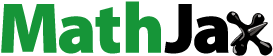
Abstract
The biomolecule-coated nanotitania catalysts were synthesised using rhizome extract of Gloriosa superba and the characteristics of the synthesised nanocatalysts were investigated by using various physiochemical methodologies. The antibacterial activity of the biomolecule coated nanotitania catalysts was tested against harmful microbial human pathogens. Nanotitania catalysts were found to be the most potential agent against gram negative bacterium i.e. Staphylococcus epidermidis. An efficient anti-biofilm activity was also observed against biofilm developing bacteria namely S. epidermidis and Pseudomonas aeruginosa. The 50% inhibitory concentration (IC50) of nanotitania catalysts noticed was 46.64 and 61.81 µg/mL for MCF-7 (cancer) L929 (normal) cell lines, respectively. Bioengineered nanotitania catalysts exhibited potential anticancer activity against breast cancer cell line. AO/EtBr staining results show distinct morphological variations such as orange and red coloured apoptotic bodies were identified in cancer cells that were treated with nanotitania catalysts. Further, the nuclear changes, mitochondrial depolarization and increased reactive oxygen species (ROS) level were also detected in nanotitania catalysts treated MCF-7 cells by Hoechst, rhodamine and DCFH-DA probe staining techniques. COMET assay confirmed the DNA destruction in the nanotitania treated cancer cells. In addition, the nanotitania catalysts exhibited potential photocatalytic activity against inorganic toxic dyes and the maximum rate of dye degradation was observed for crystal violet. The present results strongly suggest that the biomolecule coated nanotitania catalysts could be used as potential and novel compound towards biomedical as well as photocatalytic applications.
Graphical Abstract
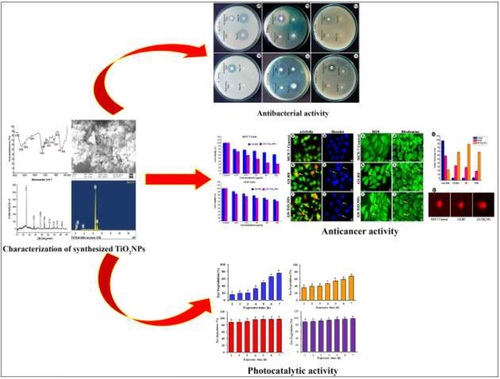
1. Introduction
Nanotechnology is being considered as the most promising technique in modern scientific system due to their effective mode of activities of nanoparticles and extensive applications in the field of biotechnology, medicine and chemistry [Citation1, Citation2]. Among metal oxide nanoparticles, titanium nanoparticles (TiO2·NPs) are being used for industry, agriculture and consumer products including sunscreens, electronics, cosmetics and food products [Citation3]. Currently, metal oxide nanoparticle synthesis is an emerging zone of all the research and the green synthesis of nanoparticles has increased exponentially over the past several years [Citation4]. Nowadays, phytonanotechnology which is a modest, cost-effective and environmental friendly method has a quick way for the synthesis of nanoparticles externally [Citation5]. Phytonanotechnology has several advantages including medical applicability of nanoparticle synthesis using water as a reducing solvent [Citation6]. Dissolvable plant extracts may execution as reducing and capping agent in metal oxide nanoparticle synthesis [Citation7]. Plant-endured biomolecules can also be used for the accelerated and functioning extracellular synthesis of TiO2·NPs [Citation8]. Most recently, the plant-mediated fusion of TiO2 NPs exhibit efficient antibacterial, larvicidal and photocatalytic activity [Citation9, Citation10]. There are triple crystalline points are having TiO2 NPs, where the anatase facet was exhibited immense photocatalytic and better antibacterial properties [Citation11, Citation12].
In the recent past, the degradation of dyes has major attention, specifically along with the usage of TiO2-based photocatalysts. The non-toxic, high energetic and magnificent physicochemical balance of TiO2 NPs generates attractively when compared to other semiconductors [Citation13–15]. Antimicrobial activity is one of the utmost crucial applications in the ongoing research of biomedical sciences. Several virulent pathogenic species like Staphylococcus epidermidis, Escherichia coli, Staphylococcus aureus, Proteus vulgaris, Pseudomonas aeruginosa and Klebsiella pneumoniae cause numerous infection in human. The above mentioned types of pathogens were induced severe diseases, therefore, we need to establish a potential antibiotic to remedy the bacterial infections. Occasionally, the prescriptive antibiotics may not able to kill or inhibit the growth of harmful virulence bacteria. The brand-new antibiotics invented by pharma group did not show effective movement against multidrug-contrary bacterial strains. Therefore, it is urgent to develop novel and nano-based drug to control bacterial infections [Citation16]. Development of resistance to the existing antibiotic by pathogens is one of major problems in the current therapeutic field. Accordingly, the exploration for novel stimulant with a new mechanism of action is a big challenge in the pharma industries and researchers. The combination of medicinal plant derived biomolecules coated nanomaterial is being considered as major possible sources of novel antimicrobial agents. Furthermore, cancer is causing the most leading deaths across the world and it is discriminated by the irregular cell division, improvement and proliferation in humans body. Various methods are currently used for the prescription of cancer including operations, chemotherapy and radiotherapy. However, these methods are very expensive and have a lot of side effects with some restrictions. Thus, it is an urgent need to make anticancer drugs at low cost without showing much side effects as well as less toxic for cancer treatment [Citation17]. Among all type of cancer, breast carcinoma is the utmost common invasive type in females worldwide also millions of women are struggling due to breast cancer. Lack of effective therapeutic drugs, as well as strategies to control and treat breast cancers, makes massive financial trouble situated on entity and society. Hence, an alternative effort may be taken to invent biomolecules loaded nano-based drugs to fight against breast cancer.
Gloriosa superba L. is an important medicinal climber, extensively scattered in the hot and sub-tropical side of India. In Ayurveda (Indian system of medicine), the tubers are benefited to treat antiperiodic, antihelminthic and also snake bites. It is used as poultices to relieve neuralgia, to treat trembling conditions, injury of the elbow, sprains, leprosy, chronic ulcers, cancer and dislocations [Citation18]. A paste of the bulb is visibly applied for skin infection. Rhizomes, sheath and leaves were also used to treat worms infection, liver cracked and filarial. It is reported that G. superba extracts show potential antimicrobial activity against various pathogens such as Candida albicans, Candida glabrata, Trichophyton longifusus, Microsporum canis and Staphylococcus aureus [Citation19]. Earlier reports showed the effective antibacterial activity of various nanoparticles including ruthenium [Citation20], copper oxide [Citation21] and cerium oxide [Citation22] synthesised using G. superba. However, till date, there are no reports available towards photocatalytic dye degradation, antibiofilm and therapeutic applications of nanotitania catalysts synthesised using G. superba rhizome extract.
