12 mg (0.043 10−5 mol g−1) of the BNPs@Cur-Pd nanocatalyst (entry 4). Increasing the amount of nanocatalyst did not give rise to a sensible improvement in the efficiency of the reaction (entry 5). Then, changing the solvent was conducted in order to evaluate solvent effects by using DMF, DMSO, and H2O instead of PEG (entries 6–8). The results revealed that the PEG solvent was the best in comparison with others. As can be seen in , DMF, and DMSO (as aprotic solvents) present the better results versus H2O as a protic solvent which can be due to the nature of curcumin as an organic ligand in BNPs@Cur-Pd. Also, like what was observed in Suzuki coupling reaction, here, PEG solvent promotes the basicity of Na2CO3 and increases the solubility of other precursors. Next, the optimization was conducted on the effect of various bases such as KOH, Et3N, and NaOEt in the Stille reaction (entries 9–11). The results indicate that sodium carbonate is the best choice to obtain a high yield of product. Finally, the temperature effect was appraised in 25 °C, 60 °C and 80 °C to choose the best reaction temperature. As shown in (entries 4, 12 and 13), the Stille reaction proceeds effectively at 80 °C.
Then, we evaluated the performance of BNPs@Cur-Pd for other substituted aryl halides in Stille reaction under optimal conditions. The results of Stille reaction are summarized in .
Table 4. The Stille coupling reactions of aryl halides with Ph3SnCl catalyzed by BNPs@Cur-PdTable Footnotea.
The various biphenyl derivatives were synthesized from different aryl iodides (, entries 1, 3 and 5) and bromides (, entries 2, 4, 6–9). As expected, the reaction times for aryl bromides were less than aryl iodides. The coupling reaction of electron-withdrawing and electron donor substituted aryl halides have been successfully conducted with good to excellent yields in relatively short reaction times (). Electron withdrawing functional groups, unlike electron donor types, have affected on coupling reactions in shorter times and higher efficiency. The comparison between entries 6 and 8 in indicate the effect of para or meta situations on coupling reaction. Scheme 3 shows schematically the proposed mechanism of the Stille coupling reaction by BNPs@Cur-Pd nanocatalyst.
To develop applications of BNPs@Cur-Pd, the performance of this nanocatalyst was evaluated in the Heck C–C coupling reaction. As a model reaction, BNPs@Cur-Pd has been examined in the cross-coupling of iodobenzene with butyl acrylate to obtain the optimum conditions. The summary of the optimization results is shown in . No reaction occurred in the absence of nanocatalyst (, entry 1).
Table 5. Optimization of the reaction conditions for Heck coupling over the BNPs@Cur-Pd catalyst.Table Footnotea
In the beginning, PEG was used as a solvent and Na2CO3 as a base by using 12–20 mg BNPs@Cur-Pd at 120 °C (entries 2–4). The best amount was obtained by 16 mg (0.058 × 10−5 mol g−1) of the BNPs@Cur-Pd nanocatalyst (entry 3). Among the various solvents (DMF, DMSO, H2O, PEG), again PEG was selected as the best solvent. Then, optimization was conducted on the effect of various bases (KOH, Et3N, and NaOEt) in the Heck reaction (entries 8–10). Based on obtained results in , sodium carbonate was the best base to produce the desired products in high yields. To set the best reaction temperature, the temperature effect was evaluated at 25 °C, 100 °C, and 120 °C and it was found that 120 °C is the best choice in the Heck reaction (, entries 3, 11, 12).
Afterward, we investigated the performance of BNPs@Cur-Pd for other substituted aryl halides in cross-coupling Heck reaction under optimized reaction conditions. The results of Heck derivations are summarized in .
Table 6. The Heck coupling reactions for aryl halides with alkenes catalyzed by BNPs@Cur-Pd nanocatalyst.Table Footnotea
As shown in , the various aryl halides (including Br and I) with electron donor and electron-withdrawing substitution were investigated in Heck coupling with Butyl acrylate to obtain the corresponding products. All coupling products were afforded in good to excellent efficiency. Furthermore, the reaction of aryl iodides with acrylonitrile and methyl acrylate was conducted in comparison with butyl acrylate (, entries 8–10). The efficiency and reaction times for coupling of methyl acrylate are almost the same as butyl acrylate.
