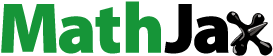
Abstract
In this work, we have described the biogenic synthesized gold nanoparticles being supported over Kaolin mediated by Pomegranate peel extract as natural reducing and stabilizing agent under mild conditions without any toxic reagents. Pomegranate peel extract was used as a green reducing agent and an excellent stabilizer of the synthesized Au NPs. Physicochemical characterization of the as-synthesized Kaolin@extract/Au NPs nanocomposite was carried out through electron microscopy (SEM and TEM), energy dispersive X-ray spectroscopy (EDX), elemental mapping, X-ray diffraction (XRD) and inductively coupled plasma-optical emission spectroscopy (ICP-OES). In the cellular and molecular part of the recent study, the treated cells with Au NPs-Kaolin nanocomposite were assessed by MTT assay for 48 h about the cytotoxicity and anti-human oral squamous cell carcinoma properties on normal (HUVEC) and oral squamous cell carcinoma cell lines i.e. HSC-2, HSC-3, HSC-4 and Ca9-22. It has been observed that the Au NPs with spherical shape and size were dispersed homogeneously on the modified-Kaolin surface. The significance of this study is the novel initiative to combine the gold nanoparticle with inexpensive Kaolin clay and utilization of them as anticancer agent. The IC50 of Kaolin@extract/Au nanocomposite were 277, 367, 250 and 545 µg/mL against HSC-2, HSC-3, HSC-4 and Ca9–22 cell lines, respectively. The viability of malignant human oral cell lines reduced dose-dependently in the presence of Kaolin@extract/Au NPs nanocomposite.
1. Introduction
Cancer is a condition where cells in a specific part of the body grow and reproduce uncontrollably. The cancerous cells can invade and destroy surrounding healthy tissue, including organs. Cancer sometimes begins in one part of the body before spreading to other areas. This process is known as metastasis [Citation1–4]. Unfortunately, therapeutic agents (chemotherapy, biology and nanotechnology) are so selective and effective in targeting in vitro cancer cells, and even in proportionate animal specimens [Citation3–6]. However, failing in clinical trials is not a rule but a rule. This is because the biological distribution of therapeutic agents can be a fundamental factor in these fractures. Inadequate concentration at unwanted concentration and target sites elsewhere, leading to dose-limiting poisoning [Citation5–7]. The biological distribution of drug agents is controlled largely by the drugs ability to penetrate biological barriers. Strategy for Adding Targeting Sections to Therapeutic Nanoparticles to Improve Location Specification to date, despite 30 years of effort in pharmaceutical companies and many laboratories, it has not yet been able to produce clinically approved drugs [Citation8–10]. This failure is because the addition of molecular agents increases the targeting of cognitive characteristics. However, it does so in the face of much greater difficulty in managing biological barriers [Citation7–10].
Nanoscience and nanotechnology are the study and application of extremely small things and can be used across all the other science fields, such as chemistry, biology, physics, materials science and engineering [Citation8–10]. Nanotechnology is defined in different ways in several countries, which affects the nanodrugs clinical validation. However, what these different definitions have in common is the use of nanoscale structures. There are several distinct benefits to using nanotechnology in the diseases treatment [Citation9–12].
A nanoparticle or ultrafine particle is usually defined as a particle of matter that is between 1 and 100 nanometres (nm) in diameter. The term is sometimes used for larger particles, up to 500 nm, or fibres and tubes that are less than 100 nm in only two directions [Citation8–11]. Metal nanoparticles and metal oxides; have been widely used by medical consumers and manufacturers. The mechanism of nanoparticle-induced toxicity against cancer cells is the production of reactive oxygen species (ROS). Excessive production of reactive oxygen species can lead to oxidative stress, disruption of normal physiological maintenance, and oxidation regulation. These effects in turn lead to DNA damage, unregulated cell signalling pathways, changes in cell evolution, cytotoxicity, apoptotic death, and the onset of cell death [Citation10–13].
Metal nanoparticles and oxides of metal nanoparticles due to their optical properties due to the large active area and high atomic number, amplify the photoelectric and Compton effects of both X-ray and gamma-ray interactions with the adsorbent in the diagnostic and therapeutic range [Citation8–13]. Finally, they can lead to the development of methods for the destruction of tumour cells and reduce their survival with minimal side effects in radiation therapy [Citation9–13].
