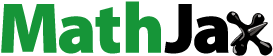
Abstract
In this work, we synthesized folate-conjugated dual-drug loaded double liposomes which are noted for their extremely high target specificity towards pituitary adenomas. It is known that while folate receptors are almost non-existent in normal tissues, they are overexpressed in non-functional pituitary adenomas. Synthesis, characterization and in vitro studies of folate-conjugated dual-drug loaded double liposomes for targeting non-functional pituitary adenomas is the highlight of this study. The size, zeta-potential, polydispersity index, in vitro release studies, stability of the nanoformulation, cytotoxicity and cellular uptake studies have been carried out. It was noted from the results that these are highly targeted liposomal formulation as expressed by the cellular uptake studies and are just sufficiently sized to escape the clearance mechanisms of body. They were also cytocompatible and were stable even after 60 days of shelf life with negligible changes in sizes, zeta potential as well as polydispersity index. The conjugation with folate particles resulted in the high specificity of the formulation to the specific targeted tissue as seen in cellular uptake by primary cell culture of non-functional pituitary adenomas. It may safely be concluded from the results that this approach may be a promising therapy for the future which has low cytotoxicity and high-specificity.
1. Introduction
Pituitary adenomas are typically recognized to be non-threatening and comparatively less aggressive. Nonetheless, one-third of the pituitary adenomas are invasive and display greater aggressiveness with increased probabilities of recurrences [Citation1–3]. These are termed as functional adenomas. But it is the clinically non-functional adenomas that are invasive and non-aggressive which are fatal, frequently recurrent and are resistant to established therapeutic approaches [Citation4,Citation5]. It is usually difficult for health care workers to point out an optimal therapeutic approach for these pituitary adenomas. The goals for therapeutic approaches are generally reduction in the tumor mass, suppression of the hormonal oversecretion, decompression of visual conduit and clearing the complications by decreasing the symptoms. Since these are not generally associated with hormone oversecretion, these are relatively diagnosed late. Additionally, the invasiveness and ability to infiltrate other nearby tissues make these adenomas incurable and uncontrolled via either surgery [Citation6] or radiation therapy. Moreover, complete removal of these is difficult to accomplish and recurrences are often. The conventional treatment options like surgery, radiation and chemotherapy are also associated with low specificity or non-targeted therapy, side effects which include increased cytotoxicity to normal adjacent tissues [Citation7,Citation8].
Hence, overcoming these shortcomings with the help of nanobiomedicine would be an ideal solution. Targeted approaches for anticancer therapeutics are proving to be an important facet to reduce the malignancy in several cases across the globe [Citation9,Citation10]. Fabrications of novel therapies which are based on biomaterials which can be manipulated at the molecular level and when are applied for clinical purposes are termed as nanoplatforms [Citation11]. These nanoplatforms are utilized to synthesize nanomedicines to target specific affected tissues which may show improvement after treatment [Citation12–14].
In our research, to reach this definitive goal, extensive labours have been applied to create a double liposome nanoplatform conjugated with folic acid and loaded with anticancer drugs to target the reduction of size of non-functional pituitary adenoma. Folic acid or folate is an essential vitamin which is extremely important for cell survival and is internalized within cells via folate receptors with great affinity or folate carrier protein with less affinity [Citation15,Citation16]. Incidentally, folate may also be used as target for tumor-homing since folate receptors have a precise and limited expression array in normal tissues and a high-level expression array in numerous human carcinomas [Citation17–20]. Investigations have revealed that non-functional pituitary adenomas exhibit a significant overexpression of folate receptor α than functional adenomas [Citation21–23]. These receptors promote folic acid uptake, growth of the adenomas and changes signal transduction too [Citation24–26]. Therefore, we hypothesized that presence of the folate receptors may assist in providing targeted therapy for non-functional pituitary adenomas.
Creating folate-conjugated double liposomes for targeting non-functional pituitary adenomas is a novelty. Liposomes are lipid carriers composed of lipid crystals that essentially consist of single or double lipid layers encasing an aqueous core [Citation27]. These are versatile, controlled drug delivery systems that are effective in transporting retained drugs within to specific targeted tissue [Citation28–31]. Therefore, we have designed the double-layered liposomes in a manner that the drugs would be loaded in the inner liposome’s aqueous core and the outer liposomes would be bigger along with being more sustainable [Citation32,Citation33]. The concept of outer liposome ensures safety of the drugs from being ruined by numerous enzymes [Citation34]. Therefore, these may be more beneficial than current traditional ones. The drugs loaded in the folate-conjugated double liposomes are temozolomide and cabergoline. Cabergoline is dopamine agonist (D2 receptor analog) which has been proven to cause tumor shrinkage in non-functional pituitary adenomas as has been reported in several case studies and investigations [Citation35–37]. On the other hand, temozolomide is an oral alkylating substance that has exhibited promising results in treatment of pituitary adenomas, albeit lower in non-functional than aggressive adenomas [Citation35,Citation38]. However, effects of these drugs individually are still being expanded where relapses are frequent. Hence, we hypothesize that synergistic effect of cabergoline and temozolomide encased in protective double-layered liposome released at a specific targeted site may prove to be reasonably effective. The combination therapy will result in greater chances of objective response against tumor and reduced chances of development of single drug resistance. In this study, we listed synthesis, characterization and in vitro studies with folate-conjugated drug-loaded double-layered liposome as compared to folate-conjugated drug-loaded inner liposome and unconjugated double-layered liposomes.
2. Materials and methods
2.1. Materials
Polyoxyethylene-bis-amine-4000, Stearylamine along with erythrosine were procured from Sigma Aldrich (Shanghai, People’s Republic of China). 1, 2-Distearoyl-snglycero-3-phosphoethanolamine (DSPE) and 1, 2-Distearoylsn-glycero-3-phosphocholine (DSPC) were procured from Lipoid, Germany. Variable diameter glass beads (BZ-04, 0.300–0.550 mm; BZ-3, 2.79–3.96 mm; and BZ-6, 5.61–6.70 mm) were obtained from Sigma Aldrich (Shanghai, People’s Republic of China). Temozolomide (TEM) and cabergoline (CAB) were obtained from Sun Pharmaceuticals (India). 0.5% DiI (1,1′-dioctadecyl-3,3,3′,3′-tetramethylindocarbocyanine perchlorate was obtained from Beyotime, China. Other reagents along with chemicals were procured from Sigma Aldrich unless mentioned otherwise.
