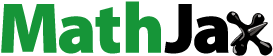
Abstract
This study aimed to synthesize Melphalan (MEL)-loaded PEGylated nanoliposomes and evaluate their cytotoxic effects on ovarian cancer A2780CP and SKOV3 cells. For this purpose, MEL-loaded PEGylated nanoliposomes were prepared by the reverse-phase evaporation method. They were then characterized, and the results showed that the size, size distribution, and zeta potential of the nanoformulation were 129.1 ± 5.5 nm, 0.240 ± 0.13, and −18.9 0.31 mv, respectively. Also, the encapsulation and loading efficiencies of MEL in the nanoparticles were determined to be 98.3 ± 2.1% and 5.2 ± 1.3%, respectively. Also, the drug release pattern was evaluated using a dialysis bag method, and the results demonstrated that 20.5% of MEL was released after 48 h from the nanoparticles, which was 2.7-fold slower compared to when the standard drug was used. In addition, the effects of the standard drug and nanoformulation on cell viability was assessed using a 3-(4,5-dimethylthiazol-2-yl)-2,5-diphenyltetrazolium bromide (MTT) assay, and the results showed that nanoformulation had 1.4-fold more toxicity than the standard drug. Moreover, the extent of apoptosis was investigated using the Annexin- propidium iodide kit and flow cytometry method. The results showed that MEL-loaded PEGylated nanoliposomes increased the apoptotic cells compared to the control group.
1. Introduction
Cancer is considered as the second leading cause of death after cardiovascular diseases worldwide. Ovarian cancer is one of the most important and deadly cancers that is the cause of a significant number of deaths worldwide [Citation1,Citation2]. In the United State, approximately 22,240 new cases of ovarian cancer were diagnosed in which 14,070 deaths were reported [Citation3]. Although most cancerous tumors often spread through the bloodstream, ovarian cancer spreads through the spread of cancer cells from the primary tumor into the abdominal cavity [Citation4].
There are various approaches, such as chemotherapy, radiotherapy, and surgery, for the treatment of cancers [Citation5]. Chemotherapy, radiotherapy, and surgery are some of the most common treatments for ovarian cancer. Since anticancer drugs are not an option in chemotherapy, high doses of these drugs are often needed to achieve the desired level of treatment in tumor tissues. Also, as anti-neoplastic drugs have a high molecular weight and a high volume of distribution, the use of high doses of the drugs causes healthy tissues to be exposed to these drugs, and thus, the toxicity of the drug increases [Citation6]. In addition, using anti-neoplastic drugs to treat cancers is associated with a wide range of side effects and deficiencies, such as non-specificity, short half-life, and multi-drug resistance (MDR) [Citation6,Citation7]. MDR has been considered as one of the most important obstacles to successful chemotherapy. Therefore, to minimize these side effects and shortcomings, efforts have been made to develop targeted drug delivery systems.
Melphalan (MEL; Alkeran®) is an alkylating agent that stops tumor growth by cross-linking guanine bases in two strands of deoxyribonucleic acid (DNA) and a direct attack on DNA. It is used to treat a variety of cancers, e.g. multiple myeloma cancer, ovarian cancer, and breast cancer [Citation8]. In recent years, nanotechnology has been widely used to improve a variety of common treatments for cancer [Citation9]. Nanotechnology involves the design, synthesis, and description of materials and devices organized on a nanoscale in at least one dimension, and their characteristics are used to diagnose and treat diseases [Citation8,Citation10]. Among nano-based drug delivery systems, nanolipids, such as liposomes, have been successfully used in clinical applications, and most of them have been approved by the Food and Drug Administration (FDA) of the USA [Citation11]. Liposomes are phospholipid vesicles composed of a hydrophobic tail and a hydrophilic head and can encapsulate water- and lipid-soluble drugs in the liposome space and hydrophobic space, respectively [Citation12]. The structural components of the liposome are synthetic amphiphilic or phospholipids, which combine with sterol lipids, such as cholesterol, to affect membrane permeability. Thin-film hydration is the most common preparation for liposomes, in which lipid components are dissolved in an organic solvent, which is evaporated by rotational evaporation followed by the re-watering of the film in an aqueous solvent [Citation13]. Instability is one of the drawbacks of liposomes, as they are transferred to proteins in the blood and removed from the bloodstream by macrophages in the liver [Citation14]. Therefore, the circulation time of liposomes in the blood increases by covering their surface with hydrophilic polymers, e.g. polyethylene glycol (PEG), which is a non-toxic and FDA approved compound [Citation15]. PEGylated liposomes can be formulated with different molar ratios of their constituents to vary in size, composition, and charge. Ghaffari et al. synthesized cisplatin-loaded PEGylated nanoliposome using SPC80 (soybeanphospholipids with 75% phosphatidylcholine), cholesterol, 1,2-distearoyl-sn-glycero-3-phosphoethanolamine-N-[methoxy(polyethylene glycol)] (DSPE‐MPEG) (85:10:5 weight ratio), and evaluated the cytotoxicity of the nanoformulation on breast cancer MCF-7 cells. The results showed that nanoformulation with a size of 119.7 ± 2.1 nm and zeta potential of −26.03 ± 1.34 mv demonstrated 1.6-fold higher toxicity compared to the standard drug (half maximal inhibitory concentration (IC50) = 34.7 and 56.5 µg/mL, respectively) [Citation16].
