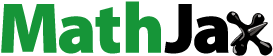
Abstract
Herein, we represent the bio-synthesis of gold nanoparticles (Au NPs) employing Oak gum extract as the green template, an efficient natural and non-toxic reductant and stabilizer based on its phytochemicals. The characterization of as-synthesized Au NPs was performed through Fourier transformed infrared spectroscopy (FT-IR), scanning electron microscopy (SEM), transmission electron microscopy (TEM), energy dispersive X-ray spectroscopy (EDS), elemental mapping, UV-Vis and X-ray diffraction (XRD). TEM analysis explored the average particle size being 10-15 nm. The as-developed material was biologically explored in the inhibition of human colon cancer. Cytotoxicity of assessed over standard colon cancer cell line HT-29 and Caco-2. Cell viability of the two cell lines showed excellent outcomes, as observed by MTT assay. Futhermore, the Au@O. Gum nanocomposite was employed in the determination of the antioxidant properties following DPPH radical scavenging potential in presence of butylated hydroxytoluene (BHT) as the positive control and the result was compared to the raw Oak gum which resulted the scavenging activity as 21.54 and 54.56% respectively. The obtained results evidently validate the Au@O. Gum material as a potent colon cancer protective drug for the treatment of human colon cancer.
1. Introduction
Among the different human gastrointestinal disorders, colorectal cancer (CRC) is the most deadly, invasive and metastatic disease [Citation1,Citation2]. In the western developed countries CRC is one of the leading reason for morbidity and mortality [Citation3]. Different routine factors, ageing, bacterial infections along with some genetic disorders are the general factors behind this disease [Citation4,Citation5]. CRC usually affects the bowel lining initially and then gradually spreads in the wall and surrounding muscle layers [Citation6]. The symptoms frequently observed in CRC are constipation, irregular bowel movement, blood coming out of rectum, weakness and unusual loss in weight. Although the survival rate is very low, it is curable if detected in early stages. Among the different treatment procedures surgical operation, chemotherapy, radio therapy and targeted therapy are the most common [Citation7,Citation8]. However, in spite of following up with these treatment protocols, patients endure invasion with undesirable entailments like swelling of the operated area, weakness of the nerves, hair loss, fatigue, stomach upset etc. Scientific survey have shown that the occurrence and mortality rate due to CRC will be augmented by 60% all over the world within 2030 [Citation9,Citation10]. Consequently, a new formulation method is of high demand and scientists are continuously behind the improved methodology in order to improve their specificity and efficacy [Citation11].
Nanoscience and technology has come up with tremendous prospects in the domain of diagnostics and medicinal therapeutics following a précised manner since last 30 years and more [Citation12]. In recent times it has come into prominence as one of the most ubiquitous segment of medical science which finds vital role in biological screening, explicit detection and regioselective treatment of different ailments with high specificity and enhanced success rate [Citation13]. There are several reports where different kind of bare or modified biofunctionalized nanomaterials have been used in medicinal therapeutics, like targeted drug delivery, tissue engineering, photoablation therapy, bioimaging, hyperthermia, antimicrobial activities etc, in addition to the treatment in different cancers [Citation14–26]. Different nano-formulations like quantum dots, modified magnetic NPs, silica NPs, Ag NPs, Au NPs, liposomes, dendrimers, nano-emulsions etc have been reported as either drug delivery agent or itself as chemotherapeutic nanomedicines, derived from their unique pharmacokinetics, high surface area, greater drug loading capacity, low dose application, stabilizing the drug under cell environment and biocompatibility [Citation27–32]. The as used nano-drug is distinctively architectured by surface engineering over a central NP with phytochemicals, bio-macromolecules, organic ligands or another set of NPs, following simple covalent or coordinate bonding [Citation33–37]. Some recent reports indicate the outstanding anti-cancer potential of bio-engineered metal NPs without any undesirable side effects [Citation38–41].
