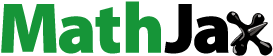
Abstract
Alhagi maurorum (camelthorn) is one of the popular medicinal plants. The plant has many pharmaceutical uses in traditional medicine. In this study, nickel nanoparticles were synthesized according to green chemistry rules using the aqueous extract of A. maurorum. The green-synthesized NiNPs were characterized using different techniques such as EDX, FE-SEM, XRD, UV–vis and FT-IR. The FE-SEM results confirm spherical morphology for the nanoparticles with size of 20.56–36.63 nm. In the oncological part of the present study, the treated cells with NiNPs were assessed by MTT assay for 48 h about the cytotoxicity and anti-human ovarian cancer properties on normal (HUVEC) and ovarian cancer cell lines i.e. OVCAR-3, ES-2, TOV-21G, OV-90 and UWB1.289. The viability of malignant ovarian cell lines reduced dose-dependently in the presence of NiNPs. The IC50 of NiNPs was 191, 312, 250, 396 and 241 µg/mL against OVCAR-3, ES-2, TOV-21G, OV-90 and UWB1.289 cell lines respectively.
1. Introduction
Alhagi maurorum or camelthorn, belongs to Fabaceae family. A. maurorum is a perennial herb that grows in various places in the world such as Asia, Europe and North Africa [Citation1, Citation2]. A. maurorum is well known as a medicinal plant with wide variety of applications in traditional medicine. The whole plant of A. maurorum has laxative, diuretic and expectorant properties [Citation3]. A. maurorum is effective agent to reduce gastric acidity [Citation4]. Diaphoretic, antiseptic, healing of wounds are the other medicinal properties of the plant [Citation5]. The leaves volatile oil of the plant is an efficient agent to cure rheumatism [Citation6]. According to the previous studies, phenolic compounds, glycosides, sterols, steroids, resins, alkaloids, flavonoids, fatty acids, coumarins and vitamins are the most important secondary metabolites in A. maurorum. Indeed, this variety of compounds in A. maurorum is responsible to wide spectrum of pharmaceutical uses of the plant [Citation4, Citation7–9].
Today, nanoparticle technology has made great strides in the production of many drugs, and the production of nanoparticles is one of the hopes in the effective treatment and diagnosis of many diseases, including cancer [Citation10–16]. Metallic nanoparticles have long been considered as a candidate for cancer treatment. Because natural metal oxides are present in large quantities in nature, the processing and synthesis of these nanoparticles can be one of the least expensive synthesis protocols [Citation17–23]. Metallic nanoparticles are one of the new types of widely used mineral particles that have been considered by researchers due to their suitable physical and chemical properties and at the same time, it has more adsorption power than other metallic nanoparticles-containing compounds [Citation24–30]. Metallic nanoparticles are one of the therapeutic compounds recognized by the US Department of Food and Drug Administration as a safe substance. Metallic nanoparticles are biocompatible and non-toxic and have also been used as medical fillers, cosmetics and drug carriers [Citation31–33]. Among the special properties of metallic nanoparticles are high chemical stability, low dielectric constant, high catalytic activity, absorption of infrared and ultraviolet light and most importantly its antibacterial properties. If the therapeutic and anticancer effects of these compounds are confirmed, this could be a significant step in advancing cancer therapies [Citation34–37].
In the current research, the properties of NiNPs against common ovarian cancer cell lines i.e. OVCAR-3, ES-2, TOV-21G, OV-90 and UWB1.289 were evaluated.
2. Material and methods
2.1. Preparation and extraction of aqueous extract
First, the dried leaves of A. maurorum were grounded. Then, 75 g of the sample was macerated in 800 mL of boiling water for 5 h. After that, the extract was filtrated and evaporated to concentrate. Finally, the extract was placed in a freeze drier for 72 h. The obtained extract as brown powder was kept in cold place.
