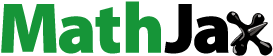
Abstract
In the present study, iron nanoparticles were green-synthesized using the aqueous extract of Alhagi maurorum leaf aqueous extract. The synthesized FeNPs were characterized by analytical techniques including EDX, FE-SEM, XRD, UV–vis and FT-IR. The anti-human lung cancer activity of FeNPs was evaluated using MTT assay. The nanoparticles were formed in a spherical shape in the average size of 60.11 nm. In the cellular and molecular part of the recent study, the treated cells with FeNPs were assessed by MTT assay for 48 h about the cytotoxicity and anti-human lung cancer properties on normal (HUVEC) and lung cancer cell lines i.e. HLC-1 and PC-14. The viability of malignant lung cell line reduced dose-dependently in the presence of FeNPs. The IC50 of FeNPs was 195 and 181 µg/mL against HLC-1 and PC-14 cell lines, respectively. In the antioxidant test, the IC50 of FeNPs and BHT against DPPH free radicals was 214 and 62 µg/mL, respectively. After the clinical study, iron nanoparticles containing A. maurorum leaf aqueous extract may be used to formulate a new chemotherapeutic drug or supplement to treat several types of human lung cancer.
1. Introduction
Alhagi maurorum, with common name of camelthorn, belongs to Fabaceae family. The plant is a perennial herb. A. maurorum grows in different parts of Asia, Europe and North Africa [Citation1,Citation2] The plant is known as an important medicinal plant in folk medicine around the world. The whole plant of A. maurorum is used as is laxative, diuretic and expectorant [Citation3]. The plant is effective to reduce gastric acidity [Citation4]. The plant showed diaphoretic, antiseptic, healing of wounds properties [Citation5]. The essential oil of the leaves of A. maurorum is used to treat rheumatism [Citation6]. A. maurorum is rich in phenolic compounds, glycosides, sterols, steroids, resins, alkaloids, flavonoids, fatty acids, coumarins and vitamins. The presence of these different classes of secondary metabolites in A. maurorum is the chiefly responsible for its bioactivity [Citation4, Citation7–9].
In recent centuries, the application of nanotechnology has played an important role in the development of science. Nanoscience has shown that if we reduce the size to manometers, unique properties such as optical properties, electrical conductivity, hardness and chemical reaction will be obtained [Citation10–12]. Nanoparticles are widely used because of their high surface-to-volume ratio, small size, and excellent reactivity. One of the most important advances in nanotechnology is the production and application of nanoparticles in the biological sciences [Citation10–14]. Nanoparticles are generally effective in a wide variety of sectors that if their production is based on green chemistry, they have great applications in the fields of food, medicine, cosmetics and health. Nanoparticles centred on inorganic materials such as magnetic metals, their oxides and alloys, and semiconductors have the most studies and potential in biomedicine from diagnosis to treatment of diseases [Citation11–13]. The effects of nanoparticles should be predictable, controllable and get the desired results with minimal toxicity. Metallic nanoparticles used in treatment and diagnosis, in addition to being non-toxic, must be biocompatible and stable in vivo. Also, by making appropriate changes in the surface of metallic nanoparticles, they will have a wide range of applications by binding to biomolecules and various carriers to cross the cell membrane and target the desired part in the body. One of the important points in the production of nanoparticles is the use of cost-effective and efficient precursors [Citation12, Citation13, Citation15, Citation16]. There are three biological, chemical and physical methods to synthesize the nanoparticles. Chemical and physical methods are time-consuming and costly. In addition, these methods use some toxic additive chemicals that cause adverse effects on medical applications by adsorption on the surface. Applying the principles of green chemistry has decreased the use of toxic compounds or hazardous solvents, provided optimal regeneration conditions and ameliorated materials for the chemical, and raised new sources for green synthesis [Citation12,Citation13, Citation15, Citation17,]. Therefore, one of the primary goals of green nanotechnology is to produce nanomaterials without harm to human health or environment, and to develop and design nanomaterials and products that are suitable solutions to environmental problems. The synthesis of nanoparticles by similar biological methods results in greater catalytic activity and limits the use of toxic and expensive chemicals [Citation10–13]. In biological methods, plant extracts, enzymes or proteins carrying natural resources are used to produce or stabilize nanoparticles. The nature of the materials used to make nanoparticles influences the shape, structure and morphology of these nanoparticles [Citation10–14]. Biological systems involved in the green synthesis of nanoparticles, plants and their derivatives, as well as microorganisms such as algae, fungi and bacteria. Plant parts such as roots, leaves, stems, fruits and tiny parts such as the kernel and skin of the fruit are suitable to synthesize the nanoparticles because their extracts are rich in phytochemicals that act as stabilizing and reducing substances [Citation10–13]. The use of natural plant extracts is a cheap and environmentally friendly process and does not require intermediate groups. Short time, no need for expensive equipment, precursors, high purity product and excellent quality without impurities are the features of this method. This is possible very quickly, at room temperature and pressure as well as easily on a large scale. Bio-reduction in the conversion of base metal ions is carried out by various plant metabolites such as alkaloids, phenolic compounds, terpenoids and coenzymes [Citation15, Citation16].