With these inputs we have been encouraged to develop an Oak gum stabilized Au NPs (Au@O. Gum). The oxygenated phytochemicals contained therein also help to anchor the Au ions followed by their biogenic reduction into NPs. The as synthesized Au@O. Gum has been explored in the CRC in vitro studies over two HT-29 and Caco-2 cell lines. Cytotoxicity of the material was studied following standard MTT assays and the result was compared to normal human cell lines. As the anticancer and antioxidant activity are correlated in terms of invasion mechanism, we also carried out the DPPH radical scavenging assay.
2. Experimental
2.1. Materials and methods
The Oak fruits were purchased from local market and the gum was extracted from acorns. HAuCl4, H2O2, DPPH and all the solvents were purchased from Sigma-Aldrich and used as such. UV-Vis analyses were carried out over a Cary 100 spectrophotometer (Agilent). FT-IR were recorded over a Bruker VERTEX 80v spectrophotometer. A FESEM-TESCAN MIRA3 microscope was used for SEM analysis along with EDX detector. TEM analysis was done using a Philips CM10 microscope (200 Kv). XRD investigation was done using Co Kα radiation (λ = 1.78897 Å, 40 keV, 40 mA) in the diffraction angle range of 2θ = 5 to 80°.
2.2. Green synthesis of Au NPs mediated by oak gum
As a precursor of Au, HAuCl4 (50 mL, 1 mM) was added dropwise into an aqueous solution of Oak Gum (10 mL, 1%) and stirred under ambient conditions. Instantly, the reduction of HAuCl4 started, as indicated by the change in solution color from light yellow to wine red [Citation37]. After 30 min of reaction, the Au@O. Gum nanocomposite was recovered by centrifuge and the residue was thoroughly washed with deionised water and dried at 60 °C.
2.3. Antioxidant analysis
An equal volume of DPPH free radical solution (0.04 mg/mL, 150 µL) was mixed to 5 different concentrations (32.25, 62.5, 125, 250, 500 and 1000 µg/mL) of the nanocomposite and subsequently incubated for 30 min. Absorbances of the corresponding mixtures were measured at 517 nm and the following equation was used to calculate the radical scavenging activity in terms of % inhibition [Citation37].
Equation 1
Equation 1
Abs sample: absorbance of the reaction mixture, Abs blank: absorbance of the blank for each sample dilution in DPPH solvent, Abs control: absorbance of DPPH solution in sample solvent.
2.4. MTT assay
The cell lines were initially cultured in 96-well plates at 37 °C in 5% CO2 atmosphere for 24 h. The growth media (10% FBS) was then decanted off from the plate and were washed twice with PBS. The compounds were then introduced in different concentrations (0.5, 5, 50, 500, and 1000 µg/mL) in RPMI medium and the system was incubated again for 3 days. The MTT dye in PBS solution (10 μL, 5 mg/mL) was next added to each well and incubated again for 4 h. In the same way media was removed and replaced with 100 µL DMSO in each well. In order to assist the formazan crystal solubilization all the plates were gently swirled. Finally, absorbance of the resulting mixture was measured at 545 nm and % cell toxicity alongwith IC50 were determined as the following formula [Citation37].
3. Results and discussion
3.1. Characterization of the nanocomposite
The Au@O. Gum nanocomposite was prepared following an in-situ sustainable synthetic modification strategy. Au ions were green reduced over an aqueous of Oak Gum fruits where the corresponding oxygenated phytochemicals takes part in the toxic chemicals free reduction to Au NPs. The biomolecules further covers up the tiny NPs by electrochemical attachment, thereby stabilizing them by offering capping effect. The material was subsequently characterized using several advanced physicochemical methods like UV-Vis, FT-IR spectroscopy, FE-SEM, TEM, EDX, elemental mapping and XRD.