2.2. Green synthesis and chemical characterization of NiNPs
The green synthesis of nickel nanoparticles was carried out according to a previous study [Citation38]. A 20 mL of A. maurorum extract (1 g in 10 mL of deionized water) was added to 50 mL of 15 mM NiSO4·6H2O in a flask. Then, to adjust of the pH, 2 mL of NaOH (1%) was added dropwise and shake for 25 min. Next the flask was put in an ultrasonic bath (75 W) for 30 min. The NiNPs was formed as green participates during the reaction time. The NiNPs was washed with water for three times and then centrifuged at 10,000 rpm for 15 min. Finally, the precipitate was dried in an oven at 45 °C.
2.3. Anti-human ovarian cancer properties of NiNPs
The process of the controlled culture of prokaryotic or eukaryotic cells in a filtered or unfiltered flask or cell culture plate by a suitable culture medium is called. This term is mostly used for culturing multicellular cells. Special culture media are used to culture cells. The cells are usually cultured at 37 °C in equipment such as CO2 incubators. Cell culture should be performed under aseptic (disinfected) conditions because the growth of these cells is much slower than the growth of bacteria and yeasts and there is a possibility of contamination of the culture medium. Antibiotics such as penicillin, streptomycin or gentamicin are sometimes used to stop the growth of bacteria. In order for cells to proliferate well in culture medium, their density in culture medium must be low. For this purpose, the cells should be passed to the fresh culture medium from time to time. One of the goals of cell culture is to study cells in terms of how they grow, their nutritional needs, and the reasons they stop growing, each of which can have a profound effect on the morphology of the cells we see under a microscope. Therefore, to study the cell growth cycle, develop methods to control the growth of cancer cells and modulate the expression of genes, it is necessary to cultivate these cells in the external environment [Citation39].
With the help of cell culture, cells can be prepared that are in different stages of differentiation and can be differentiated into other cells with the help of hormones and growth factors. With the help of cell culture, homogenous cells can be prepared and intracellular activities such as DNA replication, DNA transcription synthesis, RNA and protein synthesis and other details related to metabolism can be studied. It is also possible to examine the subsequent events and intracellular currents, such as the displacement of these complexes, the type of intracellular messages, and how the messages are transmitted, after connecting different molecules to the corresponding membrane receptor. The cultured cells can be stored frozen at very low temperatures. Such conditions will maintain the growth rate or genetic composition of these cells and can be thawed and used again at the appropriate time. This prevents the aging of cells, while it is currently not possible to prevent the aging of animals. When working with laboratory animals, systemic changes due to the effect of the animal's natural homeostasis or the stress of the experiments on the results should be considered. While the use of cell culture eliminates this problem. In addition, standardizing laboratory tests is easier and more practical than tests on living organisms. In laboratory environments, it is much easier to control the physical and chemical factors in the living environment of cells, including acidity, heat, osmotic pressure and the pressure of gases such as oxygen and carbon dioxide. Cells that are taken directly from the individual are known as primer cells and have a limited lifespan. Most cells have a limited lifespan, except for those taken from a tumour. An immortal cell line can proliferate indefinitely by creating a random or targeted mutation (such as artificial expression of the genus and be established as a representative of specific cell types [Citation39].
In this research, we used the following OVCAR-3, ES-2, TOV-21G, OV-90 and UWB1.289 cell lines to evaluating anti-human ovarian cancer and cytotoxicity effects of NiNPs using an MTT method.