Recently, scientists have used the anticancer effects of medicinal plants in several traditional medicines for synthesizing the gold nanoparticles containing natural compounds. So far, the anticancer effects of Tinospora cordifolia, Sophora subprostrata, Euphoria hirta, Barleria prionitis, Lubinus perennis, Maytenus boaria, Cephaelis acuminate, Phyllanthus niruri, Solanum seaforthianum, Boswellia serrate, Lavendula officinalis and Cephalotaxus harringtonia drupacea have been proved [Citation16]. In the current research, the properties of iron nanoparticles formulated by A. maurorum leaf aqueous extract against common lung cancer cell lines i.e. HLC-1 and PC-14 were evaluated.
2. Material and methods
2.1. Preparation and extraction of aqueous extract
First, the dried leaves of A. maurorum were grounded. Then, 80 g of the sample was macerated in 500 mL of boiling water for 3 h. Next, the filtration and evaporation were applied to obtain the concerted extract. Finally, the extract was put in a freeze drier for 72 h to produce the powder extract of A. maurorum.
2.2. Green synthesis and chemical characterization of FeNPs
A reported procedure (with some modifications) was used to green-synthesis of FeNPs [Citation18]. First, 10 mL of the plant extract (0.2 g in 100 mL of water) was added to 30 mL of 0.02 M FeCl3·6H2O. Then, the mixture was stirred for 90 min at 50 °C. After the time, the iron nanoparticle was formed. The colour-changing from yellow to black indicated the formation of iron nanoparticles. The obtained FeNPs was washed three times with water and centrifuged at 10,000 rpm for 15 min. Finally, the precipitate was dried at room temperature. The synthesized nanoparticles as a black powder were kept in a vial for chemical characterization and biological activity evaluation.
2.3. Antioxidant activities of FeNPs
Free radicals are unstable atoms that have one or more unpaired electrons. These active species are very harmful due to their high reactivity. They are most often formed when oxygen molecules in the body split into separate unstable atoms. This process can turn into a chain reaction. Free radicals excessive production in the body causes cell damage and oxidative stress. Genetics and the environment affect the extent of free radical damage in individuals. These active molecules are produced as part of the body's natural biological processes. One of the most important free radicals is DPPH. DPPH is widely used to study the antioxidant activities of natural compounds and nanoparticles [Citation16].
In this method, the antioxidant activity of nanoparticles is measured for DPPH radical scavenging. The basis of the action is the reduction of the alcoholic solution of DPPH in the presence of hydrogen-giving antioxidants, especially phenolic compounds. To achieve the IC50 of the samples, 11 different concentrations of nanoparticles were prepared and the percentage of inhibitory versus concentration was used to plot. In practice, 300 μL of 1 M DPPH was combined with 100 μL of diluted sample to a final volume of 2 mL using methanol. After half an hour in the dark, the absorbance was read at 517 nm and the inhibitory percentage was obtained using the following formula:
In this formula, ‘Control A’ shows the negative control of light absorption that lacks nanoparticles, and ‘Sample A’ expresses the amount of light absorption of different concentrations of nanoparticles [Citation16].