In view of above, the present investigation was initiated to synthesise and characterization of biomolecule coated nanotitania catalysts using rhizome extracts of G. superba. The probable antibacterial activity of nanotitania catalysts was examined against clinically isolated 6 human pathogens and also assessed the antibiofilm activity towards two biofilm producing bacteria namely S. epidermidis and P. aeruginosa by crystal violet staining. Further, the cytotoxic role of the synthesised nanotitania catalysts was studied against MCF-7 (breast cancer cell line) and normal fibroblast mouse cells (L929). Also, the photocatalytic activity of the synthesised nanotitania catalysts was determined against various organic toxic dyes under solar irradiation.
2. Experimental section
2.1. Preparation of Gloriosa superba rhizome extract
Rhizomes of G. superba L. were collected from Thuraiyur, Tamil Nadu, India and washed under tap water to remove soil substances. About 50 g of rhizome sample was sliced and ground with mortar and pestle by adding 100 mL of sterile distilled water and boiled under microwave oven at 60 °C for 15 min. Then, the boiled rhizome mixture was allowed to coldish at normal temperature and filtered over a Whatman No. 1 filter paper.
2.2. Fabrication and characterization of titanium dioxide nanoparticles (TiO2·NPs)
The prepared rhizome extract was used for fabrication of TiO2 NPs successive the method of Keerthika et al. [Citation9]. About 100 mL of rhizome extract was fused with 900 mL of TiO(OH)4 solution (5 mM) and incubated for 48 h. The bioreduction of titanium ions was subjected to UV–visible spectroscopy (Shimadzu, UV-1800) in the observation ranging between 300 and 700 nm. After biosynthesis, the solution mixture was centrifuged for 10 min and the nano pellet was rinsed three times with sterile distilled water to pull out contaminants from synthesised TiO2 NPs. The transparent nanotitania were dried and study for further characterization of nanotitania catalysts. The presence of promising bioactive molecules involved in the reduction and capping of TiO2 NPs was identified by Fourier transform infrared (FTIR) analysis using an FTIR spectrometer (Bruker Tensor). The crystalline structure and size of the TiO2·NPs were detected by X-ray diffraction (XRD) analysis (Rigaku mini flex II). In addition, the morphology and shape of the TiO2 NPs were determined by Field emission scanning electron microscope (FESEM, Carl Zeiss Ultra55 model) and the elemental configuration of the TiO2·NPs was proved by Energy dispersive X-ray (EDX) analysis.
2.3. Antibacterial activity assay of bio-fabricated nanotitania catalysts
The antibacterial potential of TiO2·NPs was determined against clinically isolated harmful human pathogens namely E. coli, S. aureus, S. epidermidis, P. aeruginosa, E. coli, K. pneumoniae and P. vulgaris by the standard disc diffusion method [Citation9]. The painful bacterial pathogens were obtained from Mohan Kumaramangalam Government Medical College Hospital, Salem. Such microbial pathogens were maintained in nutrient agar (NA) slants and subcultured frequently in the laboratory. A loop full of the bacterial strains were added into nutrient broth medium and incubated on a rotary shaker at 37 °C overnight with the turbidity comparable to 0.5 Mc Farland standards. The prepared nutrient agar was sterilised by autoclaving on 121 °C for 15 min and the nutrient agar was spill into sterile petri plates for solidifying. After solidification, uniformly spread the bacterial culture over the plates by swab method. The sterile discs were placed onto solidified NA plates. Dried TiO2 NPs were diffuse in sterile distilled water (5 mg/mL). Aqueous rhizome extract (5 µL) and TiO2 NPs (5, 10 µL) were aliquoted and added onto sterile discs. Streptomycin discs were used in the act of positive control. The plates were stored in an incubator for overnight at 37 °C. Over the incubation period, around the disc, the inhibition of bacterial growth was noticed and measured.
2.4. Assessment of in vitro anti-biofilm activity of biomolecule-coated nanotitania catalysts
To examine the influence of nanotitania catalysts on decrement of biofilm formation, such a technique was accomplished by Prasannaraj and Venkatachalam [Citation23]. Briefly, nutrient broth (4 mL) and bacterial culture (1 mL) were added into individual sterile glass tubes. Further, rhizome extracts, synthesised nanotitania catalysts, antibiotics at various doses (10, 20, 30, 40 and 50 µg/mL) were additionally added into the tubes. Each tubes were kept in the shaking incubator at 37 °C for 24 h. Afterward, mixture of the individual tube was smoothly removed and each tube was washed with distilled water. The tubes were breeze dried for 5 min, then 5 mL of crystal violet solution (0.2% (w/v)) added into all the tubes and incubated at 37 °C for 45 min. After incubation, discarded the crystal violet solution and washed excess stain in the tubes with sterile distilled water. The dye fused by the adherent cells and the cells stabilised with 5 mL of ethanol (95% v/v). After that, the absorbent wavelength of all tubes were measured on 595 nm using UV–spectrophotometer (Shimadzu, UV-1800). The percentage of biofilm inhibition was calculated by the following formula:
All the tests were executed in triplicate, the data are conveyed as mean ± SE.
2.5. In vitro anticancer activity assay of biomolecule-coated nanotitania catalysts
2.5.1. Collection of cell line and maintenance
The present work was performed in MCF-7 (human breast cancer cell line) and L929 (normal mouse fibroblast cell line). Such cell lines were procured from NCCS (National Centre for Cell Science) Pune, India. MCF-7 cells were grown-up in high-glucose DMEM (Dulbecco’s Modified Eagle’s Medium). The medium supplemented with FBS (10% v/v) and antibiotic/antimycotic reagent (1%). The cell line was retained in culture at 37 °C and 5% of humidified CO2 atmosphere.