The present study aimed to prepare MEL-loaded PEGylated nanoliposomes using the reverse-phase evaporation technique to evaluate their effects on the toxicity of ovarian cancer cell lines and also in order to improve the therapeutic index, reduce side effects, and increase the half-life of the drug in the bloodstream. The nanoformulation was then characterized, in terms of size, size distribution, zeta potential, drug loading and encapsulation efficiency, and drug release. Also, two ovarian cancer cells (A2780CP and SKOV3) were used to assess the effects of the formulation on cell viability using a 3-(4,5-dimethylthiazol-2-yl)-2,5-diphenyltetrazolium bromide (MTT) assay.
2. Materials and methods
2.1. Materials
Phosphatidylcholine, cholesterol, PEG-2000, and fetal bovine serum (FBS) were purchased from Sigma-Aldrich (Germany). Roswell Park Memorial Institute 1640 (RPMI-1640), Dulbecco's Modified Eagle's Medium (DMEM), and Annexin V-FITC Apoptosis Detection Kit (with propidium iodide (PI)) were from Invitrogen (Carlsbad, CA, USA). Chloroform, methanol, and isopropanol, and MTT were purchased from Merck Company (Germany). MEL Vial and phosphate buffer saline (PBS) were from CELON LAB Company (India) and Medicago Company (Sweden), respectively. A2780CP and SKOV3 ovarian cancer cell lines were obtained from the Cell Bank of Pasteur Institute of Iran, Tehran. All materials used in experiments were of analytical grade.
2.2. Preparation of Melphalan-loaded nanoliposomes
MEL-loaded PEGylated nanoliposomes were prepared using the reverse-phase evaporation technique. For this purpose, 177.63, 10.18, 26.31, 2.4 mg of phosphatidylcholine, cholesterol, PEG-2000, and MEL, respectively, were dissolved in 20 mL 0f methanol/chloroform (1:2 v/v) and stirred (300 RPM, room temperature) for 24 h to obtain a clear yellow solution. The organic solvents were evaporated using a rotary evaporator (Heidolph, Germany) and a thin film of lipid was formed. To ensure the removal of any organic solvent, the film was again dried with nitrogen gas. Next, 15 mL of PBS was added, stirred (300 RPM, room temperature), sonicated under an ultra-sonic ice bath with a frequency of 60 Hz for 5 min to obtain a milky solution, and homogenized (Heidolph, Germany) to reduce the size of the nanoliposomes and make them more uniform. A control (PEGylated nanoliposomes without drug) was also prepared using the previously mentioned method.
2.3. Characterization of Melphalan-loaded nanoliposomes
The mean size, size distribution, and zeta potential of the nanoparticles were determined using a Dynamic Light Scattering (DLS) (Zetasizer, Nano ZS3600, Malvern Instrument Ltd, UK). The surface shape of the nanoparticles was also observed by transmission electron microscopy (TEM) using a Japanese Hitachi Transmission Electron. For this purpose, 1 mg/mL of the nanoformulation was prepared in PBS, from which a drop was injected into the device, and the morphology of the nanoliposome on the sample was investigated by producing high-energy electron beams.