2.4. Anti-human lung cancer potentials of FeNPs
Investigation of cell proliferation and survival is one of the most important and basic techniques in cell laboratories. This study requires accurate quantification of the number of living cells in the cell culture medium. Therefore, cell survival calculation methods are necessary to optimize cell culture conditions, evaluate cell growth factors, detect antibiotics and anticancer drugs, evaluate the toxic effects of environmental pollutants, and study apoptosis. Many methods can be used for such purposes, but indirect methods using fluorescent or dye (chromogenic) markers provide very fast large-scale methods. Among these methods, measurement of cell survival by MTT method (3-(4,5-dimethylthiazol-2-yl)-2,5-diphenyltetrazolium bromide) is the most widely used method [Citation19]. In this research, we used the following cell lines to evaluating anti-human lung cancer and cytotoxicity effects of FeCl3, A. maurorum leaf aqueous extract, and FeNPs using an MTT method.
Human lung cancer cell lines: Lung well-differentiated bronchogenic adenocarcinoma (HLC-1), and lung poorly differentiated adenocarcinoma (PC-14).
Normal cell line: HUVEC.
Because nanoparticles is not soluble directly in 1640-RPMI medium and also the solvent of dimethyl sulfoxide (DMSO) nanoparticles itself has cytotoxic effects, to eliminate the effect of this substance on treated cells, its amount in the final solution is considered less than 1%. DMSO is not toxic to concentrations less than 1% and the concentration of this solvent is important in this regard. For this purpose, 1000 µg of nanoparticle was dissolved in 100 μL of DMSO solvent after weighing. Then 1 mL of culture medium was added for better dissolution and finally the volume of solution was increased to 24 mL using culture medium: Then, successive dilutions of this stock were used in the proportions of 1–1000 μg/mL. Eleven concentrations were used for the cell lines [Citation19].
In this study, 100 μL of culture medium containing 104 cells per plate 96 were placed. After 24 h, incubation of concentrations of 1–1000 micrograms per millilitre of nanoparticles was added to the cells, and incubated for 24, 48 and 72 h, respectively. After these times, 20 μL of MTT plate with a concentration of 5 mg/mL was added to each cell and incubated in the dark for another 4 h. After some time, the MTT medium was carefully removed, and 200 μL of acidified isopropanol were added to each plate to remove the purple formanes. After 15 min of incubation at room temperature, the light absorption of each well was read using an ELISA at 570 nm against a reference wavelength of 690 nm. The findings were reported as cell survival and IC50 (concentration that inhibits cell growth up to 50%) based on the concentration curve (μg/mL) [Citation19].
It should be noted that the effect of each concentration of the nanoparticles on cell lines was investigated in five independent experiments. According to the values of light absorption obtained by the ELISA reader, the percentage of growth inhibition related to each concentration was calculated using the following formula:
Finally, linear regression was done to gain IC50, which indicates the nanoparticles concentration, which causes 50% cancer cell growth inhibition. Using the curve, the line equation for cancer cells was obtained, respectively, then by replacing 50% inhibition in the equation, the IC50 value for cancer cells was obtained [Citation19].
2.6. Statistical analysis
SPSS statistical software version 22 was used for data analysis and the findings were determined as the mean standard deviation of five replications. Data were analysed using one-way analysis of variance and Duncan post hoc test and the significance level in the test was considered 0.05.
3. Results and discussion
3.1. Chemical characterization of FeNPs
3.1.1. UV–vis analysis
The UV–vis spectrum of the green-synthetic nanoparticles of FeNPs is presented in . The surface plasmon resonance (SPR) of FeNPs was completed using UV–vis spectroscopy. The produce of the green-synthetic FeNPs was observed. The advanced SPR bands at the wavelengths of 220 and 300 nm approved the formation of the iron nanoparticles. The bands are very close to a previously reported on the green synthesized of iron nanoparticles [Citation20, Citation21].