In the recent study, the cells were cultured in medium (RPMI1640 = Roswell Park MemoryL Institute1640) with 10% FBS combined with penicillin and streptomycin antibiotics in an incubator containing 5% CO2 in a flask (T25). After three passages for purification, the cells were used to perform the next steps. Cell count and the number of viable cells were performed with a homocytometer slide using trypan blue. Evaluation of the cytotoxic effect of the NiNPs was performed by the modified 3-(4,5-dimethylthiazol-2-yl)-2,5-diphenyl-2H-tetrazolium bromide (MTT) colorimetric test. In this method, MTT, which is yellow, is converted to insoluble and formazan purple dye by the dehydrogenase enzymes in the mitochondria of active cells. The adsorption of this compound can be measured after dissolving at 540–570 nm. After 2 days and covering the flask bottom with cell, the cell layer adhering to the flask bottom was isolated enzymatically using trypsin-EDTA (5%) (tetra-acetic acid ethylenediamine), after transfer to sterile test tubes, it was centrifuged at 2000 rpm for 10 min. The cells were then resuspended in a fresh culture medium with the help of a Pasteur pipette and cell suspension (106 mL/μg) was prepared from them. 40 μL of this cell suspension (equivalent to 104×4 cells) was poured into 96-well plate flat-bottomed wells (for cell culture). Then the final volume of each well with 10% FBS medium reached 200 μL. The first-row containing cell suspension was considered as negative control (control). After incubation for 18–24 h to remove cells from the stress caused by trypsinization, the supernatant was removed slowly and carefully. A new medium was added to all rows with different concentrations of the NiNPs (only a new medium was added to the negative and positive control rows), so that the diluted NiNPs with concentrations of 1–1000 μg/mL was added to the third to sixth rows, respectively, the plate was incubated in CO2 for 24, 48 and 72 h. After the incubation time, the plate was taken out of the incubator and 20 μL of MTT (Sigma) was added to all wells, and incubated for 3 h. The supernatant was then gently removed and 100 μL of DMSO was added to the wells and pipetted to dissolve the formazan crystals. The amount of light absorption (OD) according to the intensity of the blue colour of formazan at 540 nm was read by Eliza reader. To convert OD to the percentage of living cells, the following formula was used and the percentage of cell life at each concentration was calculated after 48, 24 and 72 h [Citation39].
The concentration of the tested compounds that reduced the percentage of cell life by half was considered as IC50 (the half maximal inhibitory concentration) [Citation39].
2.4. Qualitative measurement
At least three independent replications were performed for each data and the result was presented as mean ± SD. Data statistical analysis was done with SPSS software version 19 and Anova Way One and Duncan’s test. Significance was considered at the level of p ≤ 0.05.
3. Results and discussion
3.1. Chemical characterization of NiNPs
3.1.1. EDX analysis
To characterize metallic nanoparticles, EDX is a qualitative technique to detect the elemental compositions of the NPs. shows the EDX diagram of NiNPs. The results approve the successful synthesis of nickel nanoparticles by the presences of the signals at 0.83, 7.46 and 8.15 keV for NiLα, NiKα and NiKβ respectively [Citation40]. The peak at 0.49 keV for OLα confirm the presences of oxygen in the NiNPs that shows the nanoparticles may be formed as nickel oxide, previous studies on green synthesis of NiNPs using plants extracts have reported the formation of nickel oxide. The other signal at 0.20 keV for CLα exhibits the presence of carbon in nanoparticles. This signal for carbon and also oxygen can be contributed to carbon and oxygen of secondary metabolite of A. maurorum extract that are linked to the surface of nanoparticles.
3.1.2. SEM analysis
FE-SEM technique is sufficient method to investigate morphology of nanoparticles. The FE-SEM images of NiNPs are shown if . The nanoparticles are formed in a spherical morphology in the range size of 20.56–36.63 nm. The green synthesized NiNPs exhibit a tendency to aggregate that is known as a general property for green synthetic metallic nanoparticles using plants extracts [Citation41–46].
3.1.3. XRD analysis
The XRD diffraction pattern is the method to evaluate compounds crystallinity. The XRD pattern of NiNPs is shown in . The formation of nickel nanoparticles with small size and well crystallizing is approved to this result. The obtained data for the signal at different of 2θ values were compared with the standard database of JCP2_47-1049. The signals at 27.24, 38.70, 43.21, 63.04, 75.41 and 79.67 are corresponded to (110), (111), (200), (220) and (311) planes. The crystal size of NiNPs was calculated using Scherer’s equation. The result revealed an 18.37 nm for the crystal size that was similar to the size of reported green synthetic nickel nanoparticles in literature [Citation41, Citation42, Citation47–49].