The nitrile in acrylonitrile is a withdrawing functional group, so it is expected to have less efficiency and higher reaction time versus butyl acrylate. Despite this expectation, this methodology shows high reactivity in heck coupling for acrylonitrile similar to butyl acrylate. It seems that BNPs@Cur-Pd as nanocatalyst is effective for Heck coupling of various alkenes and aryl halides. Scheme 4 schematically presents the proposed mechanism of the Heck coupling reaction by BNPs@Cur-Pd catalyst.
displays the previously reported activity of various pd nano-catalysts for the Suzuki coupling of iodobenzene with phenylboronic acid. The comparison indicates that BNPs@Cur-Pd as a versatile nanocatalyst is comparable to or maybe better in some aspects of the reaction than the other reported catalysts. Using the Curcumin as a natural ligand in reaction with boehmite can be one aspect of green chemistry in catalyst development.
Table 7. Comparing the performance of BNPs@Cur-Pd catalyst with the previously reported procedure in the C–C coupling of iodobenzene and phenylboronic acid.
The reusability and recovery of the nanocatalyst are a very significant aspect of commercial applications. Therefore, in the Suzuki coupling reaction for iodobenzene with phenylboronic acid, BNPs@Cur-Pd was separated by centrifugation after the reaction completion. Then, it was washed thoroughly with acetone, dried in an oven and reused for next coupling reactions. It was revealed in that at least for 5 runs BNPs@Cur-Pd has been reused and recovered without remarkable abatement in its reactivity. For the Suzuki reaction, the average isolated yield for 5 runs was obtained 93.2%. Pd-leaching and heterogeneity of the BCur-Pd were evaluated by hot filtration. We conducted hot filtration in the Suzuki reaction through the coupling of iodobenzene and phenyl boronic acid. The nanocatalyst was separated in half time of the reaction and filtrate was allowed to react further. The negligible increase in the yield reaction was observed (only 2%). This confirmed that Pd-leaching from BNPs@Cur-Pd was not very significant for coupling reactions. Also, ICP-OES analysis revealed that the Pd contents of fresh and recovered (after five runs) nanocatalyst were 0.00366 mmol g−1 and 0.00312 mmol g−1, respectively. This result indicated the very negligible Pd leaching from BNPs@Cur-Pd as a nanocatalyst. Given the importance of the amount of palladium leakage as heavy metal in terms of the US Food and Drug Administration (FDA), according to the ICP results for the new nanocatalysts and after 5 use run, it can be calculated that the amount of catalyst leaked after five-run is 0.00054 mmol Pd per gram of catalyst. According to the amount of catalyst used in each reaction flask (12 mg/2 ml solvent), it can be calculated that the amount of palladium leaked to the solvent for 12 mg nanocatalyst is 0.00000648 mmol palladium equivalent to 0.0006896 mg palladium at 0.002 L solvent. Therefore, the concentration of leached palladium in the solvent in the total of five reaction runs is 0.344 ppm. That is, about 0.069 ppm for each run. This amount of palladium leakage is very small and below the FDA limit. The residual Pd is below the FDA allowed 10 ppm-level.
3. Experimental
All solvents and chemicals utilized in this study were purchased from Fluka, Sigma-Aldrich and Merck companies and were utilized without further purification. The XRD patterns of nanomaterials were conducted by utilizing Philips PW 3040 diffractometer with Cu Kα (λ = 1.5406 Å) radiation. The morphology of nanopowders was evaluated by scanning electron microscopy (SEM) by utilizing the FE-SEM MIRA3 TESCAN (30 Kv) instrument. Also, the SEM-EDS detector was used for the elemental analysis of nanopowders. The JEOL-2100 microscope was utilized to obtain TEM images from nanocatalyst. The KBr disk samples were used to record FT-IR spectra by utilizing Bruker alpha-instrument. The 1H-NMR analysis was conducted by using Bruker AVANCE DPX-400 (400 MHz). In the air atmosphere, the TG-DTA thermograms of powders were obtained by utilizing the STA PT-1000 instrument at the rate of 10 °C min−1. BET analysis of nanocatalyst was conducted by utilizing the Belsorp mini II instrument. Before the start of the analysis, the sample was degassed at 373 K for five hours. The ultrasonic bath (Bandelin DT52 H/240 W-35 kHz) was used to sonicate solutions. The Pd content of catalyst was determined with ICP-OES technic conducted with Optima 7300DV (PerkinElme, USA) instrument.