2.4. Determination of loading and encapsulation efficiency of drug
To determine the encapsulation and loading efficacies of the drug, 2 mL of MEL-loaded PEGylated nanoliposomes suspension, containing 0.32 mg of MEL, or its control sample were separately ultra-centrifuged (35,000 RPM, 45 min, 4 °C) (UCEN, Iran), and the optical absorption of the supernatant was read by a spectrophotometer (UV-160 IPC, Shimadzu, Japan) at 260 nm. The amount of the drug was then calculated using a standard curve. The following equations [Citation17] were used to calculate the encapsulation and loading efficiencies of MEL.
(1)
(1)
(2)
(2)
To obtain the standard curve, different concentrations of MEL were prepared, the optical absorption of the samples was read at 260 nm, and the curve was plotted as optical absorption versus concentration.
2.5. Drug release from nanoparticles
MEL was released from the nanoparticles using the dialysis bag method. For this purpose, 2 mL of MEL-loaded PEGylated nanoliposomes, containing 0.32 mg of the drug, control, and the free drug (0.32 mg) was individually transferred into three dialysis bags (cut-off 12 kDa, Sigma). The dialysis bags were immersed in 25 mL of PBS (pH = 7.4) and stirrer (150 RPM, 37 °C) for 48 h. At different time intervals (1, 2, 4, 8, 12, 24, and 48 h), 2 mL of PBS was taken and replaced with the fresh buffer. The amount of the released drug in PBS was then measured by spectrophotometry at 260 nm, and the drug release percentage was obtained using the standard curve.
2.6. Cell viability
A2780CP and SKOV3 ovarian cancer cells and an MTT assay were used to investigate and compare the effects of MEL and MEL-loaded PEGylated nanoliposomes. For this purpose, 104 of A2780CP and SKOV3 cells were seeded in 96-well plates in RPMI-1640 and DMEM supplemented with 10% FBS, respectively, and incubated (5% CO2, 37 °C) for 24 h. The supernatant was then removed, and the cells were treated with MEL and MEL-loaded PEGylated nanoliposomes at the drug concentrations of 4.25, 8.5, 17, 34, and 68 µg/mL and incubated (5% CO2, 37 °C) for 24, 48, and 72 h. The supernatant was then discarded, 100 µL of MTT solution (0.5 mg/mL in PBS) was added to each well, and incubated for 4 h (5% CO2, 37 °C). The MTT solution was replaced with 100 μL of isopropanol and the absorbance was read at 570 nm using ELIZA Reader (Bio Tek. Instrument, USA). The cell viability and IC50 were then calculated using the following equations [Citation17]:
(3)
(3)
(4)
(4)
where the background is media alone, the negative control is the cells treated with media only, Y is inhibition percentage, and x is concentration.
2.7. Apoptosis analysis
To investigate apoptosis in A2780CP and SKOV3 cells, according to the manufacturer's instructions, 5 × 105 of A2780CP and SKOV3 cells were separately implanted in 6-plate wells and incubated (5% CO2, 37 °C) overnight. Next, the media was removed, the cells were treated with MEL-loaded PEGylated nanoliposomes and the control at the drug IC50 concentration and incubated (5% CO2, 37 °C) for 72 h. The supernatant was then removed, and the cells were washed twice with a BioLegend cell staining buffer. After that, 100, 10, and 5 μL of binding buffer, PI, and Annexin-V, respectively, were added and gently mixed. The mixture was incubated at room temperature for 15 min in dark, and cell analysis was performed by flow cytometry. Data were analyzed by the device software and the points recorded in the two-dimensional curve were divided into four regions Q1 to Q4, so that region Q1 represented necrotic cells with Annexin-V− and PI+ feature. Region Q2 referred to late apoptotic cells with Annexin-V+ and PI+ feature, region Q3 represented early apoptotic cells with Annexin-V+ and PI− feature, and region Q4 showed the healthy cells with the Annexin-V− and PI− feature.
2.8. Statistical analysis
The results were analyzed by the SPSS Software (V 22). The data were expressed as mean standard deviation (Mean ± SD, n = 3) from three independent experiments. Comparisons among groups were performed using a one-way analysis of variance (ANOVA) test. The significance level was set at 0.05.