As a result, increasing industrial knowledge in the field of scalable nanoparticle synthesis, along with the design of multifunctional nanoparticles, will dramatically change the strategies of microenvironmental preparation and therapeutic-diagnostic nanoparticles for cancer treatment [Citation7–11].
Kaolinite has the interesting composite AlSiO(OH)4 that used as a valuable support material in nanotechnology field based on its low cost, stability and sustainable ground, being comparable to carbonaceous materials such as GO and CNTs, except the advantage of high surface area in latter [Citation14–20]. In the recent research, we prepared and investigated the Kaolin@extract/Au NPs in the cytotoxicity and anti-human oral squamous cell carcinoma studies against common human oral squamous cell carcinoma cell lines i.e. HSC-2, HSC-3, HSC-4 and Ca9-22.
2. Experimental
2.1. Preparation of Kaolin@extract/Au NPs using Pomegranate peel extract
The plant extract was prepared by solving 0.1 g of the powdered Pomegranate peel extract species into 10 mL of deionized water. It was filtered through Whatman-1 filter paper to remove the unwanted matters and the filtrate was kept for next step.
Kaolin powder (0.5 g) was dispersed in DI water (100 mL) by sonication for 30 min. Subsequently, 10 mL of Pomegranate peel extract was added to the suspension and stirred for 3 h. This makes the plant biomolecules to bind on the surface of Kaolin. Then, 5 mL of 0.1 M HAuCl4 solution was added to it slowly and the mixture was agitated at 100 °C for 2 h. Finally, the Kaolin@extract/Au NPs was collected by centrifugation, washed thoroughly with DI water and dried in air. Au content in the as synthesized material was analysed by ICP-AES technique, to be 0.076 mmol/g.
2.2. Anti-human oral squamous cell carcinoma capacities of Kaolin@extract/Au nanocomposite
The extent of genetic and epigenetic diversity between and within patient tumours is being mapped in ever more detail. It is clear that cancer is an evolutionary process in which tumour cell intrinsic and extrinsic forces shape clonal selection. The pre-clinical oncology pipeline uses model systems of human cancer – including mouse models, cell lines, patient-derived organoids and patient-derived xenografts – to study tumour biology and assess the efficacy of putative therapeutic agents. Model systems cannot completely replicate the environment of human tumours and, even within the same cancer model; data are often irreproducible between laboratories. One hypothesis is that ongoing evolutionary processes remain relevant in laboratory models, leading to divergence over time. In a recent edition of Nature, Ben-David and colleagues showed that different stocks of widely used cancer cell lines – a staple of cancer research over many decades – are highly heterogeneous in terms of their genetics, transcriptomics and responses to therapies. The authors find compelling evidence of positive selection based on ongoing mutational processes and chromosomal instability. Thus, the origin, culture conditions and cumulative number of population doublings of cell lines likely influence experimental outcomes [Citation21].
In this study, HSC-2, HSC-3, HSC-4 and Ca9-22 cells were used to evaluate the anticancer effect of Kaolin@extract/Au nanocomposite on cell culture.
In the recent study, these cells in DMEM culture medium (Gibco, USA) with 10% FBS (Gibco, USA) and penicillin/streptomycin (100 μL/100 μg/mL) in an incubator containing 5% Carbon dioxide with 90% humidity was stored at 37 °C. Then, when about 80% of the flask was filled, cell passage was performed and about 5 × 104 cells (per square centimetre) were placed in 24 house bacterial petri dishes in the usual environment. The cells were treated with different concentrations of nanoparticles 24 h later and kept in this condition for 3 days. The survival rate of cultured cells was prepared with different concentrations. In this experiment, cells were cultured at 3 × 104 cells/well in 24-well plates and kept in an incubator at 37 °C for 24 h. Then the old culture medium was taken out of the wells and the cells were treated with different concentrations of nanoxidro. This test was performed on the first, second and third days after exposing the cells to the compounds; thus, at the appropriate time after culturing the cells in plates of 24 cells, the culture medium was removed and about 300 μL of fresh medium containing 30 μL of MTT solution was added to each cell. After 3–4 h of incubation at 37 °C, MTT solution is removed and 200 μL (Dimethyl Sulfoxide, Merck, USA, 100%) DMSO is added to each house. Then the sample absorption was read at 570 wavelengths using ELISA rider (Expert 96, Asys Hitch, Ec Austria). This experiment was repeated 3 times and each time, four wells were considered for each nano oxide concentration. Cell survival percentage was evaluated by the following formula [Citation21]:
2.3. Qualitative measurement
The results were evaluated as mean ± SE using SPSS software version 12 and statistical tests of variance of completely randomized block design. Drawing graphs in Excel software was performed and the significance level of the differences was considered p < 0.05.