3.1. Synthesis of boehmite nanoparticles (BNPs)
BNPs were prepared according to the previously reported recipe [Citation13]. Briefly, the 50 mL aqueous solution of NaOH (3.25 M) was slowly added to 30 mL Al(NO3)3.9H2O solution (3.13 M) under vigorous stirring. The resulted precipitate was ultra-sonicated for 3 h at 25 °C. Then the obtained gel-like precipitate was crystallized by heating at 220 °C for 4 h. Finally, the white powder was washed with water and dried in the oven at 70 °C.
3.2. Extraction of curcumin
Curcumin was extracted from fresh turmeric powder by using ethanol as a solvent. 20 grams of fine powder of turmeric was dispersed in 100 mL EtOH and was stirred for 1 h. After that, the solution was filtered and Curcumin was obtained in the ethanol media.
3.3. Preparation of BNPs@Cur-Pd
BNPs (15 g) were added to the 20 mL of solvents (1:4 v/v H2O/EtOH) and were ultrasonicated for 15 min. Then, 15 mL of (3-chloropropyl)triethoxysilane (CPTES) was added to the mixture and under the nitrogen atmosphere refluxed at 50 °C for 8.5 h. Next, a 10 mL of curcumin solution was added to the mixture and refluxed at 70 °C for 14 h to obtain nanoparticles. The orange-colored solution was centrifuged and washed with ethanol, then dried at 70 °C. To anchor Pd on the BNPs@Cur support, BNPs@Cur powder (8 g) was dispersed and sonicated 15 min in the mixture of H2O/EtOH (20 mL, 1:1 v/v H2O/EtOH). Then, palladium acetate (0.085 g) was added to the reactor and stirred for 24 h at ambient temperature. The dark gray BNPs@Cur-Pd powder was obtained after separation, by washing with ethanol and drying it in an oven.
3.4. General procedure for Suzuki reactions
In a screw cap vial including a magnetic stirrer bar, aryl halide (1 mmol) reacts with aryl boronic acid (1 mmol) and Na2CO3 (1.5 mmol), in the presence of BNPs@Cur-Pd nanocatalyst (12 mg) in PEG (2 mL) as solvent. The screw cap vial was heated at 80 °C for the period of time as listed in . It was followed until the reaction was accomplished (TLC method was used to monitor the progress of reaction). After cooling the reaction mixture, the nanocatalyst was isolated by centrifugation, and then was rinsed with acetone and it was put in the oven to dry. Next, Et2O (3 mL, 4 times) was used for the extraction of the organic layer from the reaction mixture in a separating funnel. At last, the Et2O was removed to afford a uniform and pure coupled product in excellent yield.
3.5. General procedure for Stille reactions
In a screw cap vial including a magnetic stirrer bar, aryl halide (1 mmol), triphenyltin chloride (0.5 mmol) and Na2CO3 (1.5 mmol), react with BNPs@Cur-Pd (12 mg) in 2 mL PEG. The screw cap vial was heated at 80 °C for the specified times as shown in . It was followed by TLC until the reaction was completed. After cooling the reaction mixture, the nanocatalyst was isolated by centrifugation, rinsed with acetone and put it the oven to dry. Then, Et2O (3 mL, 4 times) was used for the extraction of organic layer from the PEG mixture in a separating funnel. Finally, the Et2O was vaporized to obtain a uniform and pure product in excellent yield.
3.6. General procedure for Heck reaction
In a screw cap vial including a magnetic stirrer bar, aryl halides (1 mmol) react with alkene (1.2 mmol) and Na2CO3 (1.5 mmol) with BNPs@Cur-Pd (16 mg) in 2 mL of PEG. The screw cap was heated at 120 °C for the amount of time (). The reaction mixture was monitored by TLC until the reaction was done. Then, the reaction mixture was cooled and nanocatalyst isolated by centrifugation, rinsed with acetone and dried in the oven to next run. The product was extracted with diethyl ether (3 mL, 4 times). Eventually, evaporation of diethyl ether gave coupled product in excellent yield with high purity.
Disclosure statement
No potential conflict of interest was reported by the author(s).
Additional information
Funding
Notes on contributors
Muhammed Ali Jani
Muhammed Ali Jani is Ph.D. student of nanochemistry under the guidance of Dr. Kiumars Bahrami at faculty of chemistry, Razi university, Kermanshah, Iran. His interest covers the application of nanocatalysts in organic reactions.
