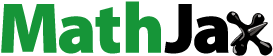
Abstract
Regarding applicative, facile, green chemical research, a bio-inspired approach is being reported for the synthesis of CuO nanoparticles by pectin (PEC) as a natural reducing and stabilizing agentin alkaline medium without using any toxic reducing agent. The biosynthesized CuO NPs@Pectin were characterized by advanced physicochemical techniques like ultraviolet–visible (UV–Vis), Fourier Transformed Infrared spectroscopy (FTIR), Scanning Electron Microscopy (SEM), Transmission Electron Microscopy (TEM), Energy Dispersive X-ray spectroscopy (EDX) and X-ray Diffraction (XRD) study. It has been established that pectin-stabilized copper nanoparticles have a spherical shape with a mean diameter from approximately 10 nm. To survey the cytotoxicity and anti-human cervical cancer effects of CuO NPs@Pectin, MTT assay was used on C-33 A [c-33a], SiHa, Ca Ski, DoTc2 4510, HT-3 and LM-MEL-41 cell lines. The resulting IC50 values of the CuO NPs@Pectin against C-33 A [c-33a], SiHa, Ca Ski, DoTc2 4510, HT-3 and LM-MEL-41 cell lines were 231, 214, 243, 376, 315 and 169 µg/mL, respectively. Antioxidant properties of CuO NPs@Pectin were surveyed through the DPPH test in presence of butylated hydroxytoluene as the positive control. The CuO NPs@Pectin inhibited half of the DPPH molecules in the concentration of 98 µg/mL. The cell viability of the treated cell lines was found to reduce dose-dependently over the CuO NPs@Pectin nanocomposite. After approving the above results in the clinical trial studies, the CuO NPs@Pectin can be used as a chemotherapeutic drug for the treatment of several types of cervical cancers.
1. Introduction
The amalgamation of advanced nanotechnology with natural product science has brought about a significant and novel implication in medicinal therapeutics [Citation1,Citation2]. The resulting nanomedicines have been experienced to serve as outstanding biocompatible clinical drugs in the treatment of several ailments [Citation3,Citation4]. In this regard, the design and development of targeted cancer nanomedicine has been a serious area of concern, considering the pharmacological restrictions of conventional cancer drugs. Recent studies have shown that, bio-formulated or bigenic nanomaterials exhibit exceptional anticancer properties during clinical trials, which are anticipated due to facile insertion of the bare material or itself carrying the effective drug into the affected cells, without damaging other healthy tissues [Citation5–11]. Several other reasons for the prominence of these bio-engineered nanomaterials are large surface area which in turn helps in higher drug loading capacity, very good biocompatibility, minimal toxic effects, controllable drug releasing competence and physicochemical stability towards intracellular fluids and other organic solvents [Citation12–14]. Earlier, assorted advanced functional materials like surface modified nanoparticles, quantum dots, nanomagnetic composites, engineered nanotubes and polyfunctionalized materials have been adopted as such novel nanomedicines [Citation15–21]. The current report is inspired by these perspectives and evidences. Hence, we are prompted to design and bio-synthesize pectin mediated and modified CuO nanoparticles (NP) following a green pathway. Pectin is a naturally occurring fiber which is found in fruits abundantly. Chemically, it is a complex polysaccharide consisting of d-galacturonic acid units bearing a number of COOH and OH functional groups [Citation22]. Such electron rich oxygenated environment is advantageous in complexing the surrounding Cu ions and subsequently in situ bio-reduction of them towards the nanoparticles. Thus, the synthesis of the NPs is also free from harsh chemicals and sustainably viable. Additionally, the as-synthesized NPs become encapsulated by the biomolecules which intrinsically stabilize them by capping and preventing to self-aggregate [Citation18]. Then, after the planned biogenic synthesis of the formulated nanomaterial, heading to bio-application, we deployed them in the study of anticancer activities against human cervical cancer.