3.1.2. FT-IR analysis
The FT-IR spectra of iron nanoparticles and plant extract are exhibited in . The formation of FeNPs is approved by the presence of the peaks at wavenumbers of 449, 532 and 613 cm−1. These peaks attribute to bending vibration of Fe–O. Similar peaks with some differences in the wavenumber have been reported for green-synthetic FeNPs by other research groups [Citation22–25]. The other peaks in the spectrum are attributed to the functional groups of different organic compounds in A. maurorum extract, which are linked to the surface of FeNPs. The presence of secondary metabolites such as phenolic, flavonoid, triterpenes in A. maurorum extract has been reported previously [Citation7–9]. The peaks in 3413 and 2927 cm−1 are related to O–H and aliphatic C–H stretching; the peaks from 1407 to 1675 cm−1 are corresponded to C = C and C = O stretching, and the peaks at 1197 and 1103 cm−1 could be ascribed to –C–O and C–O–C stretching.
3.1.3. XRD analysis
The XRD diffraction patterns of FeNPs evaluated its crystallinity. The pattern of the diffractogram is shown in . The formation of nanoparticles was approved to this result. Despite the small size of FeNPs, the pattern of XRD indicated well crystallizing. The achieved data were compared with the standard database of ICDD PDF card no. 96-900-5813. The signals with 2θ values of 35.23, 37.31, 54.03, 61.38 and 75.37 are indexed as (311), (222), (422), (404) and (533) planes. The peaks at different degrees are also reported previously [Citation26–29]. A 39.12 nm was measured for the crystal size of FeNPs that was calculated using X-ray diffraction and according to Scherer’s equation.
3.1.4. SEM analysis
The morphology of FeNPs was assessed by the FE-SEM technique. presents the FE-SEM of FeNPs. The images show the spherical shape for the nanoparticles with average particle size of 60.11 nm. Furthermore, the nanoparticles are aggregated. In our literature review, 11.0–98.79 nm was reported for biosynthesized of iron oxide using plant extracts as the capping agent [Citation30–34].
3.1.5. EDX analysis
The qualitative analysis of EDX was run to screen the elemental analysis of FeNPs. The EDX diagram of FeNPs is shown in . The findings approved the appearance of iron (by the peaks at 0.71 keV for FeLα, peak at 6.44 for FeKα and peak at 7.13 keV for FeLβ), oxygen (by the peak around 0.52 keV for OLα) and carbon (by the peak around 0.28 keV for CLα) in FeNPs. The signal for iron has been reported by other research groups [Citation27]. The presence of oxygen and carbon approved the linkage between iron nanoparticles and organic compounds of the plant extract.
3.2. Cytotoxicity, anti-human lung cancer and antioxidant activities of FeNPs
Cancer is a genetic disease that includes 277 types of diseases. There are also more than 100,000 types of chemicals in our environment, of which only 35,000 have been analysed and about 300 of them produce cancer. The remaining 65,000 chemicals in nature have not yet been tested. Cancer occurs due to uncontrolled cell division, which is the result of environmental factors and genetic disorders [Citation35, Citation36]. The four key genes involved in cancer cell conduction include DNA repair genes, tumour suppressor genes, oncogenes and programmed death genes [Citation37, Citation38]. If a genetic mutation is produced in a cell, normal cells go out of their way and are affected by new commands that progress to cancer cells. In addition to chemicals, sunlight, shortwave, viruses and bacteria also have a special role in causing cancer [Citation36–38]. Cancers have existed since the beginning of mankind. In recent decades, advances in computer molecular medicine have been able to not only study the causes and mechanisms of this deadly disease but also to perform better in its early diagnosis and treatment [Citation21, Citation39]. More than 50% of cancers are currently being treated, especially if diagnosed early. Cancer can be treated in several ways: surgery, chemotherapy, radiation therapy, immunotherapy, gene therapy or a combination of these. Due to the relative inefficiency and very severe side effects of chemotherapy drugs, researchers and scientists have sought a new formulation of various compounds, especially metallic nanoparticles [Citation21, Citation38].