2.5.2. Cytotoxicity determination by MTT assay
The cell viability of G. superba rhizome extract (GSRE) and TiO2 NPs were assessed by 3-(4,5-dimethylthiazol-2-yl)-2,5-diphenyltetrazolium bromide (MTT) assay. Briefly MCF-7 and L929 cells were seeded in a 96-well plate (3 × 103 cells/well) and the plates were incubated at 37 °C for 24 h in a humidified CO2. Over the incubation, the cells were treated continuously by the inclusion of test composites (GSRE and nanotitania catalysts) in various doses (6.25, 12.5, 25, 50 and 100 µg/mL). After 24 h, the cell viability calculated by addition of MTT 10 µL at a concentration of (5 mg/mL) for 4 h at 37 °C. Over the incubation period, the MTT and medium were removed, and the treated cells were dissolved with dimethyl sulfoxide (DMSO) for precipitate of MTT. The absorbance of dissolved formazan crystals was measured at 570 nm in a microplate reader. For cell morphology and cell density images were noticed and documented with a phase-contrast microscope (Olympus, Japan). The optical density (OD) values were used to analyze the cell activity percentage by the following formula:
2.5.3. Morphological detection of apoptotic cells by AO/EtBr and Hoechst staining
To determine the morphological changes of apoptosis and necrosis in MCF-7 cells were treated with IC50 concentration of GSRE (55.30 µg/mL) and TiO2 NPs (46.64 µg/mL). Over 24 h of incubation, the medium was removed gently, plates were washed twice with PBS dried. About 200 μL of AO/EtBr and Hoechst reagent was added to stain each well and incubated for a few min at 37 °C and monitored under a fluorescence microscope (Nikon Eclipse TS 100). Fluorescence was detected with an activity wavelength (460 nm) and transmission wavelength (maximum 650 nm).
2.5.4. Measurement of ROS generation
The level of intracellular reactive oxygen species (ROS) was estimated with DCF-DA probe on fluorescence spectrophotometer [Citation24]. MCF-7 cells (1 × 105) were inoculated in a 96-well plate and the seeded cells were exposed to IC50 doses of GSRE (55.30 µg/mL), TiO2 NPs (46.64 µg/mL) for 12 h. The cells were incubated with the addition of 25 μM DCFH-DA at 37 °C for 30 min. After that, the treated cells were washed with PBS and visualised using a fluorescent microscope (Nikon Eclipse TS 100). Qualitative percentage of intracellular ROS generation in the treated cells was compared to the untreated control cells.
2.5.5. Evaluation of mitochondrial membrane potential (MMP)
In this study, mitochondrial membrane potential (MMP) was detected using fluorescent rhodamine 123 (Rh 123) dye. MCF-7 cells were inoculated in 96-well plates (1 × 105) and the seeded cells were treated with the IC50 doses of GSRE (55.30 µg/mL) and TiO2 NPs (46.64 µg/mL) for 24 h. After incubation, cells were stained with fluorescent dye Rhodamine 123 (5 mmol/mL) for 15 min [Citation25]. Later, the stained cells were soaked with PBS and the cells formation noticed under the fluorescence microscope. Orange red luminance indicated polarised mitochondrion and green fluorescence noticed depolarised mitochondrion.
2.5.6. Comet assay
DNA fragmentation was check out by COMET assay by way of Tice et al. [Citation26]. The cancer cells were seeded in 96 well plates and exposure with IC50 doses of GSRE (55.30 µg/mL) and TiO2 NPs (46.64 µg/mL) followed by overnight incubation. Both normal (control) and MCF-7 cells were rinsed twice with PBS (ice-cold) and centrifuged for 5 min at 1200 rpm. Finally, cell suspension (100 μL) having 1 × 105 cells were mixed with 0.75% agarose (900 μL). The cell-gel sandwich was enclosed using a coverslip and kept on 4 °C for 10 min in solidification process. After incubation, the slides were dipped in chilled lysis solution (10 mM Tris, 2.5 M NaCl, 1% Triton X-100, 100 mM EDTA and maintained pH 10) for 1 h at 4 °C. Afterward, slides were immersed for 20 min in electrophoresis buffer (1 mM EDTA, 300 mM NaOH and maintained pH 13) to DNA relaxing before electrophoretic analysis. Electrophoresis was done at 25 V (300 mA) for 30 min. To prevent extra DNA damage, all the stages were done under low light condition. Afterwards, the slides were nullified using Tris (0.4 M) at pH 7.5, then the slides washed and stained using 2.5 μg/mL of propidium iodide and allow to dry. After drying, the gels were kept at room temperature for overnight and the gels were examined with a fluorescence microscope. The range of DNA destructions (head DNA, TL, TM and OTM) were determined in the normal (untreated), GSRE and TiO2 NPs treated cells.
2.6. Influence of biosynthesised nanotitania catalysts on a photocatalytic test
The photocatalytic test of nanotitania catalysts was assessed to degrade various dyes under sunlight irradiation. Stock solutions were prepared by biosynthesised TiO2 NPs (10 mg) dispersed in 50 mL of various dye solutions (methylene blue, methyl orange, alizarin red and crystal violet) [Citation9]. The reaction mixture was agitated for 30 min, then the solution open to sunlight and checked at distinct intervals (0, 1, 2, 3, 4, 5 and 6 h). An aliquot of 2 mL reaction mixture was centrifuged for 15 min at 8000 rpm and the supernatant was used to calculate the dye degradation activity. Supernatants of all the dyes were collected and measured at different wavelengths by using UV–visible spectroscopy (Shimadzu, UV-1800). The photocatalytic dye degradation percentage was calculated using this formula:
where C0 is the initial concentration and C is the concentration of after photocatalytic degradation.
2.7. Statistical analysis
Data were statistically explored using one-way ANOVA using post hoc Duncan’s test. Values were reflected as a significant difference between treated and control tests. All the data were presented as the mean ± standard error of three experiments. All the tests were designed using SPSS software version 20.0 (SPSS Inc., Chicago, IL, USA).