Cervical cancer is one of the most prevalent and metastatic cancers in uterine region observed in women. It has been the fourth among most commonly observed human malignancies [Citation23,Citation24]. Among its different variants like Squamous cell carcinoma, adenocarcinoma and adenosquamous carcinoma, the first one is major and is caused by the human papillomavirus (HPV) [Citation25,Citation26]. Basic causes of cervical cancer are late menopause, ageing, obesity, high estrogen level, not having any children, high sugar level, etc. and the corresponding symptoms are vaginal and uterine bleeding, infertility, pain during sexual intercourse and urinal discharge, pelvic pain, etc. [Citation27,Citation28]. Surgery, chemotherapy, radiotherapy, targeted therapy and immunotherapy are the most common treatment procedure of this ailment [Citation29]. Different recommended chemotherapeutic anti-endometrial drugs used are bevacizumab, carboplatin, cis-platin, paclitaxel, docetaxel, doxorubicin, etc. However, the treatment efficiency of this procedure is not very satisfactory, resulting severe side-effects. Moreover, most of the drugs used are highly expensive [Citation30–32]. Therefore, strategic development for a novel, efficient and cost-effective nanotechnology based formulation is highly recommended.
Biomolecule engineered nanoparticle based alternate nanomedicines have come into prominence due to their increased efficiency, less cytotoxicity and minimal adverse effects as compared to conventional protocols. There are several reports on the biogenic nanoparticles showing considerable efficacy in the treatment of different cancers studied both in vitro and in vivo. In our protocol, the CuO NPs@Pectin nanocomposite has been administered on a diverse range of cervical cancer cell lines, such as C-33 A [c-33a], SiHa, Ca Ski, DoTc2 4510, HT-3 and LM-MEL-41. Cytotoxicity of the material over them was assessed by standard MTT method. In addition, we studied the antioxidant potential of CuO NPs@Pectin nanocomposite, which is believed to be an adjugated part of cancer study, being estimated by DPPH radical scavenging method.
2. Experimental
2.1. Synthesis of CuO NPs@Pectin
A very simple, clean and green method was followed in the synthesis of the CuO NPs@Pectin nanocomposite material. To initiate the reaction, 50 mL of a freshly prepared aqueous solution of Cu(OAc)2 (5 mM) was added to 50 mL aqueous solution of pectin (0.05 g) under ultrasonic conditions and left for 10 min at 60 °C. pH of the solution was then adjusted to 11.0 by addition of dilute NaOH solution (3 wt.%) and again kept under sonication for 2 h at 60 °C. Progress of the reaction was monitored by the change of light blue color solution to dark brown, indicating the formation of CuO NPs. The prepared CuO NPs@Pectin was collected by centrifugation followed by washing several times with DI-water. It was then dried at 60 °C in air.
2.2. DPPH assay protocol
Antioxidant activity can be monitored using the scavenging effect of radicals on DPPH (cat.no. D9132, Sigma-Aldrich Co., St. Louis, MO, USA). We tested compounds at five different concentrations (1000, 500, 250, 125, 62.5, 31.25 µg/mL). To do that, 150 µL of the sample was mixed with 150 µL of DPPH at concentration of 0.04 mg/mL. After 30 min incubation in 37, their absorbance at 517 nm was measured and used for calculating radical scavenging activity (% inhibition).
where Abssample was the absorbance of the reaction in presence of sample (sample dilution + DPPH solution), Absblank was the absorbance of the blank for each sample dilution (sample dilution + DPPH solvent) and Abscontrol was the absorbance of control reaction (sample solvent + DPPH solution).
UV Spectrophotometer Instrument: PowerWave XS, BioTek, Inc., Winooski, VT, USA
2.3. MTT assay protocol
The mentioned cell line was cultured at 1 105 cell/well in 96-well plates for 24 h at optimal conditions (37
5% CO2 in humidified incubator). Next, the growth media (10% FBS) was removed and the cells were washed two times with PBS. New maintenance RPMI medium (10% FBS) containing 0.5, 5, 50, 500 and 1000 µg/mL of each compounds was added and the cells were incubated 72 h. Triple wells were analyzed for each concentration and column elution buffer was used as the control. A 10 μL solution of freshly prepared 5 mg/mL MTT in PBS was added to each well and allowed to incubate for an additional 4 h. The media was removed and DMSO was added at 100 µL/well. Plates were shaken gently to facilitate formazan crystal solubilization. The absorbance was measured at 545 nm using a microplate reader. The percentages of cell toxicity and half-maximal inhibitory concentration (IC50) were calculated.
Microplate Reader Instrument: STAT FAX 2100, BioTek, Winooski, USA
3. Results and discussion
The CuO NPs@Pectin bio-nanocomposite material was synthesized following a green synthetic approach using pectin as a green reductant and stabilizing agent. During in situ synthesis, the biomolecular oxygenated organofunctions play as the reducing agent converting the anchored Cu2+ ions into Cu NPs which further aerially oxidized to CuO NPs on heating. After the sustainable synthesis of CuO NPs@Pectin nanocomposite material, it was the turn to characterize it following diverse physicochemical methods like UV–Vis and FTIR spectroscopy, FE-SEM, TEM, EDX and XRD analysis.