In this investigation, the treated cells with different concentrations of the present FeCl3, A. maurorum leaf aqueous extract and FeNPs were assessed by MTT assay for 48 h about the cytotoxicity properties on normal (HUVEC) and lung malignancy cell lines i.e. HLC-1 and PC-14.
The absorbance rate was evaluated at 570 nm, which represented viability on normal cell line (HUVEC) even up to 1000 μg/mL for FeCl3, A. maurorum leaf aqueous extract and FeNPs ( and ).
Figure 6. The anti-human lung cancer properties (cell viability (%)) of FeNPs (concentrations of 0–1000 µg/mL) against human lung cancer (NCI-N87 (I) and MKN45 (II)) and normal (HUVEC: III) cell lines.
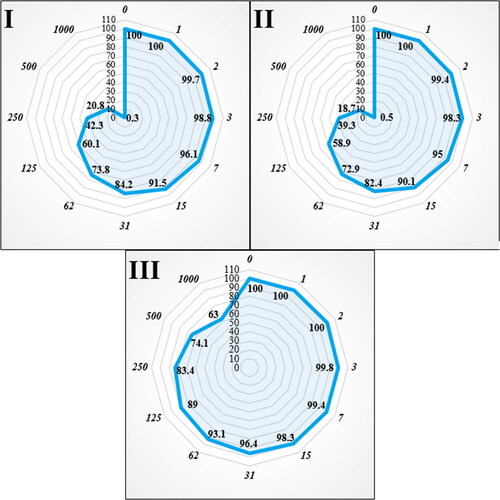
Table 1. The IC50 of FeNPs in the anti-human lung cancer test.
The viability of malignant lung cell line reduced dose-dependently in the presence of FeCl3, A. maurorum leaf aqueous extract and FeNPs. The IC50 of FeNPs was 195 and 181 µg/mL against HLC-1 and PC-14 cell lines, respectively ( and ).
The numbers indicate the percent of cell viability at the concentrations of 0–1000 μg/mL of FeNPs against several human lung cancer cell lines.
It seems that the anti-human lung cancer effect of recent nanoparticles is due to their antioxidant effects. Because tumour progression is so closely linked to inflammation and oxidative stress, a compound with anti-inflammatory or antioxidant properties can be an anticarcinogenic agent [Citation22, Citation24]. Many nanoparticles have pharmacological and biochemical properties, including antioxidant and anti-inflammatory properties, which appear to be involved in anticarcinogenic and antimutagenic activities [Citation22–24].
In this study, we assessed the antioxidant properties of A. maurorum leaf aqueous extract green-synthesized FeNPs by using the DPPH test as a common free radical. Oxidation is the electrons transfer from an atom and is the aerobic life and metabolism part of living organisms. Oxygen is the receptor for electrons in the electron transport system, which yields energy from adenosine triphosphate (ATP) in the body. Under certain conditions, oxygen may become a single electron and release free radicals. When oxygen becomes a single electron, it is called reactive oxygen species (ROS). Oxidative loss to proteins, DNA and other macromolecules is one of the internal causes of degenerative diseases such as aging, cardiovascular disease, cancer, immune system deficiency, cataracts and abnormal brain function. Single oxygen, high-energy, mutagenic oxygen can be produced by lipid peroxidation by the transmission of energy from light or the respiratory tract of neutrophils [Citation10–12]. Some free radicals have positive roles such as regulating cell growth, phagocytosis, energy production, intracellular signals, or the important biological compounds synthesis. Antioxidants produced in the body fight free radicals with two systems: enzymatic defense and non-enzymatic defense. Superoxide dismutase, catalase, and glutathione peroxidase metabolize lipid peroxide, hydrogen peroxide, and superoxide and prevent the production of toxic hydroxyl radicals [Citation12, Citation16]. In non-enzymatic defense, there are two classes of fat-soluble antioxidants (such as carotenoids and vitamin E) and water-soluble (glutathione and vitamin C) that trap free radicals. These two systems help neutralize oxidants. However, oxidants can escape from antioxidants and damage tissues. In this case, the activated antioxidant repair system (which is the enzymes lipase, protease, transferase and DNA repair enzymes), counteract the oxidant effects. However, due to deficiencies in the production of antioxidants in the body or due to physiopathological factors and situations (such as smoking, air pollution, UV radiation, diets containing high unsaturated fatty acids, inflammation, ischemia, bleeding, etc.) that ROS are yielded in large quantities at the wrong place and time, oral antioxidants are needed to counteract the oxidative damage cumulative effects [Citation11, Citation12, Citation16].