3. Results and discussion
3.1. Biosynthesis and characterization of titanium dioxide nanoparticles (TiO2·NPs)
Eco-friendly biological synthesis of nanoparticles often plays a significant role in nanotechnology. In the current study, TiO2 NPs synthesised from aqueous 5 mM TiO(OH)4 solution with rhizome extracts of G. superba was achieved under bright light conditions for 24 h. The synthesised TiO2 NPs was assessed by UV–vis spectroscopy (SHIMADZU-1800) in ranging from 200 to 800 nm. Due to the agitation of surface plasma resonance (SPR) oscillations, the absorption band was detected at 320 nm. The UV–visible absorption peak indicates the formation of TiO2·NPs in the reaction mixture [Citation27]. FTIR analysis of both the rhizome extract and synthesised TiO2·NPs was performed to identify the potential biomolecules respond as a stabilising agent for the production of TiO2 NPs. The FTIR chromatic spectrum was assimilated in the ranging from 4000 to 400 cm−1. Absorption spectra at 3737 and 3418 cm−1 were associated to the presence of alcohol O–H group. The spectrum peaks at 2922, 2855 cm−1 correspond to the alkane groups C–H bridge mode of fluctuation. The weak absorption peak at 2376 cm−1 could be accredited to P–H phosphine sharp and the peak at 2088 cm−1 correlate to N = C in R–N = C=S bridging vibrations. The absorption peak at 1637 cm−1 attributed to C = C stretching fluctuations of alkenes group. The peak at 1425 cm−1 C–C bridging vibrations of aromatics. The peak which occurs at 1246 cm−1 be permitted due to C–O–H curving vibrations and the outstanding peak at 1025 cm−1 correlate to C–O stretch (). TiO2 NPs synthesised using rhizome extract of G. superba with strong bands at 3740 cm−1 (O–H stretch) alcohol group, 3280 cm−1 (C–H stretch), 2924 cm−1 (CH2 stretch), 2284 cm−1 P–H phosphine sharp, 1645 cm−1 (C = O stretch), 1541 cm−1 NH out of plane, 1450 cm−1 (CH2 and CH3) alkanes, 1343 cm−1 (S = O sulfonic acid), 1110, 1057 cm−1 (Si–OR broad), 922 cm−1 (RCOOH O–H bend) and 754, 693 cm−1 (C–H out of plane) groups were identified (). By comparison of both the spectrum (), the peak at 3418 cm−1 corresponding stretching vibrations of alcohol O–H group of G. superba, rhizome extracts transfer noticeably to lower wavelength 3280 cm−1 which indicates the binding of TiO2 nanoparticles to the surface. This evidences that the plant-derived bioactive chemicals were presumed to be convoluted in the capping and stabilising of the synthesised TiO2 NPs.
Figure 1. FT-IR spectral analysis of (a) aqueous rhizome extract of G. superba and (b) biosynthesised nanotitania catalysts using G. superba rhizome extract.
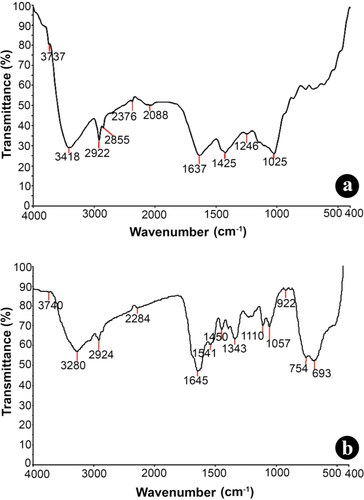
The crystal clear quality of the biosynthesised TiO2 nanoparticles was further validated by the characteristic peaks observed in the XRD analysis (). The intense peaks for the synthesised TiO2 NPs were noticed for 2θ values of 25.14°, 37.90°, 47.88°, 53.73°, 54.93°, 62.53°, 70.20°, 74.96° and 82.41° corresponding planes (101), (103), (200), (105), (213), (116), (107) and (303), towards the anatase tetragonal lattice of titanium consistent with the data of Joint Committee on Powder Diffraction (JCPD) accepted file no. 01-0562. The XRD pattern clearly illustrates that the TiO2 NPs were crystal in nature. The morphology and width of the synthesised TiO2 NPs were identified by FE-SEM. The FE-SEM images of TiO2 NPs clearly indicate the particles were dispersed and complete aggregation with a spherical shape. The width of the synthesised nanoparticles was arranged between 20 and 100 nm (). Recently, Keerthika et al. [Citation9] proved the synthesised TiO2 NPs displayed aggregation with a spherical in shape. EDX analysis of the synthesised TiO2 NPs displays specific peaks for Ti and oxygen ions. The EDX results showed a strong peak of titanium ion at 4–5 KeV and also observed other peaks of carbon and silicon elemental signals (). It is presumed that the presence of biomolecules in the surface of the biosynthesised TiO2 NPs efficacy involved in the reduction of nanoparticles. Similar observations were described earlier using various plant species such as Parthenium hysterophorus [Citation9], Calotropis gigantean [Citation28] and Psidium guajava [Citation29].
3.2. Antibacterial efficacy of the biosynthesised nanotitania catalysts
Antibacterial activity of synthesised TiO2 NPs and rhizome extracts of G. superba against six isolated human pathogens are presented in . The antibacterial activity of nanotitania catalysts and rhizome extracts was assessed based on the presence of zone formation over the discs (). For antibacterial activity, antibiotic (streptomycin), aqueous rhizome extract and two concentrations (25 and 50 μg) of synthesised nanotitania catalysts were used and the nanotitania catalysts displayed a vigorous antibacterial activity by producing the maximum level of suppression zone. Of the two concentrations tested, TiO2 NPs at 50 μg showed the largest zone of growth inhibition when compared to rhizome extract. The results showed such a potential antibacterial activity was noticed against S. epidermidis (27 mm) followed by P. aeruginosa (20 mm). Furthermore, results suggest that the gram-positive bacteria seemed to be more resistant to TiO2 NPs then gram-negative bacteria. The maximum growth inhibition was recorded for S. epidermidis and P. aeruginosa. This result is in acknowledging with previous reports on the antibacterial activeness of varied plant extracts P. hysterophorus [Citation9], Euphorbia hirta [Citation10] and Prosopis cineraria [Citation16]. Similarly, Tahir et al. [Citation30] also reported the synthesis of palladium nanoparticles from Sapium sebiferum leaf extract showed modest antibacterial action against P. aeruginosa. The TiO2 NPs were synthesised using different methods and they showed efficient antibacterial activity against Salmonella typhimurium, E. coli, S. aureus and K. pneumonia [Citation31, Citation32]. The antibacterial results strongly suggested that synthesised nanotitania catalysts exhibited potential activity and it can be considered as replacements of antibiotics in drug therapeutics.