The formation of Au NP can be detected merely by visible observations, as indicated in , where the pale yellow colored HAuCl4 solution gradually intensifies to deep purple color. This however, also quantitatively be measured by UV-Vis spectrochemical study, where the Au NPs show a typical Surface Plasmon Resonance (SPR) peak at around 545 nm, derived from the bathochromic shift due to reduction of Au3+ to Au0 species [Citation37]. depicts the reaction sequence for this reduction in terms of measured absorbances in UV-Vis, at different time intervals. As the reaction progresses, a distinct absorption peak evolves out and it completes in 1 h of reaction time. In order to compare, absorption of the fruit extract was also measured which however did not exhibit absorption in the SPR region. This evidently differentiates the O. Gum extract and Au@O. Gum nanocomposite.
FT-IR analysis was done to detect the functions over Au@O. Gum nanocomposite and also to validate the successful O. Gum phytochemicals functionalization over the NPs. The output has been displayed in . The spectrum of the fruit extract displays some characteristic peaks at 3414, 2920, 1684, 1620, 1437 and 1056 cm−1, ascribed to O-H stretching (phenolic compounds, flavonoids), C-H stretching (alkyl groups), C = O stretching (carbonyl), C = C stretching (olefins), C-H bending (alkyl groups) and C-O stretching (alcoholic moiety) respectively arising out of the phytochemicals therein (). represents the spectrum of Au@O. Gum nanocomposite, which bears all the previous vibrations of plant extract, suggesting the fruitful modification of Oak phytochemicals over Au NPs.
Figure 2. Oak gum solution (a), HAuCl4×H2O solution and color changes of synthesis gold nanoparticles (c,d,e and f).
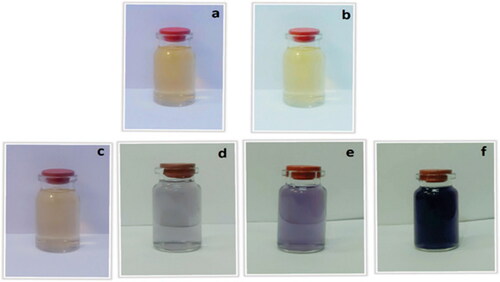
Figure 3. UV-Vis absorption spectra of Oak gum solution and different solution containing Au NPs by the times.
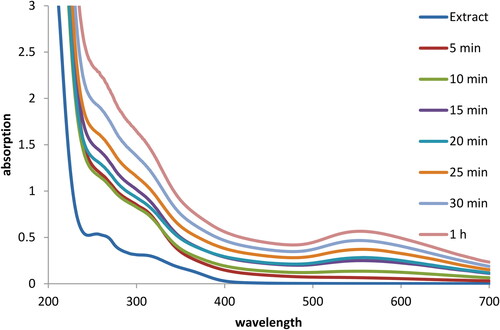
Morphological structure and apparant size of the Au@O. Gum nanocomposite were explored by TEM analysis. As can be seen from , the materials are spherical shaped and well-dispersed. Most of the particles can de distinguished individually and there is hardly any sense of aggregation between the tiny Au NPs. Mean diameter of the nanocomposite particles are within 10-15 nm. This kind of uniform distribution and regular array of particle size is markedly observed due to bio-inspired synthesis.
Chemical composition of the as-synthesized material was established by EDX analysis (). The outcome depicts a strong and discrete signal at 2.2 keV, characteristic of Au species. Another signal found at 9.8 keV arises out of the gold coating over the sample, prior to instrumental analysis. The small peaks of C, N and O are detected in the lower energy region, attributed to the O. Gum phytochemicals.
In addition to the EDX analysis for chemical identity, the constitutional study was further extended in elemental mapping, associated with the SEM analysis. represents the SEM image upon which X-ray scanning generates the elemental distribution over the surface. As can be seen from the image, C and Au species are homogeneously distributed over the matrix.
Finally, crystallinity and phase structure of Au@O. Gum nanocomposite was determined from XRD study. The pattern demonstrates a single phase and moderately crystalline material. The sharp diffraction peaks being distinguished at 2θ = 38.5°, 44.1°, 64.3° and 77.5° are attributed to the Au crystalline (1 1 1), (2 0 0), (2 2 0) and (3 1 1) phases [Citation37] ().