3. Results
3.1. Characterization of Melphalan-loaded nanoliposomes.
The size, size distribution, and zeta potential of PEGylated liposomes and MEL-loaded PEGylated nanoliposomes were determined to be 113 ± 1.43 nm, 0.213 ± 0.04, −19.4 ± 1.2 mv; 129.1 ± 5.5 nm, 0.240 ± 0.13, and −18.9 0.31 mv, respectively (). The size and size distribution of nanoparticles are considered as important factors for determining the interaction of the particles with the cell membrane and their penetration through physiological barriers. The amount of surface charge (zeta potential) on the surface of vesicles indicates the function of the particles inside the body and the amount of repulsion between adjacent vesicles. The results also demonstrated that PEGylating reduced the size of nanoparticles. In addition, PEGylating has a great effect on strengthening the zeta potential of nanoliposome that is a prominent feature of nanopolymers [Citation18]. The morphology of nanoliposomes is shown by TEM () and indicated that the particles had a uniform, homogeneous surface, and spherical shapes.
3.2. Determination of loading and encapsulation efficiency of drug
Encapsulation and loading efficiencies of MEL in the nanoformulation were calculated according to the standard curve () with the help of EquationEquations (1)(1)
(1) and Equation(2)
(2)
(2) . According to the results, encapsulation and loading efficiencies were determined to be 98.3 ± 2.1% and 5.2 ± 1.3%, respectively. High encapsulation efficiency confirms the efficiency of nanoliposome in drug release.
3.3. Drug release from nanoparticles
In order to determine the effect of the PEGylated nanoliposomes on the release of MEL, the drug release from two formulations (nanoparticles containing drug and free drug) was measured at different times (1, 2, 4, 6, 8, 12, 24, and 48 h) using a dialysis bag method and the standard curve (). The results demonstrated that only 20.5% of MEL was released from PEGylated nanoliposomes after 48 h, while after this value for the free drug sample was 54.8% ().
3.4. Cell viability
The effects of MEL-loaded PEGylated nanoliposomes on A2780CP and SKOV3 cell viability were assessed and compared with the free drug using an MTT assay after 24, 48, and 72 h ( and ). The results demonstrated that by increasing concentrations, toxicity increased, indicating that drug concentrations played an important role in the in vitro toxicity of the MEL. Also, the nanoformulation had higher potency to kill A2780CP cells by 1.5-, 1.4-, and 1.4-fold compared to the standard drug after 24, 48, and 72 h, respectively (IC50 = 14.24, 10.94, 10.76; 9.23, 8.01, and 7.6 µg/mL, for the standard MEL and MEL-loaded PEGylated nanoliposomes, after 24, 48, and 72 h, respectively). In addition, MEL-loaded PEGylated nanoliposomes demonstrated higher toxicity against SKOV3 cells by 1.6-, 1.5-, and 1.5-fold compared to the free drug after 24, 48, and 72 h, respectively (IC50 = 15.21, 12.92, 11.88; 9.47, 8.74, and 8.06 µg/mL, for the standard MEL and MEL-loaded PEGylated nanoliposomes, after 24, 48, and 72 h, respectively) (). The results demonstrated that SKOV3 cells were more resistant to both formulations than the A2780CP.
Figure 5. Cytotoxicity effect of MEL-loaded PEGylated nanoliposomes and the free drug on A2780CP cells after 24, 48, and 72 h incubation (Error bars present means ± SD (n = 3)).
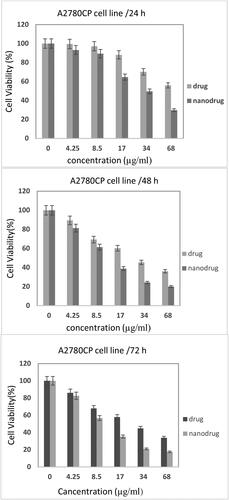
3.5. Apoptosis analysis
To evaluate the effect of the new formulation of MEL on the induction of cell death and cytotoxicity of MEL, apoptosis analysis was performed on A2780CP and SKOV3 cells using the flow cytometry method. As shown in , A2780CP and SKOV3 cells treated with MEL-loaded PEGylated liposomes underwent necrosis and apoptosis. The mean percentage of induced apoptosis in both cells treated with the nanoformulation compared to the control group is shown in and . The results demonstrated that using PEGylated nanoliposomes as a carrier for MEL led to an increase in the apoptotic cells compared to the control group.