3.1.4. FT-IR analysis
FT-IR technique is another qualitative method in characterization of nanoparticles. The presence of the bands in the specific wavenumber regions reveals sufficient information about the metallic nanoparticles. For example, if the nanoparticles are formed as metal oxide, the peaks at 400–700 cm−1 belong to metal oxygen bond. The presences of the peaks at the other region are attributed to the different bonds for organic compounds in the plant extract that bind to the nanoparticles. The FT-IR spectra of NiNPs are shown in . The peaks at wavenumbers of 414, 474 and 617 cm−1 (belong to Ni–O bond) confirm the formation of NiO that is accordance to the XRD and EDX results. A previous study has reported the peaks for green synthetic NiNPs with a little difference in wavenumber [Citation41]
Furthermore, the peaks at 3404 and 2927 cm−1 (O–H and aliphatic C–H stretching), 1431–1675 cm−1 (C = C and C = O stretching), 1261 and 1085 cm−1 (–C–O and –C–N stretching) belong to the various bonds of organic compounds in A. maurorum extract that exist as the plant secondary metabolites. These compounds can be comprised different class of compounds such as phenolic, flavonoid, triterpenes, which were reported previously [Citation7–9].
3.1.5. UV–vis analysis
The surface plasmon resonance (SPR) of the nanoparticle can be analysed using UV–vis spectroscopy. The UV–vis spectrum of the green-synthetic nanoparticles of NiNPs is presented in . The creation of the green-synthetic NiNPs was approved by the results. There is a SPR band appearance at the wavelength of 341 nm that approved the formation of the nanoparticles. The band is very close to a previously reported on the green synthesized of NiO nanoparticles using plant extracts [Citation42].
3.2. Cytotoxicity and anti-human ovarian cancer activities of NiNPs
Cancer is recognized as one of the leading causes of death in today's society and several drugs have been introduced to treat this disease, but, most common cancers are not yet controllable and this disease imposes huge costs on the patient and society [Citation50–52]. The main factor in the development and progression of cancer has not yet been precisely identified, however, the available data suggest that metabolic disorders in the tissue and immune disorders may be involved in the development and exacerbation of this disease. In addition, metabolic disorders in the production and excretion of oxygen free radicals are important factors affecting cancer cells [Citation53–56]. Free radicals are destructive compounds that are produced as a by-product by the body's chemical reactions and are destroyed by the body's defense system and enzyme system and antioxidants. However, in cases where the body's metabolic disorders and the production of free radicals are high and they are not destroyed by the neutralizing system, due to their instability, these compounds have a strong tendency to react with a variety of molecules in the body [Citation51–54]. It is estimated that each cell in the human body is exposed to free radicals 10,000 times a day and DNA strands 5000 times a day. Damage to cell components includes proteins (genetic disorder), fats (lipid oxidation) and cell membranes (permeability disorder) that if the damage is not repaired, it leads to disruption of the chemical reaction and normal proteinization of the cell and the formation of harmful compounds and sometimes cancer cells in the body [Citation45–48]. It is reported that thousands of cancer cells are produced daily in the human body that are killed by the body's defense system. In some cases, due to dysfunction of the above systems, cancer cells proliferate and conditions for cancer development in different tissues [Citation51–55]. According to the above, antioxidants play a vital role in preventing disorders caused by the effects of free radicals and thus the prevention and treatment of cancer. Antioxidants are a wide range of molecular compounds with complex properties that combine with and neutralize free radicals. The results show that more than 60,000 types of molecular antioxidants have been identified so far. Antioxidants can be effective in three known ways to prevent and treat cancer; (1) destruction of free radicals, (2) strengthen the immune system to destroy cancer cells, (3) prevent the adhesion of cancer cells to other cells and prevent their proliferation [Citation52–56].
In this study, the treated cells with different concentrations of the present NiNPs were assessed by MTT assay for 48 h about the cytotoxicity properties on normal (HUVEC) and ovarian malignancy cell lines i.e. OVCAR-3, ES-2, TOV-21G, OV-90 and UWB1.289.
The absorbance rate was evaluated at 570 nm, which represented viability on normal cell line (HUVEC) even up to 1000 μg/mL for NiNPs ( and ). The viability of malignant ovarian cell line reduced dose-dependently in the presence of NiNPs. The IC50 of NiNPs was 191, 312, 250, 396 and 241 µg/mL against OVCAR-3, ES-2, TOV-21G, OV-90 and UWB1.289 cell lines respectively ( and ).