UV–Vis absorption spectroscopy is a typical procedure for the detection of noble metal NPs. The biochemical reduction over pectin solution was monitored as . After 100 min of reaction, CuO NPs were formed, being visibly detected from the color change of reaction solution from sky blue to deep brown as well as spectroscopically by the formation of characteristic surface plasmon resonance (SPR) at 400 nm.
Identification of the functional bonds in the as synthesized material was determined by FTIR analysis. depicts the co-plotted FTIR spectra of pectin solution and CuO NPs@Pectin nanocomposite in order to validate the pectin modification over the NPs. shows a broad vibrational band ranging from 3100 to 3400 cm−1 which is contributed by free hydroxyl groups and carboxyl hydroxyl groups (O-H stretching) of pectin and their intermolecular H-bonded structure. The characteristic functional vibrations observed at 2922, 1622, 1413 and 1058 cm−1 were corresponded to saturated hydrocarbons (Csp3-H stretching), C = O stretching, O-H bending and C-O stretching, respectively. The FTIR spectrum of to CuO NPs@Pectin is shown in . It clearly defines the pectin functionalization in the nanocomposite as all the vibrational peaks of pectin are evident into it. The Cu–O stretching vibrational bonds in CuO NPs are observed at 505 and approximately 592 cm−1.
represents the FE-SEM images of the quasi-spherical CuO NPs@Pectin nanoparticles.
Apparently the particles looks porous in nature. Functional modifications can be imagined from the surface coating over the particles. To have an idea of more detailed structure of the nanocomposite, TEM analysis was carried out ().
Morphology and intrinsic structure of CuO NPs@Pectin nanocomposite was determined by TEM analysis. All the particles are of uniform dimension and nearly monodisperse in nature. Diameters of the particles lie within 10–15 nm. As can be seen from , the particles are almost uniformly distributed without much agglomeration.
Finally, X-ray diffraction study was used to study the phase morphology and crystallinity. As the profile displays a single crystalline phase, evidently the molecule is a united entity (). A number of sharp diffraction peaks indicate that the molecule is highly crystalline in nature. The diffraction peaks observed at 2θ = 33.1°, 35.7°, 39.2°, 48.8°, 53.5°, 58.2°, 62.6°, 66.4°, 68.2°, 73.1° and 76.2° were attributed to diffraction on (1 1 0), (0 0 2), (1 1 1), (–2 0 2), (0 2 0), (2 0 2), (–1 1 3), (–3 1 1), (2 2 0), (3 1 1) and (–2 2 2) crystal planes, respectively, which are in close agreement with standard JCPDS values (File no. 01-080-0076) of monoclinic CuO NP [Citation33].
3.1. Antioxidant activity of CuO NPs@Pectin
In this study, the DPPH free radical scavenging potential of CuO NPs@Pectin was studied in several concentrations (31.25, 62.5, 125, 250, 500 and 1000 μg/mL). Aqueous MeOH (1:1) and butylated hydroxytoluene (BHT) were considered as negative and positive standards, respectively, in the analysis. The antioxidant property of CuO NPs@Pectin were determined by measuring the UV-absorbances (A) at 517 nm followed by the formula-
As can be seen in , with increase in the concentration of CuO NPs@Pectin the antioxidant activity was increased. The maximum DPPH scavenging activity for CuO NPs@Pectin at the highest concentration (1000 μg/mL) was 91.25%.
3.2. Cytotoxicity and anticancer results
Subsequently, the toxicity of CuO NPs@Pectin against cervical cancer cell lines Hela, HNCF-PI 52 and CCI-PI 19 were evaluated using the MTT method under different concentration. As the results displayed in , CuO NPs@Pectin have considerable toxicity effect on the Hela, HNCF-PI 52 and CCI-PI 19 cervical cancer cell lines and the toxicity gets increased significantly with increase in concentration of the nanocomposite. Its IC50 is calculated to be around 740, 838 and 900 µg/mL against Hela, HNCF-PI 52 and CCI-PI 19 cell lines, respectively (). Therefore, the nanocomposite displays best result with Hela cell lines. The anticancer effect was assessed by observing the changes in cell viability and morphology of cells, when the CuO NPs@Pectin nanocomposite was treated with the best cell line (Hela cells) in different concentrations, being observed under inverted light microscopy ().