The scavenging capacity of A. maurorum leaf aqueous extract green-synthesized FeNPs and BHT at different concentrations expressed as percentage inhibition has been indicated in and .
Table 2. The IC50 of FeNPs and BHT in the antioxidant test.
In the antioxidant test, the IC50 of FeNPs and BHT against DPPH free radicals were 214 and 62 µg/mL, respectively ( and ).
The numbers indicate the percent of free radical (DPPH) inhibition at the concentrations of 0–1000 μg/mL of FeNPs(I) and BHT(II).
4. Conclusion
In summary, the green synthesis of iron nanoparticles was carried out using an aqueous extract of A. maurorum. The nanoparticles were characterized using common chemical techniques such as UV-vis, FT-IR, XRD, FE-SEM and EDS. The crystal size of FeNPs was 39.12 that calculated using XRD analysis. The FE-SEM images indicated a spherical morphology for FeNPs with average size of 60.11 nm, which is well known as a sufficient size for the synthetic nanoparticles. The FeNPs showed the best antioxidant activities against DPPH. The IC50 of FeNPs and BHT against DPPH free radicals was 214 and 62 µg/mL, respectively. The viability of malignant lung cell line reduced dose-dependently in the presence of FeNPs. The IC50 of FeNPs was 195 and 181 µg/mL against HLC-1 and PC-14 cell lines, respectively. After clinical study, FeNPs containing A. maurorum leaf aqueous extract can be utilized as an efficient drug in the treatment of lung cancer in humans.
Authors’ contributions
All authors contributed and discussed in the analysis and results and commented on the manuscript. All authors read and approved the final manuscript.
Ethical approval
The study did not involve human participants and/or animals.
Disclosure statement
No potential conflict of interest was reported by the author(s).
References
- Hamed A, Perrone A, Mahalel U, et al. Oleanane glycosides from the roots of Alhagi maurorum. Phytochem Lett. 2012;5(4):782–787.
- Al-Saleem MSM, Al-Wahaib LH, Abdel-Mageed WM, et al. Antioxidant flavonoids from Alhagi maurorum with hepatoprotective effect. Pharmacogn Mag. 2019;15(65):592.
- Akbar S. Handbook of 200 medicinal plants: a comprehensive review of their traditional medical uses and scientific justifications; 2020.
- Olas B, Hamed AI, Oleszek W, et al. Comparison of biological activity of phenolic fraction from roots of Alhagi maurorum with properties of commercial phenolic extracts and resveratrol. Platelets. 2015;26(8):788–794.
- Ahmad N, Shinwari ZK, Hussain J, et al. Phytochemicals, antibacterial and antioxidative investigations of Alhagi maurorum medik. Pak J Bot. 2015;47:121–124.
- Laghari AH, Ali Memon A, Memon S, et al. Determination of free phenolic acids and antioxidant capacity of methanolic extracts obtained from leaves and flowers of camel thorn (Alhagi maurorum). Nat Prod Res. 2012;26(2):173–176.
- Awaad Amani A, Maitland D, Soliman G. Antiulcerogenic activity of Alhagi maurorum. Pharm Biol. 2006;44(4):292–296.
- Laghari AH, Memon S, Nelofar A, et al. Alhagi maurorum: a convenient source of lupeol. Ind Crops Prod. 2011;34(1):1141–1145.