Kiumars Bahrami
Dr. Kiumars Bahrami is working as a Professor of organic chemistry in faculty of chemistry, Razi university, Kermanshah, Iran. He has published many papers in national and international journals. He has also participated and presented several papers at national and international conferences. To date, he has supervised 33 M.Sc. and 12 Ph.D. students. He is developing research programs in the areas of nanostructured materials in organic syntheses.
References
- Tobisu M, Xu T, Shimasaki T, et al. Nickel-catalyzed Suzuki–Miyaura reaction of aryl fluorides. J Am Chem Soc. 2011;133(48):19505–19511..
- Bahrami K, Nakhjiri Kamrani S. Synthesis, characterization and application of graphene palladium porphyrin as a nanocatalyst for the coupling reactions such as: Suzuki‐Miyaura and Mizoroki‐Heck. Appl Organometal Chem. 2018;32(2):e4102..
- Bahrami K, Targhan H. A new strategy to design a graphene oxide supported palladium complex as a new heterogeneous nanocatalyst and application in carbon–carbon and carbon‐heteroatom cross‐coupling reactions. Appl Organometal Chem. 2019;33(4):e4842..
- Li JH, Liang Y, Xie YY. An efficient Stille cross-coupling reaction catalyzed by Pd (OAc) 2/DAB-Cy catalytic system. Tetrahedron. 2005;61(30):7289–7293..
- Targhan H, Hassanpour A, Bahrami K. Highly efficient polymer‐stabilized palladium heterogeneous catalyst: Synthesis, characterization and application for Suzuki–Miyaura and Mizoroki–Heck coupling reactions. Appl Organometal Chem. 2019;33(10):e5121..
- Kantam ML, Srinivas P, Yadav J, et al. Trifunctional N, N, O-terdentate amido/pyridyl carboxylate ligated Pd (II) complexes for Heck and Suzuki reactions. J Org Chem. 2009;74(13):4882–4885..
- Bahrami K, Khodaei MM, Meibodi FS. Suzuki and Heck cross‐coupling reactions using ferromagnetic nanoparticle‐supported palladium complex as an efficient and recyclable heterogeneous nanocatalyst in sodium dodecylsulfate micelles. Appl Organometal Chem. 2017;31(6):e3627..
- Ghorbani-Choghamarani A, Tahmasbi B, Moradi P. Palladium–S‐propyl‐2‐aminobenzothioate immobilized on Fe3O4 magnetic nanoparticles as catalyst for Suzuki and Heck reactions in water or poly (ethylene glycol). Appl Organometal Chem. 2016;30(6):422–430..
- Karimi B, Fadavi Akhavan PA. novel water-soluble NHC-Pd polymer: an efficient and recyclable catalyst for the Suzuki coupling of aryl chlorides in water at room temperature. Chem Commun. 2011;47(27):7686–7688..
- Rostamnia S, Xin H. Pd (OAc) 2@ SBA‐15/PrEn nanoreactor: a high active, reusable and selective phosphine‐free catalyst for Suzuki–Miyaura cross-coupling reaction in aqueous media. Appl Organometal Chem. 2013;27(6):348–352..
- Zhang R, Ding W, Tu B, et al. Mesoporous silica: an efficient nanoreactor for liquid − liquid biphase reaction. Chem Mater. 2007;19(18):4379–4381..
- Mandal S, Roy D, Chaudhari RV, et al. Pt and Pd nanoparticles immobilized on amine-functionalized zeolite: excellent catalysts for hydrogenation and heck reaction. Chem Mater. 2004;16(19):3714–3724..
- Wu CD, Hu A, Zhang L, et al. A homochiral porous metal − organic framework for highly enantioselective heterogeneous asymmetric catalysis. J Am Chem Soc. 2005;127(25):8940–8941..
- Pan X, Fan Z, Chen W, et al. Enhanced ethanol production inside carbon-nanotube reactors containing catalytic particles. Nat Mater. 2007;6(7):507–511..
- Castillejos E, Debouttiere PJ, Roiban L, et al. An efficient strategy to drive nanoparticles into carbon nanotubes and the remarkable effect of confinement on their catalytic performance. Angew Chem Int Ed. 2009;48(14):2529–2533..
- Bai L, Wang JX. Reusable, polymer‐supported, palladium‐catalyzed, atom-efficient coupling reaction of aryl halides with sodium tetraphenylborate in water by focused microwave irradiation. Adv Synth Catal. 2008;350(2):315–320..