Figure 8. Effects of MEL-loaded PEGylated nanoliposomes to induce apoptosis in A2780CP and SKOV3 cells using flow cytometry method. (a) Dot plot of A2780CP and SKOV3 cells treated by PBS for 72 h (control), (b) dot plot of A2780CP and SKOV3 cells treated by MEL-loaded PEGylated nanoliposomes.
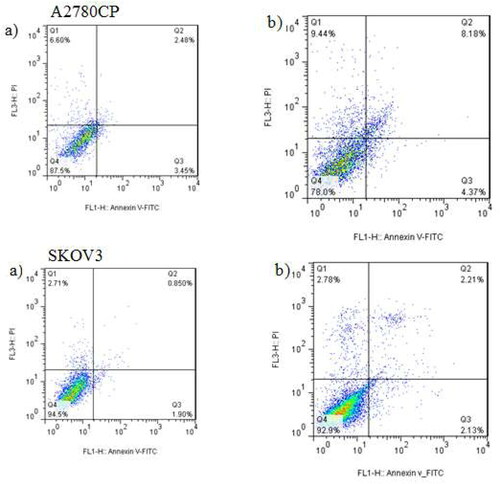
Table 1. Mean percentage of induced apoptosis in A2780CP cells treated with MEL-loaded PEGylated nanoliposomes compared to the control group after three replications.
Table 2. Mean percentage of induced apoptosis in SKOV3 cells treated with MEL-loaded PEGylated nanoliposomes compared to the control group after three replications.
4. Discussion
Ovarian cancer spreads from the cells in the ovaries to other parts of the body. Due to the late diagnosis of ovarian cancer and the lack of effective treatment methods, it has the highest mortality among women’s cancers [Citation19]. A common type of treatment for this type of cancer is chemotherapy. Some of the chemotherapy drugs used to treat ovarian cancer include malpractice. Since the efficacy of MEL chemotherapy agents based on alkylating agents is limited due to intracellular resistance, new treatment strategies are needed to improve the therapeutic effects of MEL [Citation20]. Therefore, finding a new technique to improve the effectiveness of treatment and increase the performance of chemotherapy drugs, such as MEL, with the reduction of side effects will be a serious issue. In this regard, nanodrug delivery systems have attracted increasing attention among researchers.
One of the most significant achievements of nanotechnology in drug delivery is the use of nanocarriers as a drug transfer system to the tumor site. The use of effective nanocarriers helps to increase the drug concentration to the desired level to eliminate cancerous tissues. Liposomes have been considered as an ideal carrier due to characteristics, such as high biocompatibility, ease of synthesis process, and safety [Citation21,Citation22]. Also, liposome surface modification using PEG helps to prevent the adsorption of nanoliposomes by the reticuloendothelial system (RES), increase drug life in the circulatory system, reduce side effects, and improve drug solubility and pharmacokinetic properties of the drug [Citation23,Citation24]. In the present study, nanoliposome with PEG coating, containing a high level of MEL (98.3 ± 2.1%), were synthesized using the reverse-phase evaporation method. According to the results, the preparation technique was sufficiently valid and reliable. Studies [Citation25,Citation26] have shown that particles smaller than 400 nm can exit the vascular endothelial system and accumulate at the tumor site, improving the therapeutic effect. In the current study, the DLS results showed that the average size of the synthesized nanoliposomes was 129.1 ± 5.5 nm, which was less than that of nanoliposomes (143.6 nm) obtained by Naseripour et al. [Citation27]. Because of the high permeability of PEG, it can penetrate the liposome layers and compresses them. Therefore, MEL-loaded PEGylated nanoliposomes showed a smaller diameter than that of nanoliposomes alone.
Zeta potential is one of the most important factors that have a major impact on how nanoliposomes function in drug delivery. It also affects the rate of drug release from nanoliposomes and stabilizes the enclosed drug [Citation28,Citation29]. In addition, PEGylating nanoliposomes have a significant effect on the improvement of the zeta potential of nanoliposomes. In the present study, the zeta potential of PEGylated nanoliposomes was reported −18.9 ± 0.31 mv, confirming the stability of the colloidal nanoliposome. The results of TEM showed the formation of uniform, homogeneous, and spherical nanoliposomes. The size of the nanoliposome obtained using TEM is smaller than that obtained by DLS because nanoliposomes showed a higher hydrodynamic volume in DLS due to the hydration effects. In fact, zetasizer shows the hydrodynamic diameter of the nanoliposome. This result has been also mentioned in a similar study by Lee et al. [Citation30].