Figure 6. The anti-ovarian cancer properties (cell viability (%)) of NiNPs (concentrations of 0–1000 µg/mL) against OVCAR-3 (A) and ES-2 (B) cell lines.
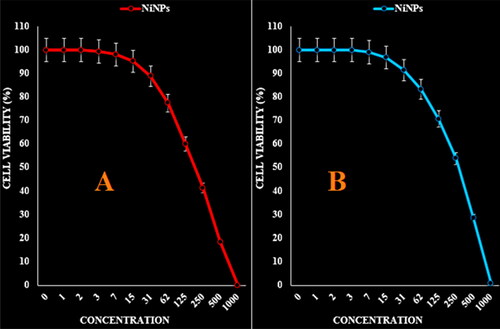
Figure 7. The anti-ovarian cancer properties (cell viability (%)) of NiNPs (concentrations of 0–1000 µg/mL) against TOV-21G (A) and OV-90 (B) cell lines.
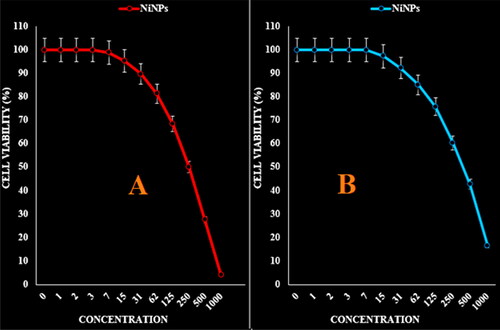
Figure 8. The anti-ovarian cancer properties (cell viability (%)) of NiNPs (concentrations of 0–1000 µg/mL) against UWB1.289 cell line.
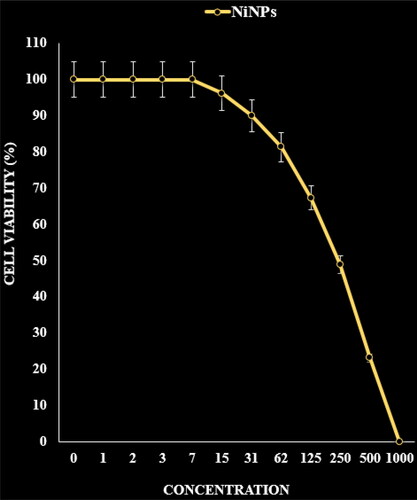
Table 1. The IC50 of NiNPs in the anti-ovarian cancer test.
It seems that the anti-human ovarian cancer effect of recent nanoparticles is due to their antioxidant effects. Because tumour progression is so closely linked to inflammation and oxidative stress, a compound with anti-inflammatory or antioxidant properties can be an anti-carcinogenic agent [Citation37, Citation38]. Many nanoparticles have pharmacological and biochemical properties, including antioxidant and anti-inflammatory properties, which appear to be involved in anticarcinogenic and antimutagenic activities [Citation38, Citation39]. Today, nanoparticles synthesized by biological methods play a vital role in treating many diseases, including cancer [Citation39].
4. Conclusion
In summarize, the nickel nanoparticles were green-synthesized using an aqueous extract of A. maurorum. The NiNPs were characterized using common chemical techniques such as UV–vis, FT-IR, XRD, FE-SEM and EDS. The results approved the synthesis of nanoparticles in form of nickel oxide nanoparticles with a spherical morphology. The size of NiNPs was in the range of 20.56–36.63 nm which is well known as an efficient size for the synthetic nanoparticles. The viability of malignant ovarian cell line reduced dose-dependently in the presence of NiNPs. The IC50 of NiNPs was 191, 312, 250, 396 and 241 µg/mL against OVCAR-3, ES-2, TOV-21G, OV-90 and UWB1.289 cell lines respectively. After clinical study, NiNPs can be utilized as an efficient drug in the treatment of ovarian cancer in humans.
Disclosure statement
No potential conflict of interest was reported by the author(s).