- Rajabi L, Derakhshan AA. Room temperature synthesis of boehmite and crystallization of nanoparticles: effect of concentration and ultrasound. Sci Adv Mat. 2010;2(2):163–172..
- Liu J, Liao X, Shi B. Pd nanoparticles immobilized on boehmite by using tannic acid as structure-directing agent and stabilizer: a high performance catalyst for hydrogenation of olefins. Res Chem Intermed. 2014;40(1):249–258. https://doi.org/10.1007/s11164-012-0959-1.
- Ghorbani-Choghamarani A, Tahmasbi B, Arghand F, et al. Nickel Schiff-base complexes immobilized on boehmite nanoparticles and their application in the oxidation of sulfides and oxidative coupling of thiols as novel and reusable nano organometal catalysts. RSC Adv. 2015;5(112):92174–92183..
- Bahrami K, Khodamorady M. Reusable BNPs‐SiO2@(CH2) 3NHSO3H‐catalysed selective oxidation of sulfides to sulfone. Appl Organometal Chem. 2018;32(12):e4553.
- Mirzaee M, Bahramian B, Amoli A. Schiff base‐functionalized boehmite nanoparticle‐supported molybdenum and vanadium complexes: efficient catalysts for the epoxidation of alkenes. Appl Organometal Chem. 2015;29(9):593–600..
- Khodamorady M, Bahrami K. Design of BNPs-TAPC palladium complex as a reusable heterogeneous nanocatalyst for the O-arylation of phenols and N-arylation of amines. Catal Lett. 2019;149(3):688–698..
- Bahrami K, Khodaei MM, Roostaei M. The preparation and characterization of boehmite nanoparticles-TAPC: a tailored and reusable nanocatalyst for the synthesis of 12-aryl-8, 9, 10, 12-tetrahydrobenzo [a] xanthen-11-ones. New J Chem. 2014;38(11):5515–5520..
- Khodamorady M, Bahrami K. Fe3O4@ BNPs‐CPTMS‐Chitosan‐Pd (0) as an efficient and stable heterogeneous magnetic nanocatalyst for the chemoselective oxidation of alcohols and homoselective synthesis of 5‐subestituted 1H‐tetrazoles. Chemistryselect. 2019;4(28):8183–8194..
- Ghorbani-Choghamarani A, Tahmasbi B, Moradi P. Synthesis of a new Pd (0)-complex supported on boehmite nanoparticles and study of its catalytic activity for Suzuki and Heck reactions in H2O or PEG. RSC Adv. 2016;6(49):43205–43216..
- Ghorbani-Choghamarani A, Derakhshan AA, Hajjami M, et al. Palladium-alumoxane framework as a novel and reusable nanocatalyst for Suzuki–Miyaura, Stille and Heck cross coupling reactions. Catal Lett. 2017;147(1):110–127..
- Ghorbani-Choghamarani A, Derakhshan AA, Hajjami M, et al. Copper–Schiff base alumoxane: a new and reusable mesoporous nano catalyst for Suzuki–Miyaura and Stille C–C cross-coupling reactions. RSC Adv. 2016;6(97):94314–94324..
- Ahamefula I, Onwuka GI, Chibuzo N. Nutritional composition of Tumeric (Curcuma longa) and its antimicrobial properties. Int J Sci Eng Res. 2014;5:1085–1089.
- Manolova Y, Deneva V, Antonov L, et al. The effect of the water on the curcumin tautomerism: A quantitative approach. Spectrochim Acta Part A: Mol Biomol Spectrosc. 2014;132:815–820..
- Yadav RP, Tarun G. Versatility of turmeric: A review the golden spice of life. J Pharmacogn Phytochem. 2017;6:41–46.
- Shrishail D, Handral HK, Ravichandra Tulsianand HG, et al. Turmeric: Nature’s precious medicine. Asian J Pharm Clin Res. 2013;6:10–16.
- Takada Y, Bhardwaj A, Potdar P, et al. Nonsteroidal anti-inflammatory agents differ in their ability to suppress NF-κB activation, inhibition of expression of cyclooxygenase-2 and cyclin D1, and abrogation of tumor cell proliferation. Oncogene. 2004;23(57):9247–9258.,.
- Bhange DS, Rasal NK, Sonawane RB, et al. Application of Curcumin as ligand in [Pd (Curc‐H) 2] catalyst for Carbon‐Carbon bond formation in Heck and Suzuki coupling reactions. Chemistryselect. 2018;3(40):11230–11235..