3. Results and discussion
Regarding the cytotoxicity of metal nanoparticles, several studies have been performed and it has been determined that the toxicity of metal nanoparticles is more than the non-nanoparticle state or mass of metal materials [Citation22–24]. However, the effects of nanoparticles on human health or the environment in general are still debated and need further research and study. Studies have also shown that metal nanoparticles can be effective at the cellular, microcellular, and molecular levels, such as proteins and genes [Citation25–27]. Evaluation of metal nanoparticles on cellular and animal models indicates that these particles cause cytotoxicity by inducing the production of passive oxygen species. In this regard, a decrease for glutathione, an increase for oxygen and an increase in lipid peroxidation have been reported in samples treated with metal nanoparticles [Citation26–30]. According to previous research, metal nanoparticles have a toxic activity on cancerous cells, which may be because of the relatively tumour cells acidic environment in which the release of metal ions from metal nanoparticles occurs more rapidly [Citation30–33]. In addition, the metal toxic effects on cancer cells could be because of the interaction of metal atoms with functional groups of proteins, as well as phosphate and nitrogen bases of the DNA molecule. Another effect of metal nanoparticles on cancer cells is growth inhibition by inhibiting cell division, which results in their interaction with the structure and organization of the actin cytoskeleton. Evidence and results of several studies indicate the induction of apoptosis because of the use of metal nanoparticles in cancer cells [Citation31–34]. This seems to happen through the production of reactive oxygen; thus, the active surfaces of metal nanoparticles yield free radicals by releasing metal ions, which these free radicals destroy DNA, especially the mitochondrial membrane, causing the release of cytochrome c and the induction of apoptosis from the mitochondrial pathway [Citation32–35].
The Kaolin@extract/Au nanocomposite has been synthesized by post-synthetic modification approach where Kaolin was initially modified using Pomegranate peel extract and continued by in situ supporting of gold nanoparticles. In order to characterization of the material was scrutinized over diverse analytical techniques, viz., TEM, FE-SEM, EDX, elemental mapping, XRD and ICP-OES.
More detailed intrinsic structure and detailed morphology of Kaolin@extract/Au nanocomposite was studied by TEM analysis. As presented, the modified Kaolin (Kaolin@extract) with flake like shape where the superposed layers are clearly seen. On the other hand, the images of Kaolin@extract/Au display black dots as the Au NPs being deposited over the modified Kaolin (). Size of spherical Au NPs are around 10-15 nm.
depicts the morphology, shape, layered structure and the elemental mapping of Kaolin@extract/Au nanocomposite, being ascertained over FE-SEM. The flaky nanomaterial reveals a continuously layered and rough surface which is a clear indication of phytochemical functionalization. However, Au NPs are not clear visible in the FESEM image, might be due to well-dispersion over the surface. Also, the elemental mapping evidently supports the EDX data and reveals the homogeneous dispersion of the Si, Al, C, O and Au species elemental species over the surface.
The chemical constitution of Kaolin@extract/Au nanocomposite was confirmed by EDX analysis. demonstrated the corresponding profile which indicates the occurrence of Si, Al, and Au as metallic as well as C and O as non-metallic counterpart. Evidently, Si and Al signify the attachment of Kaolin matrix whereas the non-metals indicate the biomolecular involvement.
Phase structure and crystallinity of Kaolin@extract/Au nanocomposite is checked by XRD study (). The major peak obtained at 2θ = 26.3° represents the quartz structure of Kaolin. Two more diffraction peaks observed at 2θ = 45.8° and 21.2° are also assigned to Kaolin structure [Citation18]. Also, the peaks obtained at 2θ = 38.4°, 42.6°, 65.2°, and 77.9° correspond to the (111), (200), (220), and (311) planes of fcc structure of Au crystal (JCPDS No. 04-0783) [Citation20].
In this experiment, the treated cells with different concentrations of the present Kaolin@extract/Au nanocomposite were assessed by MTT assay for 48 h about the cytotoxicity properties on normal (HUVEC) and human oral malignancy cell lines i.e. HSC-2, HSC-3, HSC-4, and Ca9-22.