The release pattern of MEL from PEGylated nanoliposomes was investigated using a dialysis bag method. Drug release pattern is an important factor in diagnosing the biological effects of nano-carriers [Citation31–33]. According to the results of the present study, it was found that the drug release rate from PEGylated nanoliposomes was slower compared to when the standard drug was used. In the early hours of drug release, an explosive release was observed that could be due to the drug release from the nanoliposomes surface. Reduction in the rate of release over time could be attributed to the overlapping and inhibitory effect of PEG on drug release from nanoliposome. Also, as the vesicles become more compact, the possibility of release from the nano-carrier wall becomes slower. This phenomenon can increase the efficiency of the drug encapsulation in nanoparticles.
Also, cell viability was assessed using MTT assay to determine the cytotoxicity effect of MEL in standard form and loaded into PEGylated liposomes. In this experiment, PEGylated nanoliposomes (without drug) did not show toxicity on A2780CP and SKOV3 cells. Also, it was demonstrated that MEL-loaded PEGylated liposomes had a lower IC50 compared to the standard MEL at all concentrations and various incubation times, indicating that the toxicity of the MEL increased when it was loaded into PEGylated nanoliposomes. Previous studies [Citation34,Citation35] have reported similar results, which reflect the effect of PEGylated nanoliposomes on cancer cell death.
The process of apoptosis or programmed cell death is a conserved method, controlled by genes used to kill unwanted and unnecessary cells in living organisms [Citation36,Citation37]. The results of flow cytometry demonstrated that the IC50 concentration MEL-loaded PEGylated nanoliposomes increased the induction of apoptosis in A2780CP and SKOV3 cells in the control group after 72 h, confirming the results of cell viability. Also, the induction of apoptosis was higher than cell necrosis, reflecting that the synthesized formulation could cause apoptosis and inhibit the growth of cancer cells [Citation38]. According to the results reported in , it could be observed that the percentage of apoptotic cells in A2780CP cells treated with MEL-loaded PEGylated nanoliposomes increased compared to the control group. According to the p-values presented in , apoptotic and necrotic cells in the treatment group showed a significant level compared to the control group (p < 0.05). As shown in , 2.13% of the treated SKOV3 cells experienced late apoptosis, increased compared to the control group and exhibited a significant difference (p < 0.048) compared to the control group, while the increase in the primary apoptosis with a significance level of 0.063 and the increase in necrosis with a significance level of 0.196 did not show a significant difference between the two groups. Hence, the results of cell apoptosis confirmed the results of cytotoxicity, which indicated the resistance of SKOV3 cancer cells to MEL-loaded PEGylated nanoliposomes. Due to the normality of data distribution, the comparison between the treatment group and the control group was analyzed using a one-way ANOVA method at a significance level. The information is displayed as a standard deviation of the mean. Similar results have been reported by previous studies [Citation39,Citation40], indicating the importance of PEGylated nanoliposomes in nanodrug delivery systems and their potential impact on cellular toxicity.
5. Conclusion
In this study, MEL-loaded PEGyulated nanoliposomes were prepared by the reverse-phase evaporation method. Physical and chemical properties of nanoliposomes, such as particle size, size distribution, and zeta potential, were investigated. It was found that the synthesized nano-carriers containing Melphalan MEL had a greater cellular toxicity effect on the ovarian cancer cells compared to the standard MEL. Considering the valuable properties of MEL-loaded PEGylated nanoliposomes produced in this study, such as high biocompatibility, suitable size, and slow curve of drug release, it is necessary to perform more studies on this formulation in vivo. It is hoped that the prepared formulation will be an effective way to increase the stability of MEL in the body to treat ovarian cancer in the near future.
Acknowledgments
This work was a part of the Ph.D. thesis and carried out in Sheikh Bahaei's Department Research Laboratory in Science and Research Branch, Islamic Azad University, Tehran, Iran.
Disclosure statement
The authors confirm that this article content has no conflict of interest.
References
- Doun SKB, Alavi SE, Esfahani MKM, et al. Efficacy of Cisplatin-loaded poly butyl cyanoacrylate nanoparticles on the ovarian cancer: an in vitro study. Tumour Biol. 2014;35(8):7491–7497.