3.2. Study of antioxidant potential of Au@O. Gum nanocomposite
Herein, we investigated the antioxidant efficiency of bare Oak gum and its Au nanocomposite in terms of DPPH radical scavenging activity. The Au@O. Gum nanocomposite was taken in several concentrations, viz., 31.25, 62.5, 125, 250, 500 and 1000 μg/mL. In the study, 1:1 MeOH and BHT were measured as negative and positive controls. UV absorption (A) of the nanocomposite-DPPH complex were measured at 517 nm and based on those results the antioxidant property were calculated (Equation 1). Evidently, the activity got enhanced dose dependently, being proportional to the sample concentration (). The corresponding activities were found 54.56% and 21.54% respectively in favor of the bare gum and Au@O. Gum respectively at the 1000 μg/mL concentration [Citation42,Citation43].
3.3. Cytotoxicity studies over Au@O. Gum nanocomposite
Conventional MTT method was used to evaluate the cytotoxicity of bare Oak Gum and biosynthesized Au@O. Gum nanocomposite in variable concentrations over HT-29 and Caco-2 CRC cell lines and the results were compared with normal cell lines (CHO). represents the corresponding outcomes. Evidently, the Au@O. Gum nanocomposite demonstrates significant cytotoxicity, which increased proportionally with concentration, over both the cancer cell lines. The corresponding IC50 values were estimated as 2.7 and 289.6 µg/mL respectively (). The absorbance rate was evaluated at 545 nm, which represented toxicity on normal cell line (CHO) even up to 2000 μg/mL for Au@O. Gum NPs ( and ).
Figure 10. In vitro toxicity analysis of Oak Gum (A) and biosynthesized Au@O. Gum NPs (B) on HT-29 cell.
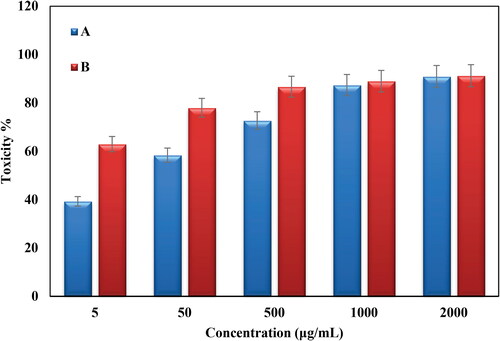
Figure 11. In vitro toxicity analysis of Oak Gum (A) and biosynthesized Au@O. Gum NPs (B) on Coca-2 cell.
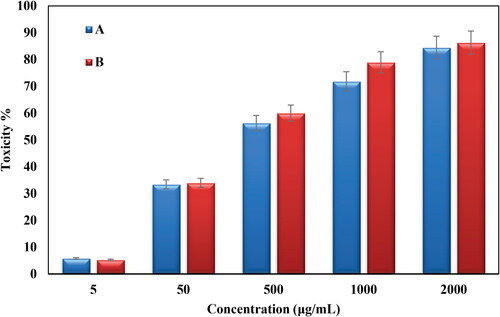
Figure 12. In vitro toxicity analysis of Oak Gum (A) and biosynthesized Au@O. Gum NPs (B) on CHO normal cell.
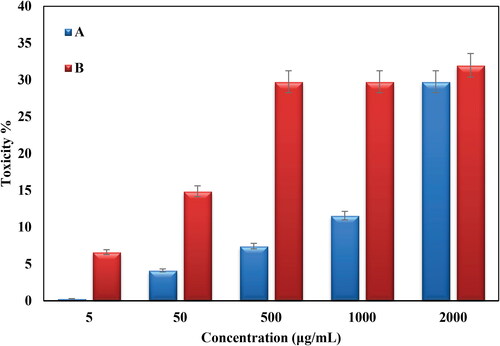
Table 1. The IC50 of Oak Gum and biosynthesized Au@O. Gum NPs in the anti-human colon cancer test.