2.2. Synthesis of the liposomes
2.2.1. Activation of folic acid
The process was followed from Verma et al. [Citation27] with adequate modifications to suit our study [Citation30,Citation39]. 2 ml of anhydrous dimethyl sulphoxide (DMSO) and 50 μL of triethylamine was used to dissolve 100 mg of folic acid. Consequently, 50 mg of N-hydroxysuccinimide (NHS) was added to the mixture in presence of 90 mg dicyclohexylcarbodiimide. The mixture was stirred through the night at room temperature. The spin-off product so obtained was dicyclohexylurea which was filtered and kept apart. DMSO concentration process was carried out by heating and pressure reduction following NHS-folate precipitation was done in diethylether. The NHS-folate obtained finally was washed with anhydrous ether numerous times followed by vacuum drying resulting in a yellow powder.
2.2.2. Synthesis of Folate-NH2
An equimolar quantity of NHS-folate was treated with polyoxyethylene-bis-amine-4000 in 5 ml DMSO which also contained 1 M equivalent of dicyclohexylcarbodiimide along with 10 μL of pyridine. The reaction mixture was constantly stirred for 12 h in a dark room. Following this, 10 ml of Milli-Q water was supplemented and dicyclohexylurea, which remained insoluble, was removed using centrifugation. The supernatant was dialyzed against 5 mM sodium bicarbonate buffer with pH 9.0. This solution was again dialyzed against deionized water for the removal of DMSO and unreacted folic acid in the assortment. Lyophilization of the obtained folate-NH2 was then done [Citation29,Citation30].
2.2.3. Conjugation of DSPE with Folate-NH2 and its activation
Initially, 1.0 M equivalent of succinic anhydride was reacted overnight with 100 mg of DSPE and 5 ml chloroform with 10 μl of pyridine. Cold acetone was utilized for the precipitation of the product. Using chloroform, N-Succinyl-DSPE was dissolved again and activation of its carbon group was carried out by treating with 1 M equivalent dicyclohexylcarbodiimide for about 5 h at room temperature. Synthesized folate-NH2 was dissolved in chloroform in an equimolar quantity following the addition of activated DSPE. The solvent was separated from the prepared mixture by the process of continuous stirring for 10–15 h at room temperature and lipid pellet comprising of folate-DSPE conjugate was cleaned twice using cold acetone. The substance so obtained was again dissolved in chloroform, and kept at −20 °C.
2.2.4. Preparation of inner liposomes
The inner casting method was employed to synthesize the inner liposomes. The mixture of 5 mL chloroform and methanol was prepared in the ratio of 80:20 (v/v) in a round bottom flask (RBF). DSPC, cholesterol (CHOL) & SA (Stearyl amine) were dissolved in the above mixture at molar ratios of 7.5:2.5:0.5. 10 mg of temozolomide was dissolved in 2 ml of ethanol (90% v/v) and this was then mixed with lipid solution prepared above. A rotary evaporator (Rotavapor, Buchi, Germany was used to evaporate the organic phase of solution in vacuum at 50 °C at 70 rpm at 230–250 V to obtain a fine film lining on the internal wall of the flask. A fine dried film of the lipid mixture was formed and hydrated with PBS (Phosphate-buffered saline, 10 ml) having pH 7.4 which also contained 10 mg of cabergoline. The resultant mixture was sonicated at 40% output frequency at 40 W for 15 min in 30 s on and off mode. Vortexing of the obtained inner liposomes was done for 15 min for removal of surplus residual organic solvent to acquire the inner liposomes. Inner liposomes conjugated with folate and loaded with drugs were termed as FOL-DUAL-ILs.
2.2.5. Preparation of double liposomes
Double liposomes were synthesized following a process from [Citation40] with slight modifications. DSPC, cholesterol & folate-DSPE prepared as mentioned above were mixed in chloroform in various molar ratios. The mixture was kept in RBF having the glass beads (BZ 0.350–0.500 mm). For formation of the lipid film, the organic solvent was vaporized at room temperature. Hydration of this film was done by the suspension of inner liposome formed beyond the phase transition temperature of the lipids with gentle shaking for one hour. Sonication of the mixture was carried out for 10 min at 40 W and output frequency of 40% by an ultrasonicator. Centrifugation method was utilized to separate the double liposomes so formed and they would henceforth be termed as FOL-DUAL-DLs. Folate unconjugated double liposomes were also formed which were prepared in exactly same manner, except that instead of folate-DSPE, only DSPE was used and they would henceforth be termed as UN-DUAL-DLs. Hence the three types of liposomes formed were FOL-DUAL-ILs, FOL-DUAL-DLs and UN-DUAL-DLs. Part of liposomes was fluorescently labelled by added 0.5% DiI to lipid mixture and dried under reduced nitrogen pressure followed by lyophilization. The lipid mixture was hydrated in HEPES buffer and then fluorescein isothiocyanate (FITC) (100 mM) loaded in 0.4 M NaOH was added followed by repeated freezing and thawing. After FITC was encapsulated, the free FITC was removed by gel chromatography. This was used for cellular uptake experiments. The procedure was adopted from Kamps et al.
2.3. Characterization of the liposomes
2.3.1. Fourier Transform infrared spectroscopy (FTIR) of the conjugate and liposomal formulations
Lyophilized folate-DSPE conjugate, FOL-DUAL-ILs, FOL-DUAL-DLs, UN-DUAL-DLs free temozolomide and cabergoline were mixed with potassium bromide (KBr) and pellets of the liposomes were prepared. These were then scanned with a Perkin-Elmer (USA) spectrophotometer from a range of 4000–400 cm−1 at room temperature.
2.3.2. Size distribution, polydispersity index (PDI) and Zeta potential of the liposomal formulations
The size distribution, zeta potential and PDI of the liposomal formulations were noted by Malvern Zetasizer ZS 90 (UK). Centrifugation of the liposomal samples at 13,000 rpm for 20 min was carried out for the estimation of zeta potential. Pellets formed were again suspended in ultra-purified Milli-Q water at 25 °C.