- Al-Snai A, Al-Kamel ML, Esmael ME. Antifungal effect of Alhagi maurorum phenolic extract. IOSR J Pharm. 2019;9:7–14.
- (a) Ghashghaii A, Hashemnia M, Nikousefat Z, et al. Wound healing potential of methanolic extract of Scrophularia striata in rats. Pharm Sci. 2017;23(4):256–263. (b) Goorani S, Shariatifar N, Seydi N, et al. The aqueous extract of Allium saralicum RM Fritsch effectively treat induced anemia: experimental study on Wistar rats. Orient Pharm Exp Med. 2019;19:403–413.
- (a) Abdoli M, Sadrjavadi K, Arkan E, et al. Polyvinyl alcohol/Gum tragacanth/graphene oxide composite nanofiber for antibiotic delivery. J Drug Deliv Sci Technol. 2020;60:102044. (b) Dou L, Zhang X, Zangeneh MM, et al. Efficient biogenesis of Cu2O nanoparticles using extract of Camellia sinensis leaf: evaluation of catalytic, cytotoxicity, antioxidant, and anti-human ovarian cancer properties. Bioorg Chem. 2021;106:104468. (c) Han Y, Gao Y, Cao X, et al. Ag NPs on chitosan-alginate coated magnetite for synthesis of indazolo [2,1-b] phthalazines and human lung protective effects against α-Guttiferin. Int J Biol Macromol. 2020;164:2974–2986.
- (a) Sujayev A, Taslimi P, Garibov E, et al. Novel cyclic thiourea derivatives of aminoalcohols at the presence of AlCl3 catalyst as potent α-glycosidase and α-amylase inhibitors: synthesis, characterization, bioactivity investigation and molecular docking studies. Bioorg Chem. 2020;104:104216. (b) Sun T, Gao J, Shi H, et al. Decorated Au NPs on agar modified Fe3O4 NPs: investigation of its catalytic performance in the degradation of methylene orange, and anti-human breast carcinoma properties. Int J Biol Macromol. 2020;165:787–795.
- Kooti W, Servatyari K, Behzadifar M, et al. Effective medicinal plant in cancer treatment. Part 2: review study. J Evid Based Complement Altern Med. 2017;22(4):982–995.
- Sharma N, Jandaik S, Kumar S, et al. Synthesis, characterisation and antimicrobial activity of manganese- and iron-doped zinc oxide nanoparticles. J Exp Nanosci. 2016;11(1):54–71.
- Hummers WS, Offeman RE. Preparation of graphitic oxide. J Am Chem Soc. 1958;80(6):1339–1339.
- Soni A, Krishnamurthy R. Plants – the next generation treatment of leukemia. Indian J Plant Sci. 2013;2:117–125.
- Senthilkumar S, Dhivya V, Sathya M, et al. Synthesis and characterization of magnetite/hydroxyapatite nanoparticles for biomedical applications. J Exp Nanosci. 2021;16(1):160–180.
- Wei Y, Fang Z, Zheng L, et al. Green synthesis of Fe nanoparticles using Citrus maxima peels aqueous extracts. Mater Lett. 2016;185:384–386.
- Arunachalam KD, et al. One-step green synthesis and characterization of leaf extract-mediated biocompatible silver and gold nanoparticles from Memecylon umbellatum. Int J Nanomed. 2003;8:1307–1315.
- Harshiny M, Iswarya CN, Matheswaran M. Biogenic synthesis of iron nanoparticles using Amaranthus dubius leaf extract as a reducing agent. Powder Technol. 2015;286:744–749.
- Katata-Seru L, Moremedi T, Aremu OS, et al. Green synthesis of iron nanoparticles using Moringa oleifera extracts and their applications: removal of nitrate from water and antibacterial activity against Escherichia coli. J Mol Liq. 2018;256:296–304.
- Sangami S, Manu B. Synthesis of green iron nanoparticles using laterite and their application as a Fenton-like catalyst for the degradation of herbicide Ametryn in water. Environ Technol Innov. 2017;8:150–163.