Cancer is an abnormal growth of tissue or cells exhibiting uncontrolled division autonomously resulting in a progressive increase in the number of cell divisions [Citation36–39]. It causes significant morbidity and mortality and is a major health problem worldwide [Citation38–40] and there are increasing demands for anticancer therapy [Citation40–43]. The fight against cancer is difficult particularly in the development of therapies for severely multiplying tumours. Chemotherapy is available for treatment of cancer but still it exhibits low specificity and is restricted by dose limiting toxicity. It is a challenge to find the therapy and drugs for the treatments of various types of cancer [Citation42–45]. Therefore, conventional methods require the combination of controlled released technology and targeted drug delivery, which is more effective and less harmful. Nanomaterials are expected hopefully to revolutionize cancer diagnosis and therapy [Citation44]. Recently the nanoparticles used in anticancer therapy for several types of cancer are Hep2 cell line, HT-29 cell lines, Vero cell line and breast cancer line MCF-7 [Citation45–50].
The absorbance rate was evaluated at 570 nm, which represented viability on normal cell line (HUVEC) even up to 1000 μg/mL for Kaolin@extract/Au nanocomposite ( and ).
Figure 6. The cytotoxicity effects of HAuCl4 and Kaolin@extract/Au nanocomposite against normal (HUVEC) cell line.
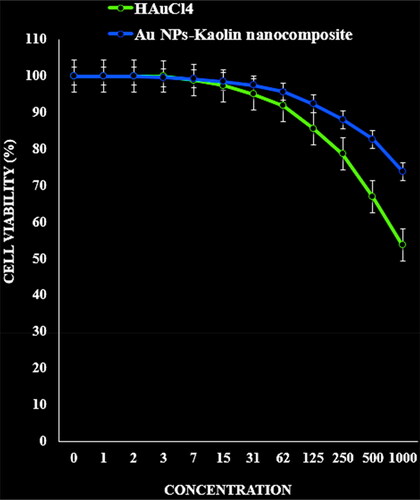
Table 1. The IC50 of HAuCl4 and Kaolin@extract/Au nanocomposite in the anti-human oral squamous cell carcinoma test.
The viability of malignant human oral cell lines (HSC-2, HSC-3, HSC-4 and Ca9-22) reduced dose-dependently in the presence of Kaolin@extract/Au nanocomposite.
The IC50 of Kaolin@extract/Au nanocomposite were 277, 367, 250 and 545 µg/mL against HSC-2, HSC-3, HSC-4 and Ca9–22 cell lines, respectively (; and ).
Figure 7. The anti-human oral squamous cell carcinoma properties (cell viability (%)) of HAuCl4 and Kaolin@extract/Au nanocomposite (concentrations of 0–1000 µg/mL) against HSC-2 and HSC-3 cell lines.
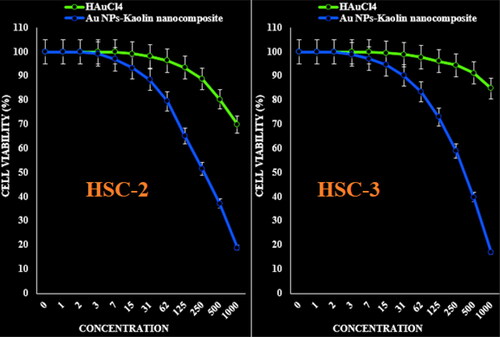
Figure 8. The anti-human oral squamous cell carcinoma properties (cell viability (%)) of HAuCl4 and Kaolin@extract/Au nanocomposite (concentrations of 0–1000 µg/mL) against HSC-4 and Ca9-22 cell lines.
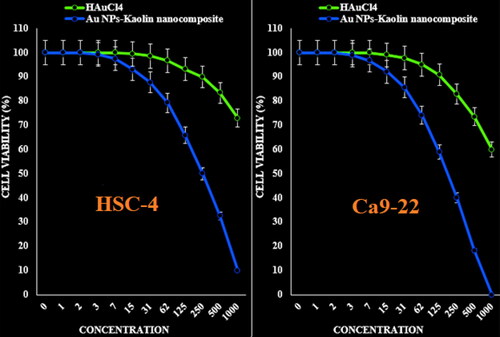
The cytotoxic effect of gold nanoparticles is the result of active physicochemical interaction of gold atoms with the functional groups of intracellular proteins, as well as with the nitrogen bases and phosphate groups in DNA [Citation51]. In previous report, Sriram et al. [Citation52] reported that the nanoparticles acquiring anticancer properties are known for their potential ability to slow down the activities of abnormally expressed signalling proteins, such as Akt and Ras, cytokine-based therapies, DNA- or protein based vaccines against specific tumour markers, and tyrosine kinase inhibitors which exhibit a consistent antitumor effect [Citation53].