Additional information
Notes on contributors
Chaojun Yuan
• • •
References
- Hamed A, Perrone A, Mahalel U, et al. Oleanane glycosides from the roots of Alhagi maurorum. Phytochem Lett. 2012;5(4):782–787.
- Al-Saleem MSM, Al-Wahaib LH, Abdel-Mageed WM, et al. Antioxidant flavonoids from Alhagi maurorum with hepatoprotective effect. Phcog Mag. 2019;15(65):592.
- Akbar S. Handbook of 200 medicinal plants: a comprehensive review of their traditional medical uses and scientific justifications; 2020.
- Olas B, Hamed AI, Oleszek W, et al. Comparison of biological activity of phenolic fraction from roots of Alhagi maurorum with properties of commercial phenolic extracts and resveratrol. Platelets. 2015;26(8):788–794.
- Ahmad N, Shinwari ZK, Hussain J, et al. Phytochemicals, antibacterial and antioxidative investigations of Alhagi maurorum medik. Pak J Bot. 2015;47:121–124.
- Laghari AH, Ali Memon A, Memon S, et al. Determination of free phenolic acids and antioxidant capacity of methanolic extracts obtained from leaves and flowers of camel thorn (Alhagi maurorum). Nat Prod Res. 2012;26(2):173–176.
- Awaad Amani A, Maitland D, Soliman G. Antiulcerogenic activity of Alhagi maurorum. Pharm Biol. 2006;44(4):292–296.
- Laghari AH, Memon S, Nelofar A, et al. Alhagi maurorum: a convenient source of lupeol. Ind Crops Prod. 2011;34(1):1141–1145.
- Al-Snai A, Al-Kamel ML, Esmael ME. Antifungal effect of Alhagi maurorum phenolic extract. IOSR J Pharm. 2019;9:7–14.
- Maier-Hauff K, Ulrich F, Nestler D, et al. Efficacy and safety of intratumoral thermotherapy using magnetic iron-oxide nanoparticles combined with external beam radiotherapy on patients with recurrent glioblastoma multiforme. J Neurooncol. 2011;103(2):317–324.
- Kolosnjaj-Tabi J, di Corato R, Lartigue L, et al. Heat-generating iron oxide nanocubes: subtle “destructurators” of the tumoral microenvironment. ACS Nano. 2014;8(5):4268–4283.
- Bhattacharyya S, Kudgus RA, Bhattacharya R, et al. Inorganic nanoparticles in cancer therapy. Pharm Res. 2011;28(2):237–259.
- Hilger I, Kaiser WA. Iron oxide-based nanostructures for MRI and magnetic hyperthermia. Nanomedicine. 2012;7(9):1443–1459.
- Orel V, Shevchenko A, Romanov A, et al. Magnetic properties and antitumor effect of nanocomplexes of iron oxide and doxorubicin. Nanomedicine. 2015;11(1):47–55.
- Van Landeghem FK, Maier-Hauff K, Jordan A, et al. Post-mortem studies in glioblastoma patients treated with thermotherapy using magnetic nanoparticles. Biomaterials. 2009;30(1):52–57.
- Silva AC, Oliveira TR, Mamani JB, et al. Application of hyperthermia induced by superparamagnetic iron oxide nanoparticles in glioma treatment. Int J Nanomed. 2011;6:591–603.
- Johannsen M, Thiesen B, Wust P, et al. Magnetic nanoparticle hyperthermia for prostate cancer. Int J Hyperthermia. 2010;26(8):790–795.
- Bañobre-López M, Teijeiro A, Rivas J. Magnetic nanoparticle-based hyper-thermia for cancer treatment. Rep Pract Oncol Radiother. 2013;18(6):397–400.
- Klein S, Sommer A, Distel LV, et al. Superparamagnetic iron oxide nanoparticles as novel X-ray enhancer for low-dose radiation therapy. J Phys Chem B. 2014;118(23):6159–6166.
- Chatterjee DK, Fong LS, Zhang Y. Nanoparticles in photodynamic therapy: an emerging paradigm. Adv Drug Deliv Rev. 2008;60(15):1627–1637.