- Beheshtkhoo N, Kouhbanani MAJ, Savardashtaki A, et al. Green synthesis of iron oxide nanoparticles by aqueous leaf extract of Daphne mezereum as a novel dye removing material. Appl Phys A. 2018;124(5):363–369.
- Radini IA, Hasan N, Malik MA, et al. Biosynthesis of iron nanoparticles using Trigonella foenum-graecum seed extract for photocatalytic methyl orange dye degradation and antibacterial applications. J Photochem Photobiol B. 2018;183:154–163.
- Cui H, Ren W, Lin P, et al. Structure control synthesis of iron oxide polymorph nanoparticles through an epoxide precipitation route. J Exp Nanosci. 2013;8(7–8):869–875.
- Patra JK, Baek K-H. Green biosynthesis of magnetic iron oxide (Fe3O4) nanoparticles using the aqueous extracts of food processing wastes under photo-catalyzed condition and investigation of their antimicrobial and antioxidant activity. J Photochem Photobiol B. 2017;173:291–300.
- Kuang Y, Wang Q, Chen Z, et al. Heterogeneous Fenton-like oxidation of monochlorobenzene using green synthesis of iron nanoparticles. J Colloid Interface Sci. 2013;410:67–73.
- Yew YP, Shameli K, Miyake M, et al. Green synthesis of magnetite (Fe3O4) nanoparticles using seaweed (Kappaphycus alvarezii) extract. Nanoscale Res Lett. 2016;11(1):276–282.
- Askarinejad A, Bagherzadeh M, Morsali A. Sonochemical fabrication and catalytic properties of α-Fe2O3 nanoparticles. J Exp Nanosci. 2011;6(3):217–225.
- Nurbas M, Ghorbanpoor H, Avci H. An eco-friendly approach to synthesis and charactrization of magnetite (Fe3O4) nanoparticles using Platanus orientalis L. leaf extract. Dig J Nanomater Biostruct. 2017;12:993–1000.
- Gautam A, Rawat S, Verma L, et al. Green synthesis of iron nanoparticle from extract of waste tea: an application for phenol red removal from aqueous solution. Environ Nanotechnol Monit Manage. 2018;10:377–387.
- Zhang S, Wu D, Li H, et al. Rapid identification of α-glucosidase inhibitors from Dioscorea opposita Thunb peel extract by enzyme functionalized Fe3O4 magnetic nanoparticles coupled with HPLC-MS/MS. Food Funct. 2017;8(9):3219–3227.
- Devatha C, Jagadeesh K, Patil M. Effect of green synthesized iron nanoparticles by Azadirachta indica in different proportions on antibacterial activity. Environ Nanotechnol Monit Manage. 2018;9:85–94.
- Akhtar S, Saba S, Rehman S, et al. Microemulsion-based synthesis of strontium hexaferrite cobalt iron oxide nanoparticles and their biocompatibility in albino mice. J Exp Nanosci. 2018;13(1):199–211.
- Tahvilian R, Zangeneh MM, Falahi H, et al. Green synthesis and chemical characterization of copper nanoparticles using Allium saralicum leaves and assessment of their cytotoxicity, antioxidant, antimicrobial, and cutaneous wound healing properties. Appl Organomet Chem. 2019;33(12):e5234.
- You C, Han C, Wang X, et al. The progress of silver nanoparticles in the antibacterial mechanism, clinical application and cytotoxicity. Mol Biol Rep. 2012;39(9):9193–9201.
- Mao B-H, Tsai J-C, Chen C-W, et al. Mechanisms of silver nanoparticle-induced toxicity and important role of autophagy. Nanotoxicology. 2016;10(8):1021–1040.
- Namvar F, Rahman HS, Mohamad R, et al. Cytotoxic effect of magnetic iron oxide nanoparticles synthesized via seaweed aqueous extract. Int J Nanomed. 2014;9:2479–2488.
- Sankar R, Maheswari R, Karthik S, et al. Anticancer activity of Ficus religiosa engineered copper oxide nanoparticles. Mater Sci Eng C Mater Biol Appl. 2014;44:234–239.