Regarding the cytotoxicity mechanism of the nanocomposite over malignant cells, it is believed that Au NPs cause the cell death through apoptosis. The Au NPs or Au atoms specifically interacts with the functional groups of abnormally signaling intracellular proteins including the nucleosides and nucleotides of the corresponding DNA molecules through physicochemical interactions followed by restraining their metastatic activities. This results the fragmentation or breakdown of DNA double stranded ladders of the angiogenic cells causing apoptosis without affecting the normal cells. The NPs also effectively block the tyrosine kinase inhibitors and cytokine-based therapies as well as DNA- or protein based vaccines against specific tumor markers, thus exhibiting specific anticancer potential.
4. Conclusion
To end with, we have demonstrated herein a sustainable process for the preparation of Oak gum modified Au NPs as a novel nanocomposite material. The oxygen rich polar Oak gum phytochemicals facilitate the green reduction of incoming Au3+ ions into Au NPs. Biomolecular fabrication over the Au NPs enhances its stabilization from agglomeration by capping. Structural characterizations of Au@O. Gum material was ascertained through several analytical techniques. UV-Vis spectroscopy distinctly identifies the Au NP SPR signal at around 550 nm where TEM analysis displays the tiny Au NPs (10-15 nm) without noticeable agglomerations. EDX and elemental mapping analysis confirmed the constituent elements and their dispersion over the surface. The material is found to be highly crystalline as indicated by the sharp and distinct signals of Au NPs. Towards its application, the material was explored biologically in anti colorectal cancer assays using HT-29 and Caco-2 cell lines. The cytotoxicity studies were carried out following standard MTT assay. Cell viability of the CRC cell lines reduced dose-dependently over the nanocomposite. The corresponding IC50 values were obtained as 2.7 and 289.6 µg/mL respectively. However, the material was found benign over the normal cell lines. In addition, the Au@O. Gum material exhibited excellent antioxidant effects as observed in DPPH radical scavenging investigations. The corresponding quenched colored radical was studied over UV-Vis spectroscopy. The promising outcomes revealed by the novel nanocomposite validate it as a potent formulation drug in CRC management.
Disclosure statement
No potential conflict of interest was reported by the authors.
References
- Dong J, Li J, Luo J, et al. CircHMGCS1 is upregulated in colorectal cancer and promotes proliferation of colorectal cancer cells by targeting microRNA-503-5p. Eur J Inflamm. 2019;17:1–11.
- Lin A, Zhang J, Luo P. Crosstalk between the MSI status and tumor microenvironment in colorectal cancer. Front Immunol. 2020;11:2039.
- Arvelo F, Sojo F, Cotte C. Biology of colorectal cancer. Ecancermedicalscience. 2015;9:520.
- Gulbake A, Jain A, Jain A, et al. Insight to drug delivery aspects for colorectal cancer. World J Gastroenterol. 2016;22(2):582–599.
- Saus E, Iraola-Guzmán S, Willis JR, et al. Microbiome and colorectal cancer: Roles in carcinogenesis and clinical potential. Mol Aspects Med. 2019;69:93–106.
- Datta K, Suman S, Kumar S, et al. Colorectal carcinogenesis, radiation quality, and the ubiquitin-proteasome pathway. J Cancer. 2016;7(2):174–183.
- Han YD, Oh TJ, Chung TH, et al. Early detection of colorectal cancer based on presence of methylated syndecan-2 (SDC2) in stool DNA. Clin Epigenetics. 2019;11(1):51–61.
- Valeri N. Streamlining detection of fusion genes in colorectal cancer: Having “ "Faith" in Precision Oncology in the (Tissue) "Agnostic" Era. Cancer Res. 2019;79(6):1041–1043.
- Brand M, Gaylard P, Ramos J. Colorectal cancer in South Africa: an assessment of disease presentation, treatment pathways and 5-year survival. S Afr Med J. 2018;108(2):118–122.