Figure 10. Morphology of Hela cells after 24 h exposure of CuO NPs@Pectin with different concentrations (a) control; (b) 5 μg/mL; (c) 50 μg/mL, (d) 500 μg/mL, (e) 1000 μg/mL and (f) 2000 μg/mL.
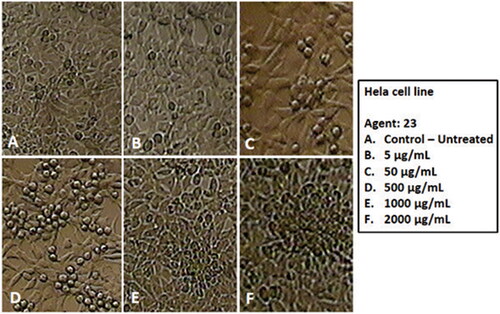
Table 1. The IC50 of CuO NPs@Pectin in the anti-human cervical cancer test.
It is anticipated that the potential anticervical cancer effect of our devised nanocomposite over the cervical cancer cell lines is due to their excellent antioxidant effects. As tumor progression is very closely linked to inflammation and oxidative stress, a compound with significant anti-inflammatory or antioxidant properties should have considerable anticarcinogenic activity and this we observed in our studies.
4. Conclusion
In this study, an eco-friendly approach described for in situ formation of CuO nanoparticles on the surface of pectin polymer, without using any toxic reducing and capping agents. The structure, morphology and physicochemical properties were characterized using UV–Vis and FTIR spectroscopy, FESEM, TEM, XRD and EDX analysis. Average diameters of the particles were approximately 10–15 nm. The CuO NPs@Pectin was assessed in biological applications like cytotoxicity and anticancer activities against common human cervical cancer cell lines, i.e., Hela, HNCF-PI 52 and CCI-PI 19. The viability of cervical cancer cell lines reduced dose-dependently in the presence of CuO NPs@Pectin nanocomposite. The CuO NPs@Pectin nanocomposite showed the best cytotoxicy against the Hela cell lines. It seems that the anti-human cervical cancer effect of recent nanoparticles is due to their antioxidant effects.
Notes on contributors
▪▪▪
Disclosure statement
No potential conflict of interest was reported by the authors.
References
- Namiki Y, Fuchigami T, Tada N, et al. Nanomedicine for cancer: lipid-based nanostructures for drug delivery and monitoring. Acc Chem Res. 2011;44:1080–1093.
- Saha B, Vlachos DG, Jouyandeh M, et al. Cutting-edge research for a greener sustainable future. Green Chem. 2021;23:4907–4915.
- Klimkowska MB, Poplawska M, Grudzinski IP. Nanocomposites as biomolecules delivery agents in nanomedicine. J Nanobiotechnol. 2019;48:17.
- Nagamune T. Biomolecular engineering for nanobio/bionanotechnology. Nano Converg. 2017;4(1):9.
- Wang J, Li Y, Nie G. Multifunctional biomolecule nanostructures for cancer therapy. Nat Rev Mater. 2021;6:766–783.
- Sun Q, Zhou Z, Qiu N, et al. Rational design of cancer nanomedicine: nanoproperty integration and synchronization. Adv Mater. 2017;29:1606628.
- Liu Z, Jiang W, Nam J, et al. Immunomodulating nanomedicine for cancer therapy. Nano Lett. 2018;18:6655–6659.
- Shi J, Kantoff PW, Wooster R, et al. Cancer nanomedicine: progress, challenges and opportunities. Nat Rev Cancer. 2017;17(1):20–37.
- Anselmo AC, Mitragotri S. Nanoparticles in the clinic: an update. Bioeng Transl Med. 2019;4:e10143.
- Mahendran D, Kavi Kishor PB, Geetha N, et al. Efficient antibacterial/biofilm, anti-cancer and photocatalytic potential of titanium dioxide nanocatalysts green synthesised using Gloriosa superba rhizome extract. J Exp Nanosci. 2021;16(1):11–31.
- Huang Y, Zhu C, Xie R, et al. Green synthesis of nickel nanoparticles using fumaria officinalis as a novel chemotherapeutic drug for the treatment of ovarian cancer. J Exp Nanosci. 2021;16(1):369–382.