3.3. Impact of nanotitania catalysts on inhibition of antibiofilm formation
The synthesised TiO2 NPs were determined against P. aeruginosa and S. epidermidis by antibiofilm activity. The antibiofilm activity clearly correspond with nanoparticles dose and the dosage effect was listed for both nanoparticles and plant extract. The maximum rate of anti-biofilm action recorded was 27.5% at 50 µg/mL TiO2 NPs treatment with S. epidermidis. In case of P. aeruginosa, the highest rate of biofilm inhibition noticed was 30.8% at 50 µg/mL TiO2 NPs exposure. The inhibition of biofilm formation was found to be high in TiO2 NPs when compare to rhizome extract of G. superba (). Recently, Prasannaraj and Venkatachalam [Citation23] reported the enhanced antibiofilm activity using AgNPs synthesised using several plant extracts. This result suggested that nanotiania catalysts could be used as a valuable substitute for antifouling agent. Biofilms are the aggregation of surface-attached in cells that are fixed in self-made extracellular polymeric substances (EPS). The EPS consists of nucleic acid, polysaccharides and polypeptides [Citation33, Citation34]. The effect of MNPs-doped matrix was performed to impede the biofilm deposit [Citation35]. Similar inhibitory effect of TiO2 NPs against P. aeruginosa was reported earlier by Vincent et al. [Citation36]. Kalishwaralal et al. [Citation37] also reported enlarging anti-biofilm activity of AgNPs from Bacillus licheniformis.
Table 1. Effect of different doses of nanotitania catalysts and rhizome extract of G. superba on anti-biofilm activity in S. epidermidis and P. aeruginosa.
3.4. Influence of nanotitania catalysts on anticancer activity
3.4.1. Cytotoxicity of the synthesised nanotitania catalysts
The cytotoxic effects of G. superba rhizome extract (GSRE) and TiO2 NPs were investigated against MCF-7 and L929 (breast cancer and mouse fibroblast normal cells) by MTT assay. The synthesised nanotitania catalysts were assessed for their cytotoxic effects on cell viability using MCF-7 and L929 cell lines at a different dosage of (6.25, 12.5, 25, 50 and 100 µg/mL). shows the cytotoxic effects of GSRE and nanotitania catalysts against the MCF-7 cell line. In the present study, a dose dependent apoptotic activity was determined in GSRE and nanotitania catalysts treated MCF-7 and normal L929 cell lines. The 50% inhibitory concentration (IC50) value for biosynthesised TiO2 NPs against MCF-7 and L929 cell lines recorded was 46.64 and 61.81 µg/mL (), respectively. The nanotitania catalysts did not disturb the normal L929 cell lines growth at a lower concentration. Interestingly, the cytotoxicity against cancer cell line was increased with increasing the concentrations of nanotitania catalysts in the medium. Earlier, Murugan et al. [Citation5] reported the cytotoxic effect of TiO2 nanoparticles against MCF-7 cells at 60.00 μg/mL dose. Recently, the cytotoxic effect of TiO2 nanoparticles against MCF-7 cells was described by Remya et al. [Citation38] and Lotfian and Nemati [Citation39]. The present results strongly indicate that the synthesised nanotitania catalysts have a higher apoptotic activity of MCF-7 cancer cell line compared to control.
3.4.2. Acridine orange and ethidium bromide (AO/EtBr) and Hoechst staining to detect apoptosis
The inception of apoptosis shows a significant appearance in anticancer drug delivery. Commercial anticancer remedy has the potential to generate apoptosis in affected (cancer) cells. After AO/EtBr staining, the morphological view of the apoptotic, necrotic and regular cells of MCF-7 was diagnosed independently using fluorescence microscopy. In order to attempt the hypothesis whether the inhibitory action of the TiO2 NPs on MCF-7 cancer cell line was due to the apoptosis or not, the synthesised nanotitania was exposed to cancer cell lines and studied its effect using staining methods. Cell death was observed with cell pattern and chromatin condensation. Such, modifications appear due to the stimulation of caspase cascades, in which dissect the exact substrates liable for the DNA repair activation. Nanotitania catalysts (46.64 µg/mL) and GSRE (55.30 µg/mL) treated cells were collected and measured the cell morphology after AO/EtBr staining. The smear cells were identified by live cells in light green colour, initial apoptotic cells, belate apoptotic cells in orange colour and almost death cells in red coloured. Orange coloured cells have proapoptotic shape, also red coloured cells contain dead bodies found in the nano catalysts (46.64 µg/mL) treated MCF-7 cells after 24 h exposure (). Whereas, the cancer cells indicated a green colour that displays the presence of live cells in control cells (). Microscopic identification showed that the regular cells appeared to be a green colour for the binding of AO into cell membranes. Although, proapoptotic cells were detected as orange coloured forms due to the shrinking of nuclei and nuclear blubbing for the stimulation of EtBr into cells. However, no more cells were turned toward red colour luminance due to their damage of membrane reliability by nanotitania catalyst exposure. It was witnessed that the level of dead cells was found to be high in nanotitania catalysts treated MCF-7 cells compared to the control cells.
Figure 6. Fluorescence microscopy images of AO/EtBr stained individual control and nanotitania catalysts treated MCF-7 cells, (a) control (untreated) cells, (b) G. superba rhizome extract and (c) nanotitania catalysts treated cells. The arrows are denoted by green colour (live cells), orange colour (proapoptotic cells) and red colour (dead cells). Hoechst nuclear staining images of control cells (d) and arrows indicate nuclear materials in apoptotic cells (e, f). The white scale bars are 10 µm.
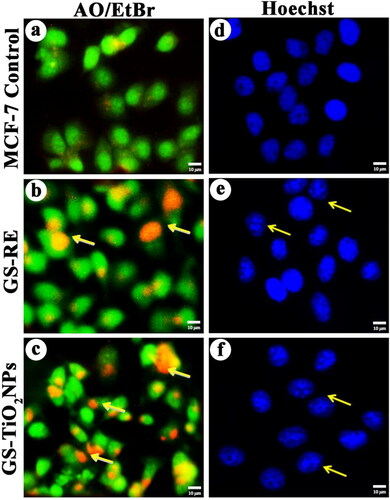
Furthermore, nuclear morphology of the nanotitania catalysts (46.64 µg/mL) and GSRE (55.30 µg/mL) treated MCF-7 cells were noticed under fluorescence microscopy after Hoechst 33258 staining. MCF-7 cells initiate to apoptotic characteristics such as cell shrinking, nuclear damage after exposure of nanotitania catalysts at 46.64 µg/mL for 24 h, () while, the control cells displayed regular nuclei (smooth) (). Selvi et al. [Citation40] also described AgNPs synthesised using seaweed extracts of Padina tetrastromatica which showed apoptosis in MCF-7 cell line.