Nam et al. [Citation54] designed functionalized gold nanoparticles using dendrimers for fight cancer cells. Similarly, the nanoparticles synthesized by brown seaweed Ulva show good cytotoxic activity against human laryngeal cancer (Hep-2) cell line, human breast cancer (MCF 7) cell line and human colon cancer (HT 29) cell line [Citation55] and breast cancer line MCF-7 [Citation56]. Recently, Rajeshkumar et al. [Citation57] synthesized the nanoparticles and reported their great potential to inhibit the cell viability of liver and lung cancer cell lines.
In this report, the anticancer activity was observed and that the synthesized gold nanoparticles induce a dose dependent inhibition activity against oral cancer cells. Some of the approved chemotherapeutic agents were caused side effect and high cast. Therefore, there is an important need to develop alternative medicines against this deadly disease. Synthesized gold nanoparticles to fulfil the need of new therapeutic treatment were discovered.
4. Conclusions
In this study, an eco-friendly approach described for in situ immobilization of Au nanoparticles on the surface of Kaolin mediated by Pomegranate peel extract, without using any toxic reducing and capping agents. The prepared Kaolin@extract/Au nanocomposite were characterized through a number of advanced analytic techniques. The viability of malignant human oral cell lines reduced dose-dependently in the presence of Kaolin@extract/Au nanocomposite. The IC50 of Au NPs-Kaolin nanocomposite were 277, 367, 250 and 545 µg/mL against HSC-2, HSC-3, HSC-4 and Ca9-22 cell lines, respectively. After clinical study, Kaolin@extract/Au nanocomposite can be utilized as an efficient drug in the treatment of human oral squamous cell carcinoma in humans.
Disclosure statement
No potential conflict of interest was reported by the authors.
Additional information
Funding
References
- Borm PJ, Robbins D, Haubold S, et al. The potential risks of nanomaterials: a review carried out for ECETOC. Part Fibre Toxicol. 2006;3(1):11.
- De Jong WH, Borm PJ. Drug delivery and nanoparticles: applications and hazards. Int J Nanomedicine. 2008;3(2):133–149.
- Mao B-H, Tsai J-C, Chen C-W, et al. Mechanisms of silver nanoparticle-induced toxicity and important role of autophagy. Nanotoxicology. 2016;10(8):1021–1040.
- You C, Han C, Wang X, et al. The progress of silver nanoparticles in the antibacterial mechanism, clinical application and cytotoxicity. Mol Biol Rep. 2012;39(9):9193–9201.
- Trojer MA, Li Y, Wallin M, et al. Charged microcapsules for controlled release of hydrophobic actives part II: surface modification by LbL adsorption and lipid bilayer formation on properly anchored dispersant layers. J Colloid Interface Sci. 2013;409:8–17.
- Liu D, Chen L, Jiang S, et al. Formulation and characterization of hydrophilic drug diclofenac sodium-loaded solid lipid nanoparticles based on phospholipid complexes technology. J Liposome Res. 2014;24(1):17–26.
- Tong AM, Lu WY, Xu JH, et al. Bioorg. Med. Chem. Lett. 2004;14:2095–2097.
- T, Baran I, Sargin M, Kaya, et al. Clean Technol Environ Policy. 2021;23:1797–1806.
- Wang X, Hu P, Xue F, et al. Cellulose-supported N-heterocyclic carbene-palladium catalyst: synthesis and its applications in the suzuki cross-coupling reaction. Carbohydr Polym. 2014;114:476–483.
- Veisi H, Sedrpoushan A, Faraji AR, et al. RSC Adv. 2018;810:69–77.
- Veisi H, Joshani Z, Karmakar B, et al. Ultrasound assisted synthesis of Pd NPs decorated chitosan-starch functionalized Fe3O4 nanocomposite catalyst towards Suzuki-Miyaura coupling and reduction of 4-nitrophenol. Int J Biol Macromