Abstract
In this study, iron nanoparticles were synthesised in aqueous medium using Calendula officinalis extract as stabilising and reducing agents. The synthesised nanoparticles (FeNPs) were characterised using different techniques, including UV–Vis. and FT-IR spectroscopy, X‐ray diffraction (XRD), scanning electron microscopy (SEM) and Energy Dispersive X-ray Spectrometry (EDS). According to the XRD analysis, 34.29 nm was measured for FeNPs crystal size. SEM images exhibited a uniform spherical morphology in size of 46.30 nm for the biosynthesized nanoparticles. TUNEL test was used to show apoptosis and DNA fragmentation. The mitochondrial membrane potential was studied by Rhodamine123 fluorescence dye. Iron nanoparticles-treated cell cutlers decreased the DNA fragmentation. They raised the mitochondrial membrane potential in the high concentration of Mitoxantrone-treated HDMVECn, HUVEC, HAEC, HCAEC, HCASMC and HPAEC cells. The best result of cardiovascular protective properties was seen in the high dose of iron nanoparticles, i.e. 4 µg. According to the above findings, iron nanoparticles containing Calendula officinalis leaf aqueous extract may be administrated as a cardiovascular protective supplement for treating cardiovascular diseases after approving in the clinical trial studies in humans.
1. Introduction
Calendula officinalis is a short-lived aromatic that grows to 80 cm tall. The plant has sparsely branched lax or erect stems. The leaves are oblong-lanceolate, hairy on both sides, and with margins entire or occasionally waved or weakly toothed. The inflorescences are yellow, comprising a thick capitulum or flowerhead 4–7 cm diameter surrounded by two rows of hairy bracts; in the wild plant they have a single ring of ray florets surrounding the central disc florets. C. officinalis is widely cultivated and can be grown easily in sunny locations in most kinds of soils. C. officinalis is used as a medicinal plant in traditional medicines of different cultures. Some people use the plant leaf for the prevention, control, and cure of various cancers, such as breast, ovarian, prostate and blood cancers [Citation1]. The plant is rich in antioxidant compounds, such as terpenoids, phenolic and flavonoids [Citation2–4].
The field of synthetic nanotechnology has developed the self-sufficiency of materials with specific sizes and shapes at the nanometre scale environmentally friendly. Nanomaterials can be created from the engineering of multiple or single molecules which are engineered and have several functional groups. Biocompatible nanocarriers include peptides, engineered antibodies, micelles, polymers and liposomes [Citation5–7]. Nanomaterials can be designed to gain the desired chemical and physical characteristics and purposefully direct drugs to dynamic tumour environment with high therapeutic effects and low toxicity. Features to be controlled when designing nanoparticles are surface to volume ratio, shape, size, molecular charge and drug release. For example, nanoparticles, after binding to functional chemical components such as antibodies and ligands, bind to the surface receptors of the cancer cell and prevent its clearance by the blood [Citation6–12].
Drug delivery by nanoparticles using biodegradable polymers solves many problems. In 1975, a drug-conjugated model of the polymer was proposed by Ringdorf, which increased drug delivery to the cancer cell site [Citation4–7]. She suggested that the conjugated pharmacological properties of drugs and polymers could be manipulated by altering the chemical and physical properties of the polymer. For example, a water-insoluble drug can be soluble by adding solvent components to a polymer. Therefore, it increases both bioavailability and biodegradability. For cytotoxic drugs to be efficiently delivered to cancer cell tissue, first, cytotoxic agents must be removed from the bloodstream and after leaving the extracellular space, the cancerous tissue must pass through the membrane surface and be targeted inside the cancer cell [Citation6–9]. The ideal properties of nano foundations that should be considered when designing are 1- Continuous drug release; 2- Inactive accumulation of the drug in cancerous tissue; 3- Targeting antigens or surface receptors of cancer cells with a controlling effect on endosomal uptake and membrane destruction. 4- Drug release into the cytoplasm and protection from enzymatic degradation [Citation5–8].
In the recent research, we decided to investigate the application of a novel cardiovascular protective drug formulated by iron nanoparticles containing Calendula officinalis on Mitoxantrone-induced cell death in HDMVECn, HUVEC, HAEC, HCAEC, HCASMC and HPAEC cell lines.
2. Material and methods
2.1. Materials
Bovine serum, 2,2-diphenyl-1-picrylhydrazyl (DPPH), dimethyl sulfoxide (DMSO), decamplmaneh foetal, 4-(dimethylamino) benzaldehyde, hydrolyzate, antimycotic antibiotic solution, Ehrlich solution and borax–sulfuric acid mixture, DMED, all were afforded from the US Sigma-Aldrich company.
2.2. Preparation and extraction of aqueous extract
After collection, Calendula officinalis leaves were kept away from direct sunlight and dried in the shade, and the powder was prepared by a grinder. To prepare the aqueous extract, 30 grams of dried Calendula officinalis powder was sandwiched on Whatman paper. To remove colour and fat, it was placed in a Soxhlet device with 500 mL of N-hexane and placed on the heater at 70 °C for 10 to 12 h, until the powder inside the Whatman paper is colourless and N-hexane coloured. The powder was then added to an Erlenmeyer flask containing 300 mL of water and then the mixture was boiled over a gentle heat for 15 min and filtered using Whatman No. 1 filter paper. Finally, it was concentrated by a rotary apparatus. With the help of a freezer dryer (Scientific LTE UK, Ltd), it was completely dried and turned into a powder. Finally, the dry powder was stored in a sealed glass container and refrigerated and used to prepare different concentrations.
2.3. Green synthesis and chemical characterisation of FeNPs
The biosynthesis of FeNPs was carried out according to the previous studies with some modification [Citation10]. A 10 mL of aqueous extract solution (20 mg/mL) was added to 30 mL of FeCl3·6H2O in the concentration of 0.02. The mixture was stirred for 90 min at 50 °C. The color-changing from yellow to black indicated the formation of iron nanoparticles. The precipitate was triplet washed with water and centrifuged at 12,000 rpm for 15 min subsequently. The obtained black powder was kept in a vial for the chemical characterisation and evaluation of its biological activity.
2.4. Cardiovascular protective analyses of iron nanoparticles
2.4.1. Cell culture
In this experiment, Human Dermal Microvascular Endothelial Cells; Neonatal (HDMVECn), Human Umbilical Vein Endothelial Cells (HUVEC), Human Aortic Endothelial Cells (HAEC), Human Coronary Artery Endothelial Cells (HCAEC), Human Coronary Artery Smooth Muscle Cells (HCASMC) and Human Pulmonary Artery Endothelial Cells (HPAEC) cell lines were cultured according to protocol rules in the gibco RPMI1640 cell culture environment. In first, 100 IU/mL penicillin (Sigma), 100 µg/mL streptomycin (Sigma) and 10%fetal bovine serum (FBS, Gibco) supported in cell cultures and standard case in T-25cm2 tissue culture bottles (5% incubated at 37 °C in CO2). It led to changes in the morphology of Mitoxantrone cells, so apoptotic cells led to the cell being treated with diluted Mitoxantrone with 100 mM water. For FeCl3·6H2O, Calendula officinalis leaf aqueous extract, and iron nanoparticles solutions in the RPMI1640 water cell cultural environment were first dissolved in DMSO and added to the cultural environment with a final volume of 0.1%. Then, 12 h after the plating and washing, they were classified into several groups including I) Mitoxantrone: Cell culture contains 100 μM Mitoxantrone; II) Control: Cell culture medium without Mitoxantrone, FeCl3·6H2O, Calendula officinalis leaf aqueous extract, and iron nanoparticles; III) T1: Cell culture contains 100 μM Mitoxantrone and 2 μg of FeCl3·6H2O; IV) T2: Cell culture contains 100 μM Mitoxantrone and 4 μg of FeCl3·6H2O; V) T3: Cell culture contains 100 μM Mitoxantrone and 2 μg of Calendula officinalis leaf aqueous extract; VI) T4: Cell culture contains 100 μM Mitoxantrone and 4 μg of Calendula officinalis leaf aqueous extract; VII) T5: Cell culture contains 100 μM Mitoxantrone and 2 μg of iron nanoparticles; VIII) T6: Cell culture contains 100 μM Mitoxantrone and 4 μg of iron nanoparticles [Citation11].
2.4.2. Cell death index
For determining of cell death index in the various treatments of I–VII, TUNEL staining was used Eight random wells were selected to count TUNEL positive cells with an Olympus AX-70 fluorescence microscope. The ratio of cell death index to apoptotic cells to total cells is equal [Citation11].
2.4.3. Mitochondrial membrane potential (MMP)
For half an hour different treatments were exposed to 10 mg/mL rhodamine-123. The cell was then washed using PBS. Subsequently, 900 μL triton X-100 was added to each well and held at 4 °C for 2 h. The solutions were taken into microtubes to be centrifuged at 16,000 rpm for 20 min. Fluorescent absorbing in cells was performed using a fluorescent microplate reader (488 nm excitation and 520 nm emissions) [Citation11].
2.5. Statistical analysis
The data obtained because of experimental studies were evaluated by using the SPSS-22 program and in coordination with Tukeys post hoc process with ANOVA (p ≤ 0.01).
3. Results and discussion
3.1. Chemical characterisation of FeNPs
3.1.1. UV–visible spectroscopy analysis C. officinalis
presents the UV–Vis. spectrum of green synthesised FeNPs using C. officinalis extract. The result confirms the creation of FeNPs. The peaks at 215 and 299 nm belong to the surface plasmon resonance (SPR) of FeNPs. The band is very close to a previously reported one for the green synthesised of FeNPs [Citation13,Citation14].
3.1.2. FT‐IR analysis
presents the FT-IR spectrum of FeNPs. The formation of FeNPs is approved by the presence of the peaks at 470, 549, and 597 cm−1 that relate to bending vibration of Fe–O. These peaks, with a small difference in the wavenumber, have been previously reported for the iron oxide nanoparticles [Citation15,Citation16]. FT-IR analysis is well known as an efficient method to screen the organic compound as the capping and reducing agents of ferric chloride precursor to FeNPs. The presences of different-IR bands correlate to the presences of various functional groups in C. officinalis extract. For example, the peaks at 3417 and 2925 cm−1 for O–H and C–H; the peaks at a range of 1471 to 1662 cm−1 for C = C and C = O; and peak at 1096 cm−1 for –C–O. These peaks can be considered for the presence of various secondary metabolites in C. officinalis extract such as phenolic, flavonoid, saponins, Quinones, Terpenoids which have been reported previously [Citation2–4]. In the green synthetic of metallic NPs, these types of compounds are known as reducing, stabilising and dispersing agents that bind to NPs through the functional groups such as hydroxyl and carbonyl [Citation17].
3.1.3. XRD analysis
The XRD diffraction patterns of FeNPs evaluated its crystallinity. The pattern of the diffractogram is showed in . The result approves the green synthesis of iron oxide. The peaks at 35.61(311), 40.52 (222), 53.01(422), 57.40 (511) and 62.38 (440) are matched as well as to those of standard database ICDD PDF card no. 00-015-0806. The crystallinity of the FeNPs has been approved clearly using the XRD diffractogram. The peaks and the related planes are also reported previously [Citation18]. The broad peak correlates to the presence of organic compounds in C. officinalis extract adsorbed on the surface of FeNPs in accordance with previous report [Citation19]. The FeNPs nanoparticles had an average crystal size of 34.29 nm. This size was calculated using X‐Ray diffraction according to the Scherrer equation.
3.1.4. SEM analysis
The morphology of FeNPs was assessed by the FE-SEM technique ((a–d)). The image exhibited the spherical morphology for the synthesised FeNPs, which has been reported previously [Citation20]. The images presented a uniformity and homogeneity for the FeNPs. Furthermore, FE-SEM images reveal a tendency to aggregate for ther nanoparticles that is a common property for the green synthetic of metallic nanoparticles such as FeNPs, CdNPs, CuNPs, AgNPs, TiNPs and NiNPs have been reported previously [Citation13,Citation17,Citation21–24]. An average size of 46.3 nm was obtained for FeNPs. In our literature review, 11.0–98.79 nm was reported for biosynthesized of iron oxide using plant extracts as the capping agent for NPs [Citation25–28].
The qualitative analysis of EDS was run to screen the elemental analysis of FeNPs. The result is shown in . The spectrum clearly shows the elemental composition profile of the FeNPs. The signals around 0.71 Kev (for FeLα), 6.44 Kev (for FeKα) and 7.13 Kev (for FeKβ) belong to iron in nanoparticles. These signals for the biosynthesized FeNPs have been reported by other research group [Citation28]. A single at 0.52 Kev shows the presence of oxygen and another one at 0.28 Kev belongs to carbon. The presence of oxygen and carbon approved the linkage between iron nanoparticles and organic compounds of the plant extract.
3.2. Cardiovascular protective potentials of iron nanoparticles green-synthesised by Calendula officinalis leaf aqueous extract
Mitoxantrone is prescribed as a chemotherapeutic drug and may be of concern because of its side effects [Citation29]. Abuse of this drug may affect the cardiovascular system cells; its metabolites may be harmful to the digestive and excretion system [Citation30]. The pathway for the breakdown of Mitoxantrone passes through the liver and kidneys, and consequently, the potential of side effects is high in these organs [Citation29,Citation30]. In a study, the effects of Mitoxantrone on the lung and brain (cerebral cortex and hippocampus) are revealed. They emphasised the role of oxidative stress, neuronal and pulmonary damage in the disruptive effects of Mitoxantrone abuse [Citation29–31].
Mitoxantrone has direct effects on the cardiovascular system and increases the reactive oxygen species (ROS) by reducing the level of antioxidant activity in the plasma. Therefore, it seems necessary to evaluate the toxic effect of Mitoxantrone in cardiovascular system cells and study on compounds that can decline these toxic effects [Citation29]. Several studies have indicated that Mitoxantrone increases the pro-inflammatory cytokines such as IL1α, IL1β, IL6, and TNFα and decrease the anti-inflammatory cytokines such as IL13, IL10, IL5, IL4 and IL3. The cytotoxicity properties of Mitoxantrone increase cell death significantly and reduce cell proliferation in cardiovascular system cells [Citation30,Citation31]. Also, a study showed that Mitoxantrone through apoptosis induction, caspase-3 and caspase-9 activation, and cell proliferation inhibition induced cell death in cells [Citation29]. The findings of our experiment also agreed with these results and revealed that Mitoxantrone at high concentrations reduced significantly (p ≤ 0.01) cell viability. Treatment of these cells with both doses of FeNPs synthesised using Calendula officinalis leaf aqueous extract increased the cell proliferation and cell viability potentials due to the cell cytotoxicity reduction.
The previous studies indicated that Mitoxantrone produced many free radicals, especially ROS in the body [Citation29–31]. Free radicals with the degradation of DNA molecules cause cellular degradation and apoptosis in the cells [Citation31]. ROS directly damages the DNA and increases the apoptosis in cardiovascular system cells through the production of free radicals [Citation29–31]. In our study, the experiment of apoptosis by the TUNEL test indicated that Mitoxantrone caused DNA fragmentation and induced apoptosis in HDMVECn, HUVEC, HAEC, HCAEC, HCASMC and HPAEC cells. Further experiments revealed that Mitoxantrone causes apoptosis in these cells by reducing the mitochondrial membrane potential. Our findings also indicated that iron nanoparticles synthesised using Calendula officinalis leaf aqueous extract significantly (p ≤ 0.01) increased the mitochondrial membrane potential and reduced the rate of DNA fragmentation in HDMVECn, HUVEC, HAEC, HCAEC, HCASMC and HPAEC cells treated with Mitoxantrone ( and ).
Figure 6. The apoptosis index of different treatments after 48 h. M: Mitoxantrone, C: Control, T1: 100 μM Mitoxantrone and 2 μg FeCl3·6H2O, T2: 100 μM Mitoxantrone and 4 μg FeCl3·6H2O, T3: 100 μM Mitoxantrone and 2 μg Calendula officinalis leaf aqueous extract, T4: 100 μM Mitoxantrone and 4 μg Calendula officinalis leaf aqueous extract, T5: 100 μM Mitoxantrone and 2 μg iron nanoparticles, T6: 100 μM Mitoxantrone and 4 μg of iron nanoparticles. A: HDMVECn, B: HUVEC, C: HAEC, D: HCAEC, E: HCASMC and F: HPAEC. *Indicate the significant difference (p ≤ 0.01) between experimental groups with Mitoxantrone group.
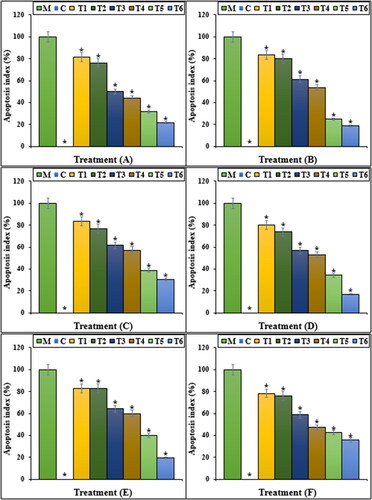
Figure 7. The Rh123 absorption of different treatments after 48 h. M: Mitoxantrone, C: Control, T1: 100 μM Mitoxantrone and 2 μg FeCl3·6H2O, T2: 100 μM Mitoxantrone and 4 μg FeCl3·6H2O, T3: 100 μM Mitoxantrone and 2 μg Calendula officinalis leaf aqueous extract, T4: 100 μM Mitoxantrone and 4 μg Calendula officinalis leaf aqueous extract, T5: 100 μM Mitoxantrone and 2 μg iron nanoparticles, T6: 100 μM Mitoxantrone and 4 μg of iron nanoparticles. A: HDMVECn, B: HUVEC, C: HAEC, D: HCAEC, E: HCASMC and F: HPAEC. *Indicate the significant difference (p ≤ 0.01) between experimental groups with Mitoxantrone group.
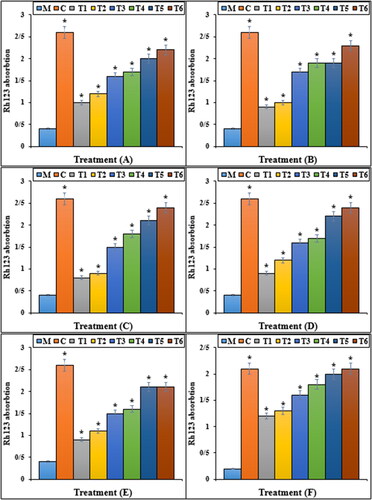
It seems that iron nanoparticles green-synthesised by Calendula officinalis leaf, due to its antioxidant potential, significantly (p ≤ 0.01) raised cell viability and mitochondrial membrane potential, and reduced DNA fragmentation in the high concentration of Mitoxantrone-treated HDMVECn, HUVEC, HAEC, HCAEC, HCASMC and HPAEC cells. Today, antioxidants are introduced as a reducer of cell cytotoxicity because they prevent ROS production and oxidative stresses in the cells [Citation11].
4. Conclusion
In this research, the iron oxide nanoparticles were produced from the reaction of C. officinalis leaf extract and FeCl3·6H2O. FE-SEM, UV–Vis and FT-IR methods were used to evaluate the major characteristics of the FeNPs. The relevant results revealed that iron nanoparticles had been successfully synthesised. Based on the FT-IR results, the presence of a large number of secondary metabolites produced appropriate conditions for reducing iron. In the FE-SEM technique, the average size of iron nanoparticles was assessed to be 46.3 nm, which is favourable in nanotechnology study.
In the biological experiments, we concluded that a high dose of Mitoxantrone causes cell death in HDMVECn, HUVEC, HAEC, HCAEC, HCASMC and HPAEC cell lines through the induction of cell inflammation and apoptosis. Iron nanoparticles enhanced cell viability in a high dose of Mitoxantrone-treated cells. They repressed mitochondrial membrane disruption activity. These events reveal that iron nanoparticles suppressed Mitoxantrone-induced cell death in a dose-dependent manner in HDMVECn, HUVEC, HAEC, HCAEC, HCASMC and HPAEC cell lines.
In the future, iron nanoparticles green-synthesised by C. officinalis leaf can be consumed for increasing the physiological activity of the cardiovascular system.
Acknowledgement
We acknowledge from Yuyan Wang, Hao Xia, Yan Zhou, Yong Huang, Saizhu Wu, Zhaoxi Wu, Liuying Li, Qinyang Li, Behnam Mahdavi and Zhaoning Wang for scientific supporting at the recent project.
Disclosure statement
No potential conflict of interest was reported by the authors.
Additional information
Funding
References
- Al-Snafi A. The chemical constituents and pharmacological effects of Calendula officinalis-A review. Indian J Pharm Sci Res. 2015;5(3):172–185.
- de Oliveira Carvalho H, Góes LDM, Cunha NMB, et al. Development and standardization of capsules and tablets containing Calendula officinalis L. hydroethanolic extract. Rev Latinoam Quím. 2018;46(1):16–27.
- Długosz M, Markowski M, Pączkowski C. Source of nitrogen as a factor limiting saponin production by hairy root and suspension cultures of Calendula officinalis L. Acta Physiol Plant. 2018;40(2):35.
- Verma PK, Raina R, Agarwal S, et al. Phytochemical ingredients and pharmacological potential of Calendula officinalis Linn. PBR. 2018;4(2):1–17.
- (a) Ghashghaii A, Hashemnia M, Nikousefat Z, et al. J Photochem Photobiol B Biol. 2019;192:103–112.
- (a) Abdoli M, Sadrjavadi K, Arkan E, et al. Bioorg Chem. 2020;106:104468.
- (a) Seydi N, Mahdavi B, Paydarfard S, et al. Appl Organomet Chem. 2019;33:e5164.
- Kooti W, Servatyari K, Behzadifar M, et al. Effective medicinal plant in cancer treatment, part 2: Review study. J Evid Based Complementary Altern Med. 2017;22(4):982–995.
- Hummers WS, Offeman RE. Preparation of graphitic oxide. J Am Chem Soc. 1958;80(6):1339–1339.
- Wei Y, Fang Z, Zheng L, et al. Green synthesis of Fe nanoparticles using Citrus maxima peels aqueous extracts. Mater Lett. 2016;185:384–386.
- Shaneza A, et al. Herbal treatment for the ovarian cancer. SGVU J Pharm Res Educ. 2018;3(2):325–329.
- Arunachalam KD, et al. One-step green synthesis and characterization of leaf extract-mediated biocompatible silver and gold nanoparticles from memecylon umbellatum. Int J Nanomedicine. 2003;8:1307–1315.
- Harshiny M, Iswarya CN, Matheswaran M. Biogenic synthesis of iron nanoparticles using Amaranthus dubius leaf extract as a reducing agent. Powder Technol. 2015;286:744–749.
- Katata-Seru L, Moremedi T, Aremu OS, et al. Green synthesis of iron nanoparticles using Moringa oleifera extracts and their applications: removal of nitrate from water and antibacterial activity against Escherichia coli. J Mol Liq. 2018;256:296–304.
- Sangami S, Manu B. Synthesis of green iron nanoparticles using laterite and their application as a fenton-like catalyst for the degradation of herbicide ametryn in water. Environ Technol Innov. 2017;8:150–163.
- Beheshtkhoo N, Kouhbanani MAJ, Savardashtaki A, et al. Green synthesis of iron oxide nanoparticles by aqueous leaf extract of Daphne mezereum as a novel dye removing material. Appl Phys A. 2018;124(5):363–369.
- Radini IA, Hasan N, Malik MA, et al. Biosynthesis of iron nanoparticles using Trigonella foenum-graecum seed extract for photocatalytic methyl orange dye degradation and antibacterial applications. J Photochem Photobiol B. 2018;183:154–163.
- Ghidan AY, Al-Antary TM, Awwad AM. Green synthesis of copper oxide nanoparticles using Punica granatum peels extract: effect on green peach aphid. Environ Nanotechnol Monit Manag. 2016;6:95–98.
- Zangeneh MM, Zangeneh A, Pirabbasi E, et al. Falcaria vulgaris leaf aqueous extract mediated synthesis of iron nanoparticles and their therapeutic potentials under in vitro and in vivo condition. Appl Organometal Chem. 2019;33(12):e5246.
- Lourenço IM, Pieretti JC, Nascimento MHM, et al. Eco-friendly synthesis of iron nanoparticles by green tea extract and cytotoxicity effects on tumoral and non-tumoral cell lines. Energ Ecol Environ. 2019;4(6):261–270.
- Devatha C, Thalla AK, Katte SY. Green synthesis of iron nanoparticles using different leaf extracts for treatment of domestic waste water. J Clean Prod. 2016;139:1425–1435.
- Rao MD, Pennathur G. Green synthesis and characterization of cadmium sulphide nanoparticles from Chlamydomonas reinhardtii and their application as photocatalysts. Mater Res Bull. 2017;85:64–73.
- Baghayeri M, Mahdavi B, Hosseinpor‐Mohsen Abadi Z, et al. Green synthesis of silver nanoparticles using water extract of salvia leriifolia: antibacterial studies and applications as catalysts in the electrochemical detection of nitrite. Appl Organometal Chem. 2018;32(2):e4057.
- Seydi N, Mahdavi B, Paydarfard S, et al. Preparation, characterization, and assessment of cytotoxicity, antioxidant, antibacterial, antifungal, and cutaneous wound healing properties of titanium nanoparticles using aqueous extract of Ziziphora clinopodioides Lam leaves. Appl Organomet Chem. 2019;33(9):e5009.
- Nurbas M, Ghorbanpoor H, Avci H. An eco-friendly approach to synthesis and charactrization of magnetite (Fe3O4) nanoparticles using Platanus orientalis L. leaf extract. Dig J Nanomater Biostruct (DJNB). 2017;12(4):993–1000.
- Gautam A, Rawat S, Verma L, et al. Green synthesis of iron nanoparticle from extract of waste tea: an application for phenol red removal from aqueous solution. Environ Nanotechnol Monit Manag. 2018;10:377–387.
- Zhang S, Wu D, Li H, et al. Rapid identification of α-glucosidase inhibitors from Dioscorea opposita Thunb peel extract by enzyme functionalized Fe3O4 magnetic nanoparticles coupled with HPLC-MS/MS. Food Funct. 2017;8(9):3219–3227.
- Devatha C, Jagadeesh K, Patil M. Effect of green synthesized iron nanoparticles by Azardirachta indica in different proportions on antibacterial activity. Environ Nanotechnol Monit Manag. 2018;9:85–94.
- Ghalie RG, Edan G, Laurent M, et al. Cardiac adverse effects associated with Mitoxantrone (Novantrone) therapy in patients with MS. Neurology. 2002;59(6):909–913.
- Kingwell E, Koch M, Leung B, et al. Cardiotoxicity and other adverse events associated with mitoxantrone treatment for MS. Neurology. 2010;74(22):1822–1826.
- Najafian J, Nasri A, Etemadifar M, et al. Late cardiotoxicity in MS patients treated with mitoxantrone. Int J Prev Med. 2019;10(1):211.