3.4.3. Nanotitania catalysts-induced apoptosis of MCF-7 cancer cells due to reactive oxygen species (ROS) generation and mitochondrial membrane potential (MMP)
The level of ROS generation from GSRE (55.30 µg/mL) and nanotitania (46.64 µg/mL) exposed MCF-7 cells were determined. The DCFH-DA method was adopted to measure the intracellular formation of hydrogen peroxide which is an indirect measure for estimating ROS generation. In the present experiment, the generation of intracellular ROS was found to be significantly higher in nanotitania catalysts (46.64 µg/mL) treated MCF-7 cells when compared to control cells (). Such, ROS generation can stimulate cell death by two specific pathways like apoptosis and necrosis (). Cui et al. [Citation41] revealed that the metal and metal oxide nanoparticles exposure increased the level ROS and decreased the mitochondria membrane potential which indicates the apoptotic cell death induced by nanoparticles. Metal nanoparticles have intensively associated with cells and intracellular macromolecules (protein and DNA). Cellular uptake of nanoparticles has the generation of ROS which indicates the generation of oxidative stress within the cancer cell. It is presumed that the nanoparticles may cross the nuclear membrane to interact with DNA which ultimately disturbed the DNA function, but the exact mechanism for such interaction is yet to be identified [Citation42].
Figure 7. Effects of G. superba rhizome extract and nanotitania catalysts (IC50 concentration) on intracellular ROS generation in treated MCF-7 cells, (a) Control cells, (b, c) rhizome extract and nanotitania catalysts treated cells. Rhodamine mitochondrial staining images of (d) control cells, (e, f) Rhizome extract and nanotitania catalysts exposed cells showed compressed form of mitochondrial membrane The white scale bars are 10 µm.
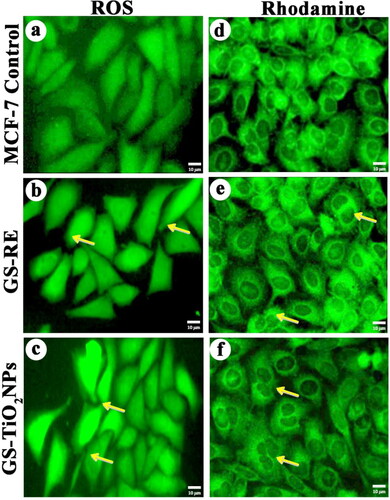
The effect of nanotitania catalysts on mitochondrial membrane potential was determined by rhodamine 123 dye staining method. After nanotitania catalysts treatment, live cells contain higher polarity, whereas dead cells drop such polarity for the destruction of mitochondrial membrane reliability, thus, exhibit a suspension of the dye [Citation43]. Higher mitochondrial depolarization was found to be nanotitania catalysts (46.64 µg/mL) treated MCF-7 cells over GSRE (55.30 µg/mL) and control cells (f)). Such changes in mitochondrial membrane potential could be associated to the constant release of nanotitania catalysts into MCF-7 cancer cells. Recently, Ramalingam et al. [Citation44] have revealed that the treatment with PVP-AuNPs has increased the mitochondria depolarization. Exposure of PVP-AuNPs could enhance the intracellular ROS generation in lung cancer cell lines including A549, H460 and H520 when compare to free Dox, Dox@PVP-AuNPs and control cells. Similarly, the effect of TiO2 nanoparticles towards damage to the mitochondria was also reported earlier [Citation45].
3.4.4. Comet assay
The nanotitania catalysts (46.64 µg/mL) treated MCF-7 cancer cells exhibited the DNA breakup in term of tail DNA (TD), DNA tail length (TL), DNA tail moment (TM) and olive tail moment (OTM) development. Otherwise, no such change was detected in untreated cancer cells (). Nanotitania catalysts exposure had automatically increased the structure of TD, TL, TM and OTM in MCF-7 cells (). It is assumed that the DNA destruction was due to the generation of free radicals by nanoparticles exposure. It is reported that nanoparticles exposure generally triggered the DNA damage and olive tail DNA movement which was identified in the current experiment. Excess of ROS generation impacted the DNA break important to cell death induced by nanotitania catalysts exposure. Similarly, Jeyaraj et al. [Citation46] also described that the effect of AgNPs on DNA disturbance was determined in circumstances of tail length, tail formation and tail moment in MCF-7 cells.
3.5. Effect of the synthesised nanotitania on photocatalytic activity
The photocatalytic activity against various dyes namely, crystal violet (CV), methylene blue (MB), alizarin red (AR) and methyl orange (MO) were investigated under sunlight for different time intervals (). The distinctive absorption peaks were observed at 646 nm (MB), 464 nm (MO), 520 nm (AR) and 580 nm (CV). Results show that the decolorisation efficiency of four different dyes was gradually increased with irradiation time. However, the per cent of decolorisation by nanotitania catalysts was found to be high in CV (98.3%) followed by AR (98.0%), MB (75.75%) and MO (68.45%) within 6 h of solar irradiation exposure (). Recently, Keerthika et al. [Citation9] and Jinu et al. [Citation10] observed the maximum dye degradation activity with MB and CV after exposure to TiO2 NPs. The solar light irradiation was found to be fast decolorising system compared to other irradiation techniques [Citation47]. Similarly, Ag and ZnO nanoparticles synthesised using various plant extracts acted as efficient photocatalysts to degrade the toxic organic dyes [Citation48, Citation49].