- Cui HJ, Shi JW, Yuan B, et al. Synthesis of porous magnetic ferrite nanowires containing Mn and their application in water treatment. J Mater Chem A. 2013;1(19):5902–5907..
- Peng YY, Liu J, Lei X, et al. Room-temperature highly efficient Suzuki–Miyaura reactions in water in the presence of Stilbazo. Green Chem. 2010;12(6):1072–1075..
- Ghorbani‐Choghamarani A, Tahmasbi B, Moradi Z. Benzylisothiourea complex of palladium on magnetic nanoparticles: A highly efficient and reusable nanocatalyst for synthesis of polyhydroquinolines and Suzuki reaction. Appl Organometal Chem. 2017;31(8):e3665..
- Ghorbani-Choghamarani A, Moradi P, Tahmasbi B. Modification of boehmite nanoparticles with Adenine for the immobilization of Cu (II) as organic–inorganic hybrid nanocatalyst in organic reactions. Polyhedron. 2019;163:98–107..
- Ghorbani-Choghamarani A, Norouzi M. Palladium supported on modified magnetic nanoparticles: a phosphine‐free and heterogeneous catalyst for Suzuki and Stille reaction. Appl Organometal Chem. 2016;30(3):140–147..
- Ghorbani‐Choghamarani A, Tahmasbi B, Noori N, et al. Pd–S-methylisothiourea supported on magnetic nanoparticles as an efficient and reusable nanocatalyst for Heck and Suzuki reactions. C R Chimie. 2017;20(2):132–139..
- Nikoorazm M, Ghorbani-Choghamarani A, Panahi A, et al. Pd (0)-Schiff-base@ MCM-41 as high-efficient and reusable catalyst for C–C coupling reactions. J Iran Chem Soc. 2018;15(1):181–189..
- Chen T, Gao J, Shi M. A novel tridentate NHC–Pd (II) complex and its application in the Suzuki and Heck-type cross-coupling reaction. Tetrahedron. 2006;62(26):6289–6294..
- Mohammadinezhad A, Akhlaghinia B. Fe3O4@ Boehmite-NH 2-Co II NPs: an inexpensive and highly efficient heterogeneous magnetic nanocatalyst for the Suzuki–Miyaura and Heck–Mizoroki cross-coupling reaction. Green Chem. 2017;19(23):5625–5641..
- Ghorbani‐Choghamarani A, Tahmasbi B, Noori N, et al. A new palladium complex supported on magnetic nanoparticles and applied as an catalyst in amination of aryl halides, Heck and Suzuki reactions. J Iran Chem Soc. 2017;14(3):681–693..
- Islam SM, Roy AS, Mondal P, Salam N. Selective hydrogenation and Suzuki cross‐coupling reactions of various organic substrates using a reusable polymer‐anchored palladium (II) Schiff base complex. Appl Organometal Chem. 2012;26(11):625–634..
- Ghorbani-Choghamarani A, Rabiei H. Synthesis, characterization, and application of palladium-dithizone immobilized on magnetic nanoparticles as an efficient and recoverable catalyst for Suzuki type coupling reactions. Tetrahedron Lett. 2016;57(1):159–162..
- Rostamnia S, Doustkhah E, Zeynizadeh B. Cationic modification of SBA-15 pore walls for Pd supporting: Pd@ SBA-15/ILDABCO as a catalyst for Suzuki coupling in water medium. Microporous Mesoporous Mater. 2016;222:87–93..
- Wang HB, Zhang YH, Yang HL, et al. Palladium immobilized in the nanocages of SBA-16: An efficient and recyclable catalyst for Suzuki coupling reaction. Microporous Mesoporous Mater. 2013;168:65–72..
- Nasrollahzadeh M, Sajadi SM, Maham M. Green synthesis of palladium nanoparticles using Hippophae rhamnoides Linn leaf extract and their catalytic activity for the Suzuki–Miyaura coupling in water. J Mol Catal A Chem. 2015;396:297–303..
- Faria VW, Oliveira DGM, Kurz MHS, et al. Palladium nanoparticles supported in a polymeric membrane: an efficient phosphine-free “green” catalyst for Suzuki–Miyaura reactions in water. RSC Adv. 2014;4(26):13446–13452..
- Nasrollahzadeh M, Azarian A, Maham M, et al. Synthesis of Au/Pd bimetallic nanoparticles and their application in the Suzuki coupling reaction. J Ind Eng Chem. 2015;21:746–748..