- Huyghe N, Baldin P, Van den Eynde M. Immunotherapy with immune checkpoint inhibitors in colorectal cancer: what is the future beyond deficient mismatch-repair tumours? Gastroenterol Rep (Oxf)). 2020;8(1):11–24.
- Bray F, Ferlay J, Soerjomataram IRL, et al. Global cancer statistics 2018: GLOBOCAN estimates of incidence and mortality worldwide for 36 cancers in 185 countries. CA: Cancer J Clin. 2019;68:394–424.
- Fortina P, Kricka LJ, Graves DJ, et al. Applications of nanoparticles to diagnostics and therapeutics in colorectal cancer. Trends Biotechnol. 2007;25(4):145–155.
- Upendra L, Minakshi P, Brar B, et al. Nanodiagnostics: a new frontier for veterinary and medical sciences. J Exp Biol Agric Sci. 2016;4:307–318.
- Baig B, Halim SA, Farrukh A, et al. Current status of nanomaterial-based treatment for hepatocellular carcinoma. Biomed Pharmacother. 2019;116:108852.
- Xia Y, You P, Xu F, et al. Novel functionalized selenium nanoparticles for enhanced anti-Hepatocarcinoma activity in vitro. Nanoscale Res Lett. 2015;10:349–362.
- Kanai M, Imaizumi A, Otsuka Y, et al. Dose-escalation and pharmacokinetic study of nanoparticle curcumin, a potential anticancer agent with improved bioavailability, in healthy human volunteers. Cancer Chemother Pharmacol. 2012;69(1):65–70.
- Awual MR, Khraisheh M, Alharthi NH, et al. Efficient detection and adsorption of cadmium(II) ions using innovative nano-composite materials. Chem Eng J. 2018;343:118–127.
- Mohammadi P, Heravi MM, Sadjadi S. Green synthesis of Ag NPs on magnetic polyallylamine decorated g-C3N4 by heracleum persicum extract: efficient catalyst for reduction of dyes. Sci Rep. 2020;10(1):6579–6588.
- Ding Y, Shen SZ, Sun H, et al. Design and construction of polymerized-chitosan coated Fe3O4 magnetic nanoparticles and its application for hydrophobic drug delivery. Mater Sci Eng C. 2015;48:487–498.
- Esmaeilpour M, Sardarian AR, Firouzabadi H. N-heterocyclic carbene-Pd(II) complex based on theophylline supported on Fe3O4@SiO2 nanoparticles: highly active, durable and magnetically separable catalyst for green Suzuki-Miyaura and Sonogashira-Hagihara coupling reactions. J Organometal Chem. 2018;873:22–34.
- Zhang H, Li T, Luo W, et al. Green synthesis of Ag nanoparticles from leucus aspera and its application in anticancer activity against alveolar cancer. J Exp Nanosci. 2022;17(1):47–60.
- Huang Y, Zhu C, Xie R, et al. Green synthesis of nickel nanoparticles using fumaria officinalis as a novel chemotherapeutic drug for the treatment of ovarian cancer. J Exp Nanosci. 2021;16(1):368–381.
- Liu H, Wang G, Liu J, et al. Green synthesis of copper nanoparticles using cinnamomum zelanicum extract and its applications as a highly efficient antioxidant and anti-human lung carcinoma. J Exp Nanosci. 2021;16(1):410–423.
- Li T, Yan G, Bai Y, et al. Papain bioinspired gold nanoparticles augmented the anticancer potency of 5-FU against lung cancer. J Exp Nanosci. 2020;15(1):109–128.
- Rugaie OA, Jabir M, Kadhim R, et al. Gold nanoparticles and graphene oxide flakes synergistic partaking in cytosolic bactericidal augmentation: role of ROS and NOX2 activity. Microorganisms. 2021;9(1):101–114.
- Al-Omar MS, Jabir M, Karsh E, et al. Gold nanoparticles and graphene oxide flakes enhance cancer cells’ phagocytosis through granzyme-perforin-dependent biomechanism. Nanomater. 2021;11(6):1382–1395.