4. Conclusions
In this study, an efficient method for biological fabrication of TiO2 NPs using G. superba aqueous rhizome extract was developed and studied their potential role towards antibacterial, antibiofilm, anticancer and photocatalytic activities. Nanotitania catalysts showed efficient antibacterial activity against various virulence human microbial pathogens. The green synthesised nanotitania catalysts and rhizome extracts exhibited efficient and dose-dependent response against MCF-7 cells and normal L929 cells. The stimulation of apoptosis in breast cancer cell line was displayed by the AO/EtBr staining assay and the rate of apoptotic bodies was found to be high in nanotitania catalysts exposed cells. Nuclear staining by Hoechst 33258 has resulted in bright blue colour emission in the nanotitania catalysts treated MCF-7 cells. The occurrence of oxidative stress induced by nanotitania catalysts treated cancer cell lines was confirmed by ROS detection. The measurement of mitochondrial membrane potential in the nanotiania catalysts exposed MCF-7 cell line was recorded by rhodamine staining technique and assessment of DNA damage was determined by COMET. Also, the nanotitania catalysts show efficient photocatalytic dye degradation activity against methylene blue, alizarin red, methyl orange and crystal violet. The fabrication of nanotitania catalysts using rhizome extract of G. superba and its antibacterial, antibiofilm, anticancer and photocatalytic activities was reported for the first time. Present results strongly propose that the biomolecule wrapped nanotitania catalysts could be used as a promising nano-drug to treat cancer cells and also used to remediate the polluted environment by toxic dyes in the near future.
Acknowledgements
Dr. P. Venkatachalam gratefully acknowledges the Council of Scientific and Industrial Research (CSIR), New Delhi for financial assistance provide under the CSIR Major Research Project, No. 38(1324)/12 EMR-II. DST-FIST (SR/FIST/LSI-673/2016) is gratefully acknowledged for financial assistance to the Department of Biotechnology, Periyar University.
Disclosure statement
No potential conflict of interest was reported by the authors.
References
- Park Y, Hong YN, Weyers A, et al. Polysaccharides and phytochemicals: a natural reservoir for the green synthesis of gold and silver nanoparticles. IET Nanobiotechnol. 2011;5(3):69–78.
- Palaniyandi V, Jayabrata D, Raman P, et al. Greener approach for synthesis of antibacterial silver nanoparticles using aqueous solution of neem gum (Azadirachta indica L.). Ind Crops Prod. 2015;66:103–109.
- Andrea G, Seokju S, Sung HJ, et al. Effects of titanium dioxide nanoparticles derived from consumer products on the marine diatom Thalassiosira pseudonana. Environ Sci Pollut Res Int. 2016;23(20):21113–21122.
- Prakash T, Navaneethan M, Archana J, et al. Synthesis of TiO2 nanoparticles with mesoporous spherical morphology by a wet chemical method. Mater Lett. 2012;82:208–210.
- Murugan K, Dinesh D, Paulpandi M, et al. Mangrove helps: Sonneratia alba-synthesized silver nanoparticles magnify guppy fish predation against Aedes aegypti young instars and down-regulate the expression of envelope (E) gene in dengue virus (serotype DEN-2). J Clust Sci. 2017;28(1):437–461.
- Noruzi M. Biosynthesis of gold nanoparticles using plant extracts. Bioprocess Biosyst Eng. 2015;38(1):1–14.
- Kumar V, Yadav SK. Plant-mediated synthesis of silver and gold nanoparticles and their applications. J Chem Technol Biotechnol. 2009;84(2):151–157.
- Benelli G. Plant-mediated biosynthesis of nanoparticles as an emerging tool against mosquitoes of medical and veterinary importance: a review. Parasitol Res. 2016;115(1):23–34.
- Keerthika T, Manikandan K, Elangovan N, et al. Enhanced larvicidal, antibacterial, and photocatalytic efficacy of TiO2 nanohybrids green synthesized using the aqueous leaf extract of Parthenium hysterophorus. Environ Sci Pollut Res. 2018;25(11):10328–10339.
- Jinu U, Vaitheeswari K, Manish T, et al. Nanotitania crystals induced efficient photocatalytic color degradation, antimicrobial and larvicidal activity. J Photochem Photobiol B: Biol. 2018;178:496–504.
- Ahmad R, Sardar M. TiO2 nanoparticles as an antibacterial agents against E. coli. Int J Innov Sci Eng Technol. 2013;2(8):3569–3574.
- Othman SH, Abd Salam NR, Zainal N, et al. Antimicrobial activity of TiO2 nanoparticle-coated film for potential food packaging applications. Int J Photoenerg. 2014;2014:1–6.
- Quintana M, Edvinsson T, Hagfeldt A, et al. Comparison of dyesensitized ZnO and TiO2 solar cells: Studies of charge transport and carrier lifetime. J Phys Chem C. 2007;111(2):1035–1041.
- Han F, Kambala VSR, Srinivasan M, et al. Tailored titanium dioxide photocatalysts for the degradation of organic dyes in wastewater treatment: a review. Appl Catal A: Gen. 2009;359(1–2):25–40.
- Chang S, Liu W. The roles of surface-doped metal ions (V, Mn, Fe, Cu, Ce, and W) in the interfacial behavior of TiO2 photocatalysts. Appl Catal B: Environ. 2014;156–157:466–475.
- Jinu U, Gomathi M, Saiqa I, et al. Green engineered biomolecule-capped silver and copper nanohybrids using Prosopis cineraria leaf extract: enhanced antibacterial activity against microbial pathogens of public health relevance and cytotoxicity on human breast cancer cells (MCF-7). Microb Pathog. 2017;105:86–95.
- Nagajyothi PC, Muthuraman P, Sreekanth TVM, et al. Green synthesis: In-vitro anticancer activity of copper oxide nanoparticles against human cervical carcinoma cells. Arab J Chem. 2017;10(2):215–225.
- Padmapriya S, Rajamani K, Sathiyamurthy VA. Glory Lily (Gloriosa superba L.) - A Review. Int J Curr Pharmaceut Res. 2015;7:43–49.
- Khan H, Khan MA, Mahmood T, et al. Antimicrobial activities of Gloriosa superba Linn. (Colchicaceae) extracts. J Enzyme Inhib Med Chem. 2008;23(6):855–859.
- Gopinath K, Karthika V, Gowri S, et al. Antibacterial activity of ruthenium nanoparticles synthesized using Gloriosa superba L. leaf extract. J Nanostruc Chem. 2014;4(1):83.
- Raja Naika H, Lingaraju K, Manjunath K, et al. Green synthesis of CuO nanoparticles using Gloriosa superba L. extract and their antibacterial activity. J Taibah University Sci. 2015;9(1):7–12.
- Arumugam A, Karthikeyan C, Hameed ASH, et al. Synthesis of cerium oxide nanoparticles using Gloriosa superba L. leaf extract and their structural, optical and antibacterial properties. Mater Sci Eng C Mater Biol Appl. 2015;49:408–415.