2.3.3. Vesicular count/mm3 of liposomal formulation
The counting of the liposomal vesicles was carried out following Verma et al. [Citation27]. 5 times dilution of the liposomal formulation was carried out with 0.9% w/v NaCl solution following which the number of liposomal vesicles/mm3 was calculated by a haemocytometer (Germany) and optical microscope. Counting of the liposomal vesicles was done in every 80 small squares of haemocytometer and the total number of vesicles/mm3 was calculated according to the following formula:
2.3.4 Percentage of entrapment and loading efficiency of drugs in liposomal formulation
Entrapment efficiency (EE %) of the drugs trapped in the liposomes was calculated using a method explained earlier [Citation27]. The liposomes were purified using mini-column centrifugation technique. HPLC was done for estimation of drugs utilizing water, acetonitrile and tetrahydrofuran in the ratio of 65:30:5 (v/v/v) respectively. The pH was adjusted to 3.0 with perchloric acid over Phenomenex Luna C-18 (2) column in isocratic manner. The flow rate was adjusted to 1.0 ml/min with detection by UV at 313 nm where the injection volume was adjusted to 20 μL [Citation41]. The percentage of entrapment efficiency was calculated according to the following formula:
(1)
(1)
(2)
(2)
2.4. Morphology of the liposomes formed
2.4.1. Phase contrast microscopy
Liposomes formed (FOL-DUAL-ILs, FOL-DUAL-DLs and UN-DUAL-DLs) were fixed on glass slides and observed under Leica phase contrast microscope (Germany). The photomicrographs were captured at appropriate magnification.
2.4.2. Cryo-transmission electron microscopy
Liposomes formed (FOL-DUAL-ILs, FOL-DUAL-DLs and UN-DUAL-DLs) were observed under transmission electron microscope (Hitachi, Japan) aided with accelerating voltage of 120KV. The synthesis of samples was carried out in precise atmosphere of vitrification arrangement at 25 °C and 100% relative humidity. Single drop of the sample was positioned on a carbon coated copper grid which produces a thin film on the grid. Liquid ethane bath was used to dip the sample followed by cooling utilizing liquid nitrogen and finally observed under TEM at 180 °C. The photomicrographs of the samples were captured.
2.5. Stability studies of the liposomes
The stability of liposomes formed (FOL-DUAL-ILs, FOL-DUAL-DLs and UN-DUAL-DLs) was tested at 37 °C. 10 mg of lyophilized powders were dispersed in 50 ml of Milli-Q water by sonication. Visual observation of resolving of liposomes were carefully made monitored by calculation of size distribution, polydispersity index and zeta potential of the liposomes at consistent time interlude of 10, 20, 30 days by Delsa Nano C Zetasizer to understand their aggregation.
2.6. In vitro release studies
Dialysis tube method as elaborated by [Citation34,Citation42] was applied to study the release of drug(s) from FOL-DUAL-ILs, FOL-DUAL-DLs and UN-DUAL-DLs in PBS whose pH was adjusted to 7.4 as release medium. Dialysis tube with 2.4 nm porosity comprising 2 mL of FOL-DUAL-ILs, FOL-DUAL-DLs and UN-DUAL-DLs were retained in 100 ml of release medium accompanied with incessant stirring at 38 ± 1 °C. After suitable time gaps, 1 ml of solution was withdrawn followed by immediate renewal with equal volume of fresh media for replenishment of withdrawn medium. The amount of drugs temozolomide and cabergoline was carried out by HPLC utilizing water, acetonitrile and tetrahydrofuran in the ratio of 65:30:5 (v/v/v) respectively. The pH was adjusted to 3.0 with perchloric acid over Phenomenex Luna C-18 column in isocratic manner. The flow rate was adjusted to 1.0 ml/min with detection by UV at 313 nm where the injection volume was adjusted to 20 μL.
2.7. Haematological toxicity
The animal studies were duly done as permitted by the Institute Small Animal Ethics Committee. The committee had approved the study vide ethical approval number 39/CCH/2019. The protocol for the animal studies was presented in front of the committee and changes suggested by them were duly incorporated. The protocol was duly followed from Verma et al. [Citation27] with necessary modifications to suit our study. For studying the haematological toxicity, white albino Wistar rats were used. The animals were procured from Vital River Laboratory Animal Technology Co. Ltd, Beijing, China.
The ARRIVE guidelines 2.0 were followed during the study and the ARRIVE checklist is attached separately. For all the procedures mentioned, anaesthesia was induced with 0.6% pentobarbital sodium (1 ml/100 g). The Wistar rats weighed approximately 120 ± 10 g. The experimental rats were divided into 7 groups of 5 each. PBS, free temozolomide, free cabergoline, temozolomide and cabergoline mixture, FOL-DUAL-ILs, FOL-DUAL-DLs and UN-DUAL-DLs were intravenously administered to Group 1, 2, 3, 4, 5, 6, 7 respectively each day at an equal dose of 1 mg of free drug(s) in 1 ml of PBS where the pH was adjusted to 7.4 per kg body weight of rat. The drug concentration in the liposomal formulations were adjusted to the free drug concentration of 1 mg/ml. the free drugs, drug mixture, PBS and the liposomal formulations were all injected via the tail vein of rat. Estimation of the total white blood corpuscles (WBC), red blood corpuscles (RBC), platelets count and haemoglobin (Hb) content were carried out to acquire the haematological parameters on the 8th day of experiment aided with a partially-automated blood cell counter (Sysmex cc-130, Japan).
2.8. Uptake of the liposomal formulation by pituitary adenoma cells
2.8.1. Primary cell culture
2.8.1.1. Materials
Accustase enzyme and RPMI 1640 obtained from Invitrogen, China, deoxyribonuclease procured from Sigma, Beijing, China. Cell strainer was obtained from BD Biosciences China. Fetal bovine serum (FBS), B-27 Supplement, N-2 Supplement, streptomycin, penicillin, epidermal growth factor (EGF) and basic fibroblast growth factor (BFGF) procured from Gibco, Beijing China.
2.8.1.2. Procedure
The primary cell cultures were obtained from 10 cases of non-functional pituitary adenomas that have been reported in the hospital over a time period of one year. The procedure was submitted to the Human Ethical Research Committee (HERC) of the university and approved before the initiation of the studies. For generation of primary cell cultures, the tumors were extracted and pulverised followed by Accutase digestion comprising 1-mg deoxyribonuclease for one hour at physiological temperature. Following digestion, a cell strainer was used to filter cell suspensions which was washed for two times in PBS, and cultivated in folate-free RPMI 1640 media. The media was supplemented with 10% FBS, B-27 Supplement, N-2 Supplement, penicillin (100 U/mL), streptomycin (100 µg/mL), EGF (20 ng/mL), and BFGF (20 ng/mL).
2.8.1.3. Uptake of the liposomal formulations:
Primary cell cultures were cultured within cell sphere, resuspended by transitory usage of Accutase, followed by washing with folate-free RPMI 1640 thrice. Gestation of the cells was done in 6-well plates treated with 100 µL Matrigel Basement Membrane Matrix (BD Biosciences) along with folate-free RPMI 1640 enhanced with penicillin and streptomycin for 2 days before the initiation of uptake of cells. After 2 days, the incubation of the cells was carried out on coverslips with free temozolomide, free cabergoline, temozolomide and cabergoline mixture, DiI and FITC loaded (0.06 mg/ml) FOL-DUAL-ILs, FOL-DUAL-DLs and UN-DUAL-DLs at similar concentrations of 1 µg/mL for 2 h as elaborated by Darshana et al. [Citation43] and Chu et al. [Citation44]. Following incubation, the cells were meticulously washed with PBS thrice and secured with 1% paraformaldehyde for 12 min at room temperature. 4′,6-diamidino-2-phenylindole (DAPI), a fluorescent stain was used for staining the cells and the coverslips were washed with PBS thrice following which 2.5% 1,4-diazabicyclo [2.2.2] octane (DABCO) on glass slides was used for mounting the coverslips and sealed. For image acquisition Zeiss LSM 510 confocal microscope (Germany) was employed at 60X. Image analysis was carried out with LSM Image Browser software to perceive the internalization of liposomal formulation. For quantitative analysis, 104 cells were washed meticulously with PBS followed by trypsinization, harvesting and cell acquisition on FACS Calibur (BD Biosciences, USA). The results were analysed by means of Cell Quest software.
2.8.2. Statistical analysis for the quantitative assay
Values of the error bars represented the relative Standard Deviations. One-way analysis of variance (ANOVA) was used to determine statistically significant differences between groups. Value of p ≤ 0.01 was considered to be statistically significant both when FOL-DUAL-ILs was compared with others and FOL-DUAL-DLs compared with rest.
2.9. Cytotoxicity assay
The procedure was adapted from Chu et al. [Citation44] with few modifications. The cell line used was primary tracheal epithelial cells (ATCC®PCS-300-014). The cell growth was carried out in Dulbecco’s modified Eagle’s medium (DMEM) augmented with 10% FCS, penicillin (100 IU/mL) and streptomycin (100 mg/mL). Cell growth was maintained in humidified environment comprising 5% CO2 at 37 °C. The cytotoxicity of the free drugs, drug mixture and liposomal formulations were assessed by means of an MTT assay. Concisely, cell seeding was done in 96-well plates at a density of 5 × 103 cells/well and the cells were gestated for 24 h under 5% CO2 at 37 °C. Then, the cells were treated with PBS (Control), free temozolomide, free cabergoline, temozolomide and cabergoline mixture, FOL-DUAL-ILs, FOL-DUAL-DLs and UN-DUAL-DLs for 6 h. The drug concentration of the FOL-DUAL-ILs, FOL-DUAL-DLs and UN-DUAL-DLs were adjusted to the free drug concentration of temozolomide and cabergoline which was 2.5 µg/ml. Accordingly, the drug concentration of temozolomide and cabergoline mixture was also adjusted to be 2.5 µg/ml. After the incubation, 20 µl of MTT solution was supplemented in each well followed by gestation for 4 h. Thereafter the removal of media, 200 µl of DMSO was added. At a wavelength of 570 nm, absorbance of the samples was noted and compared after mild shaking for 10 min. Determination of cell viability was done by comparing the absorbance of all samples. Cell viability of human corneal epithelial cultures was also deliberated with Live/Dead cytotoxicity kit obtained from Invitrogen™ wherein live cells and dead cells appear green and red respectively. The procedure was carried out as per the directives of the manufacturer.
2.9.1. Statistical data analysis
The experiments including cell assays were carried in triplicate and the data were represented with their corresponding relative standard deviations (RSD). Error bars represent the relative standard deviation.
3. Results
3.1. Characterization of the liposomes
3.1.1. FTIR of the conjugate and liposomal formulations
Lyophilized folate-DSPE conjugate, FOL-DUAL-ILs, FOL-DUAL-DLs and UN-DUAL-DLs were prepared by dicyclohexylcarbodiimide procedure. The conjugation of folate with DSPE in the liposomal formulations was recognized by the IR investigation of the formed composite as displayed in . The FTIR elucidated the structural chemical composition and the rearrangement of the bonding of the constituents in FOL-DUAL-DLs, FOL-DUAL-ILs, UN-DUAL-DLs and folate-DSPE conjugate in comparison with free temozolomide and cabergoline. The drug loaded nanoparticles exhibited molecular vibrations of the folate group and other functional groups. From , we can clearly see the characteristic peak of folic acid (845.6 cm−1 for C-H bending and 1699.0 cm−1 for stretching and bending of aromatic C = C) visible in the lyophilized folate-DSPE conjugate along with both liposomal formulations except UN-DUAL-DLs where there was no folate conjugation. The peak for DSPE (2917.6 cm−1 for the asymmetric stearoyl stretching of –CH2- bond) was also visible in all liposomal formulations. Interestingly, it was found that peaks corresponding to N-H stretch (3430.6 cm−1) and C = O stretch (1650.1 cm−1) established the amide connection of the formed folate-DSPE conjugate in both liposomal formulations except UN-DUAL-DLs where there was no folate conjugation. The characteristic 1681.81 cm−1 (-COOH), 1647.1 cm−1 (-CONH) of free temozolomide and cabergoline and also visible in FOL-DUAL-DLs, FOL-DUAL-ILs, UN-DUAL-DLs which were indicative of absorption of drugs in the nanoparticles.
3.1.2. Size distribution, polydispersity index (PDI) and Zeta potential of the liposomal formulations
The size distribution, PDI and zeta potential of the liposomal formulations are exhibited in .
Table 1. Size distribution, polydispersity index (PDI) and Zeta potential of the liposomal formulations.
3.1.3. Vesicular count/mm3 of liposomal formulation
The number of vesicles/mm3 was found to be 14, 19 and 12 for FOL-DUAL-ILs, FOL-DUAL-DLs and UN-DUAL-DLs respectively.
3.1.4. Entrapment efficiency (EE) and loading efficiency (LE) of drugs in liposomal formulation
The EEs and LEs are denoted in . The EE of FOL-DUAL-DLs was calculated to be 79.7 ± 2.9% and 75.3 ± 2.7% for temozolomide & cabergoline respectively. For FOL-DUAL-ILs, it was calculated to be 56.2 ± 2.3% and 51.3 ± 1.7% for temozolomide & cabergoline respectively. Finally, for UN-DUAL-DLs, it was calculated to be 72.7 ± 4.1% and 66.3 ± 1.5% for temozolomide & cabergoline respectively. The LE of FOL-DUAL-DLs was calculated to be 44.3 ± 3.7% and 49.8 ± 3.3% for temozolomide & cabergoline respectively. For FOL-DUAL-ILs, it was calculated to be 23.4 ± 3.2% and 24.7 ± 2.1% for temozolomide & cabergoline respectively. Finally, for UN-DUAL-DLs, it was calculated to be 41.6 ± 4.1% and 39.2 ± 3.6% for temozolomide & cabergoline respectively.
Table 2. EE (%) for temozolomide and cabergoline in the liposomal formulations.
3.2. Morphology of the liposomal formulations
3.2.1. Phase contrast microscopy
The phase contrast micrographic images are elaborated in . The FOL-DUAL-ILs was of spherical shape without any rough edges as seen from the figures. The FOL-DUAL-DLs was clearly noted owing to their double-membraned structure. They were also sometimes concentric circles with more than two liposomes included within the layer seen in . depicted the irregular shaped UN-DUAL-DLs although the shapes were asymmetrical. Overall, it seemed a homogenous spread of the liposomal formulations without any agglomerations.
3.2.2. Cryo-TEM microscopy
The photomicrographic images of cryo-TEM are depicted in . The figure clearly augmented the finding of phase contrast microscopy where the concentric circles were visible in case of double liposomes in . However the inner liposomes appeared to be single spheres with traces of drug loaded within. The uniform distribution of liposomal formulations without any agglomeration was seen here too.
3.2.3. Stability studies of the liposomes
The differences sizes, zeta potential and PDI of FOL-DUAL-ILs, FOL-DUAL-DLs and UN-DUAL-DLs are noted in . The parameters were measured over a time gap of 10, 20 and 30 days. As may be seen, there are non-significant decreases in the sizes, zeta potential values and PDI of FOL-DUAL-ILs, FOL-DUAL-DLs and UN-DUAL-DLs, the reasons for which will be discussed later. However, since the values remain positive and the changes insignificant, the stability of the formulations remained intact, as may be assumed. There were also no visual signs of aggregation of the liposomal aggregation under microscope.
Table 3. Size, zeta potential and PDI of FOL-DUAL-ILs, FOL-DUAL-DLs and UN-DUAL-DLs after 10, 20 & 30 days during stability studies.
3.3. In vitro release studies
The in vitro release studies depicted an interesting trend as seen in and 4 D. It was seen that in PBS with pH 7.4 (), the percentage of cumulative release of temozolomide and cabergoline from FOL-DUAL-ILs are 78.2 ± 3% and 68.3 ± 4% respectively upto a time period of 72 h whereas in FOL-DUAL-DLs, the percentage of cumulative drug release was 52.3 ± 3% for temozolomide and 43.2 ± 3% for cabergoline respectively. In case of UN-DUAL-DLs, the cumulative drug release percentage was 62.1 ± 3% and 46.3 ± 5% for temozolomide and cabergoline respectively.
Figure 4. In vitro release studies of temozolomide and cabergoline respectively 4 A & 4B pH 7.4 (normal physiological milieu) 4 C & 4 D pH 5.0 (tumor milieu).
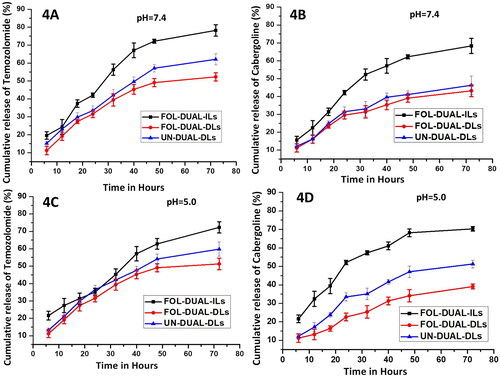
elaborated the release of drugs in pH = 5 representing the tumor milieu where the percentage of cumulative release of temozolomide and cabergoline from FOL-DUAL-ILs are 72.3 ± 2% and 70.3 ± 1% respectively upto a time period of 72 h whereas in FOL-DUAL-DLs, the percentage of cumulative drug release was 51.3 ± 3% for temozolomide and 39.2 ± 2% for cabergoline respectively. In case of UN-DUAL-DLs, the cumulative drug release percentage was 59.8 ± 4% and 51.5 ± 2% for temozolomide and cabergoline respectively.
3.4. Haematological toxicity
All the results were expressed as mean ± standard deviation. Statistical analysis was implemented by applying two way ANOVA and the results were statistically significant where p < 0.05. The results were depicted in . It was seen that the lowest WBC, RBC, platelets count and haemoglobin was seen in samples treated with free temozolomide + cabergoline mixture followed by free cabergoline and free temozolomide. The highest counts were recorded with samples treated with FOL-DUAL-DLs which were followed by UN-DUAL-DLs which are also comparable with control.
Table 4. Haematological parameters observed in rats post intravenous inject of the free drugs, mixture and liposomal formulations.
3.5. Uptake of the liposomal formulations
The qualitative uptake of the free drugs and liposomal formulations by primary cell cultures obtained from non-functional pituitary adenomas upon administration was viewed using confocal microscopy. The cells were stained with DAPI and it was seen that the cellular uptake by primary adenoma cells occurred in a time-dependent manner. The cells which were treated with folate conjugated formulations, i.e. FOL-DUAL-ILs and FOL-DUAL-DLs emitted stronger fluorescence than others due to DiI staining (5 D & 5E). The free drugs and the drugs mixture emitted weaker fluorescence than UN-DUAL-DLs (5 A, 5B, 5 C, and 5 F) as can be clearly depicted in . The lower uptake of UN-DUAL-DLs by the cells was owed to the absence of folate. The uptake of liposomal formulation was maximum during the initial 3 h as depicted by the qualitative uptake. The red and green stained liposomes were seen over surface and within cells. DAPI stained the live cells blue. It was seen that the images as captured within 3 h depicted destroyed cells in case of FOL-DUAL-ILs and FOL-DUAL-DLs. The cells did not uptake the free drugs and drug mixture as quick as others.
Figure 5. Qualitative uptakes of the free drugs and liposomal formulations by primary cell cultures 5 A free temozolomide; 5B free cabergoline; 5 C cabergoline and temozolomide mixture; 5 D FOL-DUAL-ILs; 5E FOL-DUAL-DLs; 5 F UN-DUAL-DLs.
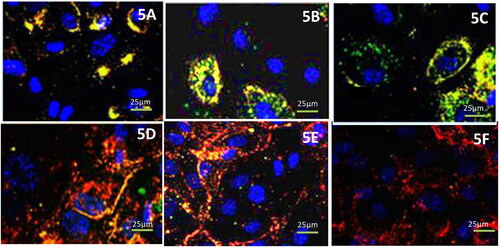
The quantitative uptake was elaborated by FACS assay depicted in which augmented the results obtained by flow cytometry. The cellular uptake of the FOL-DUAL-ILs and FOL-DUAL-DLs was enhanced owing to folate conjugation when compared to the free drugs and UN-DUAL-DLs at each time points (p < 0.1). Moreover, noteworthy changes were observed (p < 0.1) in cellular uptake from 0.5 to 3 h indicating modifications with folate may lead to increased cellular uptake.
3.6. Cytotoxicity assay
The cytotoxicity studies were performed using primary tracheal epithelial cells. illustrated the results of MTT assay where it was evident that there was almost negligible cytotoxicity associated with liposomal formulations when compared to control whereas the free drugs and drugs mixture exhibited significantly greater cytotoxicity.
Figure 7. % Cellular viability on being treated with FOL-DUAL-DLs, FOL-DUAL-ILs, UN-DUAL-DLs, PBS (control), free temozolomide, free cabergoline and temozolomide-cabergoline mixture.
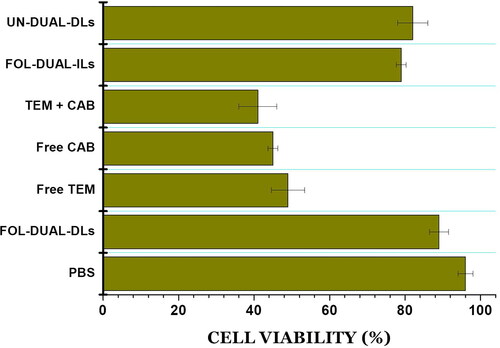
Cell viability of primary tracheal epithelial cultures was determined additionally by Live/Dead Kit where live and dead cells stain green and red respectively. The cells treated with the liposomal formulation and control had maximum number of cellular population stained green whereas the cells treated with free drugs and drug mixture had greater population of dead cells which had stained red. The images are depicted in .
4. Discussion
Double liposomes loaded with multiple drugs are a unique approach in site-targeted delivery since it’s a very dynamic method. The surfaces of the double liposomes so created interact with the cellular surface which enhances the uptake of the synthesized liposomes [Citation27]. The inner casting method used for the fabrication of the inner liposomes was very efficient in synthesizing a monodispersed colloidal solution as indicated by the values of PDI. The FOL-DUAL-ILs so synthesized was uniformly spherical in shape, had low PDI values and could be efficiently in the preparation of FOL-DUAL-DLs and UN-DUAL-DLs. The UN-DUAL-DLs were prepared for comparison with the folate conjugated double liposomes. The sizes of the liposomes synthesized were large enough not to be cleared by reticuloendothelial system. The twice increased sizes of FOL-DUAL-DLs and UN-DUAL-DLs indicated successful trapping of drugs in the core of the double liposomes. The phase contrast microscopy images also bore a testimony to this fact.
In the non-functional pituitary adenomas, overexpression of the folate receptor encoding gene (FOLR1 gene) has been found which was incidentally not the case with hormone-secreting adenomas [Citation22,Citation45]. Hence folate-conjugated liposomes would definitely be better targeted for drug delivery to the adenomas. As viewed from the stability studies of these liposomes, the negligible decrease in sizes over the time period of 30 days may correspond to the reorganization of the folic acid on the liposomal surface and edges. The loaded temozolomide and cabergoline also may have rearranged themselves in the core of inner liposomes in the FOL-DUAL-DLs and UN-DUAL-DLs. The pattern of in vitro drug release was uncertain in FOL-DUAL-ILs, FOL-DUAL-DLs and UN-DUAL-DLs formulations. As expected, in pH 7.4 () there was noteworthy differences in the in vitro release of drug from the prepared FOL-DUAL-DLs and FOL-DUAL ILs. In the first three days, the FOL-DUAL-ILs released about 3/4th of loaded temozolomide and cabergoline. This may be due to the fact that that inner liposomes prepared are single layered and the drug release is comparatively easy. Or it was also possible that drug released was not absorbed in the core of the liposome but only adhered temporarily under the surface of the inner liposome. This was supported by the in vitro release profiles of FOL-DUAL-DLs and UN-DUAL-DLs where the release of drugs was quite slow in comparison. Double layered liposomes scored an edge over single layered ones as being stable in biological media and provided sustained release of the loaded drugs. Drug release studies at pH = 5 represented tumor milieu strengthened the fact that double liposomes achieved a sustained release of drugs at acidic pH. The release of drugs from FOL-DUAL-ILs was quite high as compared to the other two liposomal formulation. This ability of double liposomes to remain stable in the acidic pH and causing slow release of the drugs would provide added advantage in forthcoming therapeutic uses of the same. The slow and continued release of drugs may also be due to the protective bilayer of lipids in the liposomes [Citation27].
Ostensibly, a decrease in count of WBC, RBC, haemoglobin and platelets observed after administration of free temozolomide, cabergoline, temozolomide and cabergoline mixture when compared to the liposomal formulations. This may be attributed to the immediate interaction of free drugs with the blood. The co-encapsulation of drugs in liposomal formulation reduced the haematological toxicity owing to the shielding and cytocompatible properties of lipids engaged in in synthesizing FOL-DUAL-ILs, FOL-DUAL-DLs and UN-DUAL-DLs which leads to negligible escape or percolation (leach) of drugs in blood. Moreover, haemolytic toxicity is an important parameter since it played a noteworthy role in limiting the utility of drug delivery carriers [Citation46]. The lack of haemolytic activity specified a safe drug carrier to the targeted tissue. Especially in the acidic pH, there were chances that due to hydrophobicity of the drugs, there may be appearances of partitions in the lipid bilayer causing disruption and release of drugs. This would have eventually led to erythrocyte lysis. But the increased RBC concentration negated any such happening which led us to believe that the liposomal formulations were hemocompatible. Before discussing the uptake of the liposomal formulation we will discuss the cytotoxicity of free drugs, drugs mixture and FOL-DUAL-ILs, FOL-DUAL-DLs and UN-DUAL-DLs since similar results were obtained. Prevention of the unnecessary drugs from the liposomes in the cellular environment due to the cytocompatible lipid bilayer also led to the minimal cell death as can be seen from the images () and MTT assay (). The uncoupled liposomal formulation (UN-DUAL-DLs) also exhibited significant cytocompatibility which was ensured by the cytoprotective layer. Moreover, since the primary tracheal cells did not possess the folate receptors, these liposomal formulations did not interact with these cells. The interesting phenomenon of the non-interaction of the uncoupled or unconjugated UN-DUAL-DLs was not exactly clear or understood.
Cellular uptake was an altogether interesting study. Specific binding of the liposomal formulation is an important prerequisite for cellular uptake but not a necessity on its own. These liposome encapsulated drugs need to deliver the drugs intracellular. There are essentially three routes for transferring drugs in specific cells. First, after specific cell binding the liposomes, they release the contents in vicinity of target cells. Secondly, they fuse with the cells releasing liposomes in cytosol and the third way is internalizing the liposomes via endocytosis and intracellular release of the drugs [Citation47]. We assume that in our case it was the second mechanism applicable since the images depict that unconjugated labelled UN-DUAL-DLs were indistinctly stained with DAPI with weaker fluorescence as was with the free drugs and drugs mixture since they weren’t stained. The cells which were treated with labelled FOL-DUAL-ILs and FOL-DUAL-DLs exhibited very strong fluorescence for initial 72 h after which there was no fluorescence which may be an indication that they were dead. Hence we believed that the prepared liposomal formulation were more effective than free drugs or drug mixture since they were cytocompatible with normal cells and effective against tumor cells.
5. Conclusion
While compiling all the results together, we arrived at the conclusion that the synthesized liposomal formulations were effective for targeted drug delivery to non-functional pituitary adenoma cells. We had aimed to prepare multiple drug loaded double liposomes which would be stable and would effectively bind with non-functional pituitary adenoma cells to release the drugs within. From the results it was evident that we have attained our goal. We had also synthesized two other formulations along with the double liposomes- inner liposomes loaded with dual drugs conjugated with folic acid (FOL-DUAL-ILs) and folate unconjugated dual drug loaded double liposomes (UN-DUAL-DLs). This was done to establish the effectiveness of folate conjugated dual drug loaded double liposomes. We found that the FOL-DUAL-DLs were much superior to FOL-DUAL-DLs or UN-DUAL-DLs in terms of in vitro drug release, uptake by cells, cytocompatibility, stability and entrapment of drugs. The data presented in our study does prove that folate conjugation helps in development of novel therapeutic applications for non-functional pituitary adenomas. Our study provides novel thrust for localization of drug carrier vehicles in cells and ways to improve liposomal uptake by cells. This may ensure vital evidences on the communication between the liposomal formulation and the target cell. However, the cellular uptake of the liposomal formulation does not guarantee improved therapeutic efficacy for which in vivo studies need to be carried out. We are currently exploring the pH-dependant properties of the liposomal formulation along with biodistribution studies of drug in male Wistar rats. An improved consideration of the fundamental mechanism of conduct of liposomal formulation in animal systems is indispensable when acclimatizing the liposomal formulation for enhancing carrier efficiency.
Authors’ contributions
C. Hu contributed to conceptualization. C. Hu, X. Wang, and Y. Li contributed to data curation. C. Hu, X. Han, and B. Ren contributed to formal analysis. C. Hu and X. Han contributed to methodology. B. Ren and G. Yin contributed to Software. C. Hu and X. Han contributed to validation. C. Hu, B. Ren, and G. Yin contributed to investigation. C. Hu, X. Wang, and Y. Li contributed to Writing – original draft. C. Hu and X. Wang contributed to Writing – review & editing. All authors have read and approved the final manuscript.
Ethics statement
The institutional small animal ethics committee had approved the study vide ethical approval number 39/CCH/2019.
Acknowledgements
The authors would like to thank Department of Neurosurgery, Cangzhou Central Hospital for supporting this research work.
Disclosure statement
The authors declare that there is no conflict of interest.
Funding
This work did not receive any external funding.
Data availability statement
All data generated or analysed during this study are included in this submitted article. The raw data shall be made available upon request to the corresponding author.
References
- Dai C, Liu X, Ma W, et al. The treatment of refractory pituitary adenomas. Front Endocrinol (Lausanne)). 2019;10:334.
- Lee M, Pellegata NS. Folate receptor-mediated drug targeting: a possible strategy for nonfunctioning pituitary adenomas? Endocrinology. 2013;154(4):1387–1389. Epub 2013/03/26.
- Wang H, Chen K, Yang Z, et al. Diagnosis of invasive nonfunctional pituitary adenomas by serum extracellular vesicles. Anal Chem. 2019;91(15):9580–9589.
- Dai C, Feng M, Liu X, et al. Refractory pituitary adenoma: a novel classification for pituitary tumors. Oncotarget. 2016;7(50):83657–83668.
- Liu X, Ma S, Dai C, et al. Antiproliferative, antiinvasive, and proapoptotic activity of folate receptor α-targeted liposomal doxorubicin in nonfunctional pituitary adenoma cells. Endocrinology. 2013;154(4):1414–1423.
- Chatzellis E, Alexandraki KI, Androulakis II, et al. Aggressive pituitary tumors. Neuroendocrinology. 2015;101(2):87–104. Epub 2015/01/13.
- Colao A, Di Somma C, Pivonello R, et al. Medical therapy for clinically non-functioning pituitary adenomas. Endocr Relat Cancer. 2008;15(4):905–915.
- Greenman Y. Dopaminergic treatment of nonfunctioning pituitary adenomas. Nat Clin Pract Endocrinol Metab. 2007;3(8):554–555.
- Jin S-E, Jin H-E, Hong S-S. Targeted delivery system of nanobiomaterials in anticancer therapy: from cells to clinics. Biomed Res Int. 2014;2014:814208.
- Mirnezami SMS, Heydarinasab A, Akbarzadeh A, et al. Investigation of characterization and cytotoxic effect of PEGylated nanoliposomal containing melphalan on ovarian cancer: an in vitro study. J Exp Nanosci. 2021;16(1):102–116.
- Shi J, Votruba AR, Farokhzad OC, et al. Nanotechnology in drug delivery and tissue engineering: from discovery to applications. Nano Lett. 2010;10(9):3223–3230.
- Zhang L, Gu F, Chan J, et al. Nanoparticles in medicine: therapeutic applications and developments. Clin Pharmacol Ther. 2008;83(5):761–769.
- Lammers T, Hennink W, Storm G. Tumour-targeted nanomedicines: principles and practice. Br J Cancer. 2008;99(3):392–397.
- Lammers T, Kiessling F, Hennink WE, et al. Drug targeting to tumors: principles, pitfalls and (pre-) clinical progress. J Control Release. 2012;161(2):175–187.
- Paulos CM, Reddy JA, Leamon CP, et al. Ligand binding and kinetics of folate receptor recycling in vivo: impact on receptor-mediated drug delivery. Mol Pharmacol. 2004;66(6):1406–1414.
- Bhattacharya S. Methotrexate-loaded polymeric lipid hybrid nanoparticles (PLHNPs): a reliable drug delivery system for the treatment of glioblastoma. J Exp Nanosci. 2021;16(1):345–368.
- Zhao X, Li H, Lee RJ. Targeted drug delivery via folate receptors. Expert Opin Drug Deliv. 2008;5(3):309–319.
- Liu Q, Lee RJ. Folate receptor targeted liposomes. DDRO. 2005;2(7):547–552.
- Low PS, Kularatne SA. Folate-targeted therapeutic and imaging agents for cancer. Curr Opin Chem Biol. 2009;13(3):256–262.
- Didion C, Henne WA. Bibliometric analysis of folate receptor research: a comprehensive bibliometric analysis of the entire field of folate receptor research. 2020.
- Galt JR, Halkar RK, Evans C-O, et al. In vivo assay of folate receptors in nonfunctional pituitary adenomas with 99mTc-folate SPECT/CT. J Nucl Med. 2010;51(11):1716–1723.
- Evans C-O, Reddy P, Brat DJ, et al. Differential expression of folate receptor in pituitary adenomas. Cancer Res. 2003;63(14):4218–4224.
- Guo Y, Sun Z. Investigating folate-conjugated combinatorial drug loaded ZnO nanoparticles for improved efficacy on nasopharyngeal carcinoma cell lines. J Exp Nanosci. 2020;15(1):390–405.
- Moreno CS, Evans C-O, Zhan X, et al. Novel molecular signaling and classification of human clinically nonfunctional pituitary adenomas identified by gene expression profiling and proteomic analyses. Cancer Res. 2005;65(22):10214–10222.
- Evans CO, Yao C, LaBorde D, et al. Folate receptor expression in pituitary adenomas cellular and molecular analysis. Vitam Horm. 2008;79:235–266.
- Yao C, Evans C-O, Stevens VL, et al. Folate receptor alpha regulates cell proliferation in mouse gonadotroph alphaT3-1 cells. Exp Cell Res. 2009;315(18):3125–3132.
- Verma A, Jain A, Tiwari A, et al. Folate conjugated double liposomes bearing prednisolone and methotrexate for targeting rheumatoid arthritis. Pharm Res. 2019;36(8):123.
- Jain A, Jain SK. Application potential of engineered liposomes in tumor targeting. Multifunctional Systems for Combined Delivery, Biosensing and Diagnostics: Elsevier; 2017. p. 171–191.
- Jain A, Gulbake A, Jain A, et al. Dual drug delivery using ‘smart’ liposomes for triggered release of anticancer agents. J Nanopart Res. 2013;15(7):1772.
- Jain A, Jain SK. Multipronged, strategic delivery of paclitaxel-topotecan using engineered liposomes to ovarian cancer. Drug Dev Ind Pharm. 2016;42(1):136–149.
- Jain A, Jain S. Advances in tumor targeted liposomes. Curr Mol Med. 2018;18:44–57.
- Ogue S, Takahashi Y, Onishi H, et al. Preparation of double liposomes and their efficiency as an oral vaccine carrier. Biol Pharm Bull. 2006;29(6):1223–1228.
- Mokale VJ, Patil HI, Patil AP, et al. Formulation and optimisation of famotidine proniosomes: anin vitroandex vivostudy. J Exp Nanosci. 2016;11(2):97–110.
- Jain A, Jain SK. In vitro release kinetics model fitting of liposomes: an insight. Chem Phys Lipids. 2016;201:28–40. Epub 2016/12/17.
- Even-Zohar N, Greenman Y. Management of NFAs: medical treatment. Pituitary. 2018;21(2):168–175.
- Greenman Y, Cooper O, Yaish I, et al. Treatment of clinically nonfunctioning pituitary adenomas with dopamine agonists. Eur J Endocrinol. 2016;175(1):63–72.
- Greenman Y, Tordjman K, Osher E, et al. Postoperative treatment of clinically nonfunctioning pituitary adenomas with dopamine agonists decreases tumour remnant growth. Clin Endocrinol. 2005;63(1):39–44.
- Raverot G, Castinetti F, Jouanneau E, et al. Pituitary carcinomas and aggressive pituitary tumours: merits and pitfalls of temozolomide treatment. Clin Endocrinol (Oxf)). 2012;76(6):769–775.
- Lee RJ, Low PS. Folate-mediated tumor cell targeting of liposome-entrapped doxorubicin in vitro. Biochim Biophys Acta Biomembr. 1995;1233(2):134–144.
- Yamabe K, Kato Y, Onishi H, et al. In vitro characteristics of liposomes and double liposomes prepared using a novel glass beads method. J Control Release. 2003;90(1):71–79.
- Sartori T, Murakami FS, Cruz AP, et al. Development and validation of a fast RP-HPLC method for determination of methotrexate entrapment efficiency in polymeric nanocapsules. J Chromatogr Sci. 2008;46(6):505–509.
- Dangi R, Hurkat P, Jain A, et al. Targeting liver cancer via ASGP receptor using 5-FU-loaded surface-modified PLGA nanoparticles. J Microencapsul. 2014;31(5):479–487.
- Jain D, Bajaj A, Athawale R, et al. Surface-coated PLA nanoparticles loaded with temozolomide for improved brain deposition and potential treatment of gliomas: development, characterization and in vivo studies. Drug Delivery. 2016;23(3):989–1006.
- Chu L, Wang A, Ni L, et al. Nose-to-brain delivery of temozolomide-loaded PLGA nanoparticles functionalized with anti-EPHA3 for glioblastoma targeting. Drug Delivery. 2018;25(1):1634–1641.
- Evans C-O, Young AN, Brown MR, et al. Novel patterns of gene expression in pituitary adenomas identified by complementary deoxyribonucleic acid microarrays and quantitative reverse transcription-polymerase chain reaction. J Clin Endocrinol Metab. 2001;86(7):3097–3107.
- Banerjee S, Sen K, Pal TK, et al. Poly(styrene-co-maleic acid)-based pH-sensitive liposomes mediate cytosolic delivery of drugs for enhanced cancer chemotherapy. Int J Pharm. 2012;436(1-2):786–797. Epub 2012/08/14.
- Mastrobattista E, Storm G, Van Bloois L, et al. Cellular uptake of liposomes targeted to intercellular adhesion molecule-1 (ICAM-1) on bronchial epithelial cells. Biochim Biophys Acta Biomembr. 1999;1419(2):353–363.