- Zhang AP, Sun YP. Photocatalytic killing effect of TiO2 nanoparticles on Ls- 174-t human colon carcinoma cells. World J Gastroenterol. 2004;10(21):3191–3193.
- Thevenot P, Cho J, Wavhal D, et al. Surface chemistry influences cancer killing effect of TiO2 nanoparticles. Nanomedicine. 2008;4(3):226–236.
- Colon J, Hsieh N, Ferguson A, et al. Cerium oxide nanoparticles protect gastrointestinal epithelium from radiation-induced damage by reduction of reactive oxygen species and upregulation of superoxide dismutase 2. Nanomedicine. 2010;6(5):698–705.
- Wason MS, Colon J, Das S, et al. Sensitization of pancreatic cancer cells to radiation by cerium oxide nanoparticle-induced ROS production. Nanomedicine. 2013;9(4):558–569.
- Tarnuzzer RW, Colon J, Patil S, et al. Vacancy engineered ceria nanostructures for protection from radiation-induced cellular damage. Nano Lett. 2005;5(12):2573–2577.
- Ali D, Alarifi S, Alkahtani S, et al. Cerium oxide nanoparticles induce oxidative stress and genotoxicity in human skin melanoma cells. Cell Biochem Biophys. 2015;71(3):1643–1651.
- Neri D, Supuran CT. Interfering with pH regulation in tumors as a therapeutic strategy. Nat Rev Drug Discov. 2011;10(10):767–777.
- Seo JW, Chung H, Kim MY, et al. Development of water-soluble single-crystalline TiO2 nanoparticles for photocatalytic cancer-cell treatment . Small. 2007;3(5):850–853.
- Hou Z, Zhang Y, Deng K, et al. UV-emitting upconversion-based TiO2 photosensitizing nanoplatform: near-infrared light mediated in vivo photodynamic therapy via mitochondria-involved apoptosis pathway. ACS Nano. 2015;9(3):2584–2599.
- Cui S, Yin D, Chen Y, et al. In vivo targeted deep-tissue photodynamic therapy based on near-infrared light triggered upconversion nanoconstruct. ACS Nano. 2013;7(1):676–688.
- Lucky SS, Idris NM, Li Z, et al. Titania coated upconversion nanoparticles for near-infrared light triggered photodynamic therapy. ACS Nano. 2015;9(1):191–205.
- Idris NM, Lucky SS, Li Z, et al. Photoactivation of core-shell titania coated upconversion nanoparticles and their effect on cell death. J Mater Chem B. 2014;2(40):7017–7026.
- Chen J, Patil S, Seal S, et al. Rare earth nanoparticles prevent retinal degeneration induced by intracellular peroxides. Nat Nanotechnol. 2006;1(2):142–150.
- Das M, Patil S, Bhargava N, et al. Auto-catalytic ceria nanoparticles offer neuroprotection to adult rat spinal cord neurons. Biomaterials. 2007;28(10):1918–1925.
- Korsvik C, Patil S, Seal S, et al. Superoxide dismutase mimetic properties exhibited by vacancy engineered ceria nanoparticles. Chem Commun. 2007;(10):1056–1058.
- Hong R, Han G, Fernandez JM, et al. Glutathione-mediated delivery and release using monolayer protected nanoparticle carriers. J Am Chem Soc. 2006;128(4):1078–1079.
- Zangeneh MM, Zangeneh A, Pirabbasi E, et al. Falcaria vulgaris leaf aqueous extract mediated synthesis of iron nanoparticles and their therapeutic potentials under in vitro and in vivo condition. Appl Organomet. Chem. 2019;33:e5246.
- Mahdavi B, Paydarfard S, Rezaei‐Seresht E, et al. Green synthesis of NiONPs using trigonella subenervis extract and its applications as a highly efficient electrochemical sensor, catalyst, and antibacterial agent. Appl Organomet Chem. 2021;35(8):e6264.
- Jalalvand AR, Zhaleh M, Goorani S, et al. Chemical characterization and antioxidant, cytotoxic, antibacterial, and antifungal properties of ethanolic extract of Allium saralicum R.M. Fritsch leaves rich in linolenic acid, methyl ester. J Photochem Photobiol B. 2019;192:103–112.