- Prasannaraj G, Venkatachalam P. Enhanced antibacterial, anti-biofilm and antioxidant (ROS) activities of biomolecules engineered silver nanoparticles against clinically isolated gram positive and gram negative microbial pathogens. J Clust Sci. 2017;28(1):645–664.
- Nataraj J, Manivasagam T, Justin Thenmozhi A, et al. Neuroprotective effect of asiatic acid on rotenone-induced mitochondrial dysfunction and oxidative stress-mediated apoptosis in differentiated SH-SYS5Y cells. Nutr Neurosci. 2017;20(6):351–359.
- Halliwell B, Whiteman M. Measuring reactive species and oxidative damage in vivo and in cell culture: how should you do it and what do the results mean? Br J Pharmacol. 2004;142(2):231–255.
- Tice RR, Agurell E, Anderson D, et al. Single cell gel/comet assay: guidelines for in vitro and in vivo genetic toxicology testing. Environ Mol Mutagen. 2000;35(3):206–221.
- Sankar R, Rizwana K, Shivashangari KS, Ravikumar V. Ultra-rapid photocatalytic activity of Azadirachta indica engineered colloidal titanium dioxide nanoparticles. Appl Nanosci. 2015;5(6):731–736.
- Marimuthu S, Rahuman AA, Jayaseelan C, et al. Acaricidal activity of synthesized titanium dioxide nanoparticles using Calotropis gigantea against Rhipicephalus microplus and Haemaphysalis bispinosa. Asian Pac J Trop Med. 2013;6(9):682–688.
- Santhoshkumar T, Rahuman AA, Jayaseelan C, et al. Green synthesis of titanium dioxide nanoparticles using Psidium guajava extract and its antibacterial and antioxidant properties. Asian Pac J Trop Med. 2014;7(12):968–976.
- Tahir K, Nazir S, Li B, et al. Sapium sebiferum leaf extract mediated synthesis of palladium nanoparticles and in vitro investigation of their bacterial and photocatalytic activities. J Photochem Photobiol B. 2016;164:164–173.
- Rajakumar G, Rahuman AA, Roopan SM, et al. Fungus-mediated biosynthesis and characterization of TiO2 nanoparticles and their activity against pathogenic bacteria. Spectrochim Acta A Mol Biomol Spectrosc. 2012;91:23–29.
- Hassan MS, Amna T, Mishra A, et al. Fabrication, characterization and antibacterial effect of novel electrospun TiO2 nanorods on a panel of pathogenic bacteria. J Biomed Nanotechnol. 2012;8(3):394–404.
- Perez-Diaz M, Alvarado-Gomez E, Magaña-Aquino M, et al. Anti-biofilm activity of chitosan gels formulated with silver nanoparticles and their cytotoxic effect on human fibroblasts. Mater Sci Eng C Mater Biol Appl. 2016;60:317–323.
- Du J, Singh H, Yi TH. Antibacterial, anti-biofilm and anticancer potentials of green synthesized silver nanoparticles using benzoin gum (Styrax benzoin) extract. Bioprocess Biosyst Eng. 2016;39(12):1923–1931.
- Chapman J, Weir E, Regan F. Period four metal nanoparticles on the inhibition of biofouling. Colloids Surf B Biointerfaces. 2010;78(2):208–216.
- Vincent MG, John NP, Narayanan PM. In vitro study on the efficacy of zinc oxide and titanium dioxide nanoparticles against metallo beta-lactamase and biofilm producing Pseudomonas aeruginosa. J Appl Pharmaceut Sci. 2014;4(7):41–46.
- Kalishwaralal K, BarathManiKanth S, Pandian SRK, et al. Silver nanoparticles impede the biofilm formation by Pseudomonas aeruginosa and Staphylococcus epidermidis. Colloids Surf B Biointerfaces. 2010;79(2):340–344.
- Remya RR, Rajasree SR, Aranganathan L, Suman TY. An investigation on cytotoxic effect of bioactive AgNPs synthesized using Cassia fistula flower extract on breast cancer cell MCF-7. Biotechnol Rep (Amst)). 2015;8:110–115.
- Lotfian H, Nemati F. Cytotoxic effect of TiO2 nanoparticles on breast cancer cell line (MCF-7). Iioab J. 2016;7:219–224.
- Selvi BCG, Madhavan J, Amutha S. Cytotoxic effect of silver nanoparticles synthesized from Padina tetrastromatica on breast cancer cell line. Adv Nat Sci: Nanosci Nanotechnol. 2016;7(3):035015.
- Cui H, Kong Y, Zhang H. Oxidative stress, mitochondrial dysfunction, and aging. J Signal Transd. 2012;2012:1–13.
- Molina MAF, Gamboa EM, Rivera CAS, et al. Antitumor activity of colloidal silver on MCF-7 human breast cancer cells. J Exp Clin Cancer Res. 2010;29(1):148.
- Tan Y, Yu R, Pezzuto JM. Betulinic acid-induced programmed cell death in human melanoma cells involves mitogenactivated protein kinase activation. Clin Cancer Res. 2003;9(7):2866–2875.
- Ramalingam V, Varunkumar K, Vilwanathan K, et al. Target delivery of doxorubicin tethered with PVP stabilized gold nanoparticles for effective treatment of lung cancer. Sci Rep. 2018;8:3815.
- Braydich-Stolle LK, Schaeublin NM, Murdock RC, et al. Crystal structure mediates mode of cell death in TiO2 nanotoxicity. J Nanopart Res. 2009;11(6):1361–1374.
- Jeyaraj M, Sathishkumar G, Sivanandhan G, et al. Biogenic silver nanoparticles for cancer treatment: An experimental report. Colloids Surf B Biointerfaces. 2013;106:86–92.
- Kansal SK, Singh M, Sud D. Studies on TiO(2)/ZnO photocatalysed degradation of lignin . J Hazard Mater. 2008;153(1–2):412–417.
- Cittrarasu V, Balasubramanian B, Kaliannan D, et al. Biological mediated Ag nanoparticles from Barleria longiflora for antimicrobial activity and photocatalytic degradation using methylene blue. Artif Cells Nanomed Biotechnol. 2019;47(1):2424–2430.
- Azarang M, Shuhaimi A, Yousefi R, et al. Synthesis and characterization of ZnO NPs/reduced graphene oxide nanocomposite prepared in gelatin medium as highly efficient photo-degradation of MB. Ceram Int. 2014;40(7):10217–10221.