- Björnmalm M, Thurecht KJ, Michael M, et al. Bridging bio-nano science and cancer nanomedicine. ACS Nano. 2017;11:9594–9613. −
- Senapati S, Mahanta AK, Kumar S, et al. Controlled drug delivery vehicles for cancer treatment and their performance. Signal Transduction Targeted Ther. 2018;3:7.
- Jabr-Milane L, van Vlerken L, Devalapally H, et al. Multi-functional nanocarriers for targeted delivery of drugs and genes. J Control Release. 2008;130:121–128.
- Bourzac K. Nanotechnology: carrying drugs. Nature. 2012;491:S58–S60.
- Rosenblum LT, Kosaka N, Mitsunaga M, et al. In vivo molecular imaging using nanomaterials: general in vivo characteristics of nano-sized reagents and applications for cancer diagnosis (review). Mol Membr Biol. 2010;27:274–285.
- Pescatori M, Davide B, Enrica V, et al. Functionalized carbon nanotubes as immunomodulator systems. Biomaterials. 2013;34:4395–4403.
- Xue W, Yang G, Karmakar B, et al. Sustainable synthesis of Cu NPs decorated on pectin modified Fe3O4 nanocomposite: catalytic synthesis of 1-substituted-1H-tetrazoles and in-vitro studies on its cytotoxicity and anti-colorectal adenocarcinoma effects on HT-29 cell lines. Arab J Chem. 2021;14:103306.
- Simon AT, Dutta D, Chattopadhyay A, et al. Quercetin-Loaded luminescent hydroxyapatite nanoparticles for theranostic application in monolayer and spheroid cultures of cervical cancer cell line in vitro. ACS Appl Bio Mater. 2021;4:4495–4506.
- Hunt NJ, Lockwood GP, Kang SWS, et al. Quantum dot nanomedicine formulations dramatically improve pharmacological properties and alter uptake pathways of metformin and nicotinamide mononucleotide in aging mice. ACS Nano. 2021;15:4710–4727.
- Sun W, Karmakar B, Ibrahium HA, et al. Design and synthesis of nano Cu/chitosan-starch bio-composite for the treatment of human thyroid carcinoma. Arab J Chem. 2022;15(1):103465.
- Buchanan BB, Gruissem W, Jones RL. 2000. Biochemistry and molecular biology of plants. Rockville (MD): American Society of Plant Biologists. ISBN 978-0-943088-37-2.
- Liu YC, Wu L, Tong RZ, et al. PD-1/PD-L1 inhibitors in cervical cancer. Front Pharmacol. 2019;10:65.
- Clarke MA, Long BJ, Morillo ADM, et al. Association of endometrial cancer risk with postmenopausal bleeding in women: a systematic review and meta-analysis. JAMA Intern Med. 2018;178:1210–1222.
- Lavanya G, Babusha K, Umesh M. Meeting the global need for radiation therapy in cervical cancer-An overview. Semin Radiat Oncol. 2020;30:348–354.
- Marshall C, Rajdev MA, Somarouthu B, et al. Overview of systemic treatment in recurrent and advanced cervical cancer: a primer for radiologists. Abdom Radiol (NY). 2019;44:1506–1519.
- Burke WM, Orr J, Leitao M, et al. Endometrial cancer: a review and current management strategies: part II. Gynecol Oncol. 2014;134:393–402.
- Zhou P, Liu W, Chen Y, et al. Nanoparticle-based applications for cervical cancer treatment in drug delivery, gene editing, and therapeutic cancer vaccines. Wiley Interdiscip Rev Nanomed Nanobiotechnol. 2021;13:e1718
- Cohen PA, Jhingran A, Oaknin A, et al. Cervical cancer. Lancet. 2019;393:169–182.
- Guillotin D, Martin SA. Exploiting DNA mismatch repair deficiency as a therapeutic strategy. Exp Cell Res. 2014;329(1):110–115.
- Chung HC, Ros W, Delord JP, et al. Efficacy and safety of pembrolizumab in previously treated advanced cervical cancer: results from the phase II KEYNOTE-158 Study. J Clin Oncol. 2019;37:1470–1478.
- Minion LE, Bai J, Monk BJ, et al. A markov model to evaluate cost-effectiveness of antiangiogenesis therapy using bevacizumab in advanced cervical cancer. Gynecol Oncol. 2015;137:490–496.
- Veisi H, Karmakar B, Tamoradi T, et al. Biosynthesis of CuO nanoparticles using aqueous extract of herbal tea (Stachys lavandulifolia) flowers and evaluation of its catalytic activity. Sci Rep. 2021;11(1):1983.