- Zhang Y, Mahdavi B, Mohammadhosseini M, et al. Green synthesis of NiO nanoparticles using Calendula officinalis extract: chemical charactrization, antioxidant, cytotoxicity, and anti-esophageal carcinoma properties. Arab J Chem. 2021;14(5):103105.
- Iqbal J, Abbasi BA, Mahmood T, et al. Green synthesis and characterizations of nickel oxide nanoparticles using leaf extract of Rhamnus virgata and their potential biological applications. Appl Organomet Chem. 2019;33(8):e4950.
- Mahdavi B, Paydarfard S, Zangeneh MM, et al. Assessment of antioxidant, cytotoxicity, antibacterial, antifungal, and cutaneous wound healing activities of green synthesized manganese nanoparticles using Ziziphora clinopodioides Lam leaves under in vitro and in vivo condition. Appl Organomet Chem. 2020;34(1):e5248.
- Mahdavi B, Saneei S, Qorbani M, et al. Ziziphora clinopodioides Lam leaves aqueous extract mediated synthesis of zinc nanoparticles and their antibacterial, antifungal, cytotoxicity, antioxidant, and cutaneous wound healing properties under in vitro and in vivo conditions. Appl Organomet Chem. 2019;33(11):e5164.
- Baghayeri M, Mahdavi B, Hosseinpor‐Mohsen Abadi Z, et al. Green synthesis of silver nanoparticles using water extract of Salvia leriifolia: antibacterial studies and applications as catalysts in the electrochemical detection of nitrite. Appl Organomet Chem. 2018;32(2):e4057.
- Nwanya AC, Ndipingwi MM, Ikpo CO, et al. Zea mays lea silk extract mediated synthesis of nickel oxide nanoparticles as positive electrode material for asymmetric supercabattery. J Alloys Compd. 2020;822:153581.
- Baranwal K, Dwivedi LM, Singh V. Guar gum mediated synthesis of NiO nanoparticles: an efficient catalyst for reduction of nitroarenes with sodium borohydride. Int J Biol Macromol. 2018;120:2431–2441.
- Rameshthangam P, Chitra JP. Synergistic anticancer effect of green synthesized nickel nanoparticles and quercetin extracted from Ocimum sanctum leaf extract. J Mater Sci Technol. 2018;34(3):508–522.
- Ibraheem F, Aziz MH, Fatima M, et al. In vitro cytotoxicity, MMP and ROS activity of green synthesized nickel oxide nanoparticles using extract of Terminalia chebula against MCF-7 cells. Mater Lett. 2019;234:129–133.
- Chen M, Zhang Y, Huang B, et al. Evaluation of the antitumor activity by Ni nanoparticles with verbascoside. J Nanomater. 2013;2013:26.
- Stewart B, Wild CP. World cancer report 2014. Lyon, France: International Agency for Research on Cancer, World Health Organization; 2014.
- Rasmussen JW, Martinez E, Louka P, et al. Zinc oxide nanoparticles for selective destruction of tumor cells and potential for drug delivery applications. Exp Opin Drug Deliv. 2010;7(9):1063–1077.
- Felice B, Prabhakaran MP, Rodríguez AP, et al. Drug delivery vehicles on a nano-engineering perspective. Mater Sci Eng C. 2014;41:178–195.
- Fernandes E, Ferreira JA, Peixoto A, et al. New trends in guided nanotherapies for digestive cancers: a systematic review. J Control Release. 2015;209:288–307.
- Caputo F, de Nicola M, Ghibelli L. Pharmacological potential of bioactive engineered nanomaterials. Biochem Pharmacol. 2014;92(1):112–130.
- Danhier F, Feron O, Preat V. To exploit the tumor microenvironment: passive and active tumor targeting of nanocarriers for anti-cancer drug delivery. J Control Release. 2010;148(2):135–146.
- Laurent S, Dutz S, Häfeli UO, et al. Magnetic fluid hyperthermia: focus on superparamagnetic iron oxide nanoparticles. Adv Colloid Interface Sci. 2011;166(1–2):8–23.