- Turner TB, Meza-Perez S, Londoño A, et al. Epigenetic modifiers upregulate MHC II and impede ovarian cancer tumor growth. Oncotarget. 2017;8(27):44159–44170.
- Bray F, Ferlay J, Soerjomataram I, et al. Global cancer statistics 2018: GLOBOCAN estimates of incidence and mortality worldwide for 36 cancers in 185 countries. CA Cancer J Clin. 2018;68(6):394–424.
- Klymenko Y, Johnson J, Bos B, et al. Heterogeneous cadherin expression and multicellular aggregate dynamics in ovarian cancer dissemination. Neoplasia. 2017;19(7):549–563.
- Koohi Moftakhari Esfahani M, Alavi SE, Shahbazian S, et al. Drug delivery of cisplatin to breast cancer by polybutylcyanoacrylate nanoparticles. Adv Polym Technol. 2018;37(3):674–678.
- Yingchoncharoen P, Kalinowski DS, Richardson DR. Lipid-based drug delivery systems in cancer therapy: what is available and what is yet to come. Pharmacol Rev. 2016;68(3):701–787.
- Alavi SE, Muflih Al Harthi S, Ebrahimi Shahmabadi H, et al. Cisplatin-loaded polybutylcyanoacrylate nanoparticles with improved properties as an anticancer agent. Int J Mol Sci. 2019;20(7):1531.
- Child JA, Morgan GJ, Davies FE, et al. High-dose chemotherapy with hematopoietic stem-cell rescue for multiple myeloma. N Engl J Med. 2003;348(19):1875–1883.
- Ghaferi M, Koohi Moftakhari Esfahani M, Raza A, et al. Mesoporous silica nanoparticles: synthesis methods and their therapeutic use-recent advances. J Drug Target. 2020;29(2):131–154.
- Allen TM, Cullis PR. Drug delivery systems: entering the mainstream. Science. 2004;303(5665):1818–1822.
- Caruso G, Caffo M, Alafaci C, et al. Could nanoparticle systems have a role in the treatment of cerebral gliomas? Nanomedicine. 2011;7(6):744–752.
- Ghaferi M, Asadollahzadeh MJ, Akbarzadeh A, et al. Enhanced efficacy of PEGylated liposomal cisplatin: In vitro and in vivo evaluation. Int J Mol Sci. 2020;21(2):559.
- Al Harthi S, Alavi SE, Radwan MA, et al. Nasal delivery of donepezil HCl-loaded hydrogels for the treatment of Alzheimer’s disease. Sci Rep. 2019;9(1):1–20.
- Madni A, Sarfraz M, Rehman M, et al. Liposomal drug delivery: a versatile platform for challenging clinical applications. J Pharm Pharm Sci. 2014;17(3):401–426.
- Alavi SE, Cabot PJ, Moyle PM. Glucagon-like peptide-1 receptor agonists and strategies to improve their efficiency. Mol Pharm. 2019;16(6):2278–2295.
- Ghafari M, Haghiralsadat F, Khanamani Falahati‐Pour S, et al. Development of a novel liposomal nanoparticle formulation of cisplatin to breast cancer therapy. J Cell Biochem. 2020;121(7):3584–3592.
- Ghaferi M, Amari S, Mohrir BV, et al. Preparation, characterization, and evaluation of cisplatin-loaded polybutylcyanoacrylate nanoparticles with improved in vitro and in vivo anticancer activities. Pharmaceuticals. 2020;13(3):44.
- Na K, Lee SA, Jung SH, et al. Elastin-like polypeptide modified liposomes for enhancing cellular uptake into tumor cells. Colloids Surf B Biointerfaces. 2012;91:130–136.
- Gayam SR, Venkatesan P, Sung Y-M, et al. An NAD(P)H:quinone oxidoreductase 1 (NQO1) enzyme responsive nanocarrier based on mesoporous silica nanoparticles for tumor targeted drug delivery in vitro and in vivo. Nanoscale. 2016;8(24):12307–12317.
- Catanzaro D, Nicolosi S, Cocetta V, et al. Cisplatin liposome and 6-amino nicotinamide combination to overcome drug resistance in ovarian cancer cells. Oncotarget. 2018;9(24):16847–16860.