- Win KY, Ye E, Teng CP, et al. Engineering polymeric microparticles as theranostic carriers for selective delivery and cancer therapy. Adv Healthcare Mater. 2013;2(12):1571–1575.
- Aravind A, Jeyamohan P, Nair R, et al. AS1411 aptamer tagged PLGA-lecithin-PEG nanoparticles for tumor cell targeting and drug delivery. Biotechnol Bioeng. 2012;109(11):2920–2931.
- Zhang Q, Liu F, Nguyen KT, et al. Multifunctional mesoporous silica nanoparticles for cancer-targeted and controlled drug delivery. Adv Funct Mater. 2012;22(24):5144–5156.
- Tao Y, Han J, Ye C, et al. Reduction-responsive gold-nanoparticle-conjugated pluronic micelles: an effective anti-cancer drug delivery system. J Mater Chem. 2012;22(36):18864–18671.
- Bose S, Panda AK, Mukherjee S, et al. Curcumin and tumor immune-editing: resurrecting the immune system. Cell Div. 2015;10(1):6–18.
- Yallapu MM, Nagesh PKB, Jaggi M, et al. Therapeutic applications of curcumin nanoformulations. Aaps J. 2015;17(6):1341–1356.
- Ghosh D, Choudhury ST, Ghosh S, et al. Nanocapsulated curcumin: oral chemopreventive formulation against diethylnitrosamine induced hepatocellular carcinoma in rat. Chem Biol Interact. 2012;195(3):206–214.,
- Xue W, Yang G, Karmakar B, et al. Sustainable synthesis of Cu NPs decorated on pectin modified Fe3O4 nanocomposite: catalytic synthesis of 1-substituted-1H-tetrazoles and in-vitro studies on its cytotoxicity and anti-colorectal adenocarcinoma effects on HT-29 cell lines. Arab J Chem. 2021;14(9):103306–103316.
- Huang Y, Kang Y, El-Kott A, et al. Decorated Cu NPs on lignin coated magnetic nanoparticles: its performance in the reduction of nitroarenes and investigation of its anticancer activity in A549 lung cancer cells. Arab J Chem. 2021;14(8):103299–103308.
- Sun W, Karmakar B, Ibrahium HA, et al. Design and synthesis of nano Cu/chitosan-starch bio-composite for the treatment of human thyroid carcinoma. Arab J Chem. 2022;15(1):103465–103475.
- Zhao P, El-Kott A, Ahmed AE, et al. Green synthesis of gold nanoparticles (Au NPs) using tribulus terrestris extract: investigation of its catalytic activity in the oxidation of sulfides to sulfoxides and study of its anti-acute leukemia activity. Inorg Chem Commun. 2021;131:108781–108791.
- Abdel-Fattah WI, Ali GW. On the anti-Cancer activities of silver nanoparticles. J Appl Biotechnol Bioeng. 2018;5(2):43–46.
- Patil MP, Kim GD. Eco-friendly approach for nanoparticles synthesis and mechanism behind antibacterial activity of silver and anticancer activity of gold nanoparticles. Appl Microbiol Biotechnol. 2017;101(1):79–92.
- Bisht G, Rayamajhi S. ZnO nanoparticles: a promising anticancer agent. Nanobiomedicine (Rij). 2016;3:9.
- Hassanien R, Husein DZ, Al-Hakkani MF. Biosynthesis of copper nanoparticles using aqueous tilia extract: antimicrobial and anticancer activities. Heliyon. 2018;4(12):e01077.
- Nakkala JR, Mata R, Sadras SR. The antioxidant and catalytic activities of green synthesized gold nanoparticles from piper longum fruit extract. Process Saf Environ Prot. 2016;100:288–294.
- Abel EE, Poonga PRJ, Panicker SG. Characterization and in vitro studies on anticancer, antioxidant activity against Colon cancer cell line of gold nanoparticles capped with cassia tora SM leaf extract. Appl Nanosci. 2016;6(1):121–129.