- Andrieux K, Couvreur P. Polyalkylcyanoacrylate nanoparticles for delivery of drugs across the blood–brain barrier. Wiley Interdiscip Rev Nanomed Nanobiotechnol. 2009;1(5):463–474.
- Bangham AD, Horne R. Negative staining of phospholipids and their structural modification by surface-active agents as observed in the electron microscope. J Mol Biol. 1964;8(5):660–IN10.
- Alavi SE, Cabot PJ, Yap GY, et al. Optimized methods for the production and bioconjugation of site-specific, alkyne-modified glucagon-like peptide-1 (GLP-1) analogs to azide-modified delivery platforms using copper-catalyzed alkyne-azide cycloaddition. Bioconjug Chem. 2020;31(7):1820–1834.
- Lee BK, Yun YH, Park K. Smart nanoparticles for drug delivery: boundaries and opportunities. Chem Eng Sci. 2015;125:158–164.
- Martins S, Tho I, Ferreira D, et al. Physicochemical properties of lipid nanoparticles: effect of lipid and surfactant composition. Drug Dev Ind Pharm. 2011;37(7):815–824.
- Nandhakumar S, Dhanaraju MD, Sundar VD, et al. Influence of surface charge on the in vitro protein adsorption and cell cytotoxicity of paclitaxel loaded poly (ε-caprolactone) nanoparticles. Bull Fac Pharm Cairo Univ. 2017;55(2):249–258.
- Naseripour M, Abrishami M, Sedaghat A, et al. Preparation and in vivo evaluation of nanoliposomes containing melphalan after intravitreal injection in albino rabbits. J Pharm Invest. 2016;46(6):575–582.
- Echeverri-Cuartas CE, Gartner C, Lapitsky Y. PEGylation and folate conjugation effects on the stability of chitosan-tripolyphosphate nanoparticles. Int J Biol Macromol. 2020;158:1055–1065.
- Kouchakzadeh H, Shojaosadati SA, Maghsoudi A, et al. Optimization of PEGylation conditions for BSA nanoparticles using response surface methodology. AAPS PharmSciTech. 2010;11(3):1206–1211.
- Li D, Lu B, Huang Z, et al. A novel melphalan polymeric prodrug: preparation and property study. Carbohydr Polym. 2014;111:928–935.
- Mishra PR, Al Shaal L, Müller RH, et al. Production and characterization of hesperetin nanosuspensions for dermal delivery. Int J Pharm. 2009;371(1-2):182–189.
- Otsuka H, Nagasaki Y, Kataoka K. PEGylated nanoparticles for biological and pharmaceutical applications. Adv Drug Deliv Rev. 2003;55(3):403–419.
- Soltani M, Chen P. Effect of tumor shape and size on drug delivery to solid tumors. J Biol Eng. 2012;6(1):4.
- Izadi M, Ebrahimi Shahemabadi H, Kanaani L. Investigation of characteristics of loaded carboplatin on the liposomal nanoparticles on the cell carcinoma of the human brain c6. Adv Biores. 2016;7(4):113–118.
- Mehrabi M, Esmaeilpour P, Akbarzadeh A, et al. Efficacy of pegylated liposomal etoposide nanoparticles on breast cancer cell lines. Turk J Med Sci. 2016;46(2):567–571.
- Cotter T, Lennon S, Glynn J, et al. Cell death via apoptosis and its relationship to growth, development and differentiation of both tumour and normal cells. Anticancer Res. 1990;10(5A):1153–1159.
- Darzynkiewicz Z, Bruno S, Del Bino G, et al. Features of apoptotic cells measured by flow cytometry. Cytometry. 1992;13(8):795–808.
- Chiani M, Norouzian D, Shokrgozar MA, et al. Folic acid conjugated nanoliposomes as promising carriers for targeted delivery of bleomycin. Artif Cells Nanomed Biotechnol. 2018;46(4):757–763.
- Dadgar N, Esfahani MKM, Torabi S, et al. Effects of nanoliposomal and pegylated nanoliposomal artemisinin in treatment of breast cancer. Indian J Clin Biochem. 2014;29(4):501–504.
- Hwang T-L, Lee W-R, Hua S-C, et al. Cisplatin encapsulated in phosphatidylethanolamine liposomes enhances the in vitro cytotoxicity and in vivo intratumor drug accumulation against melanomas. J Dermatol Sci. 2007;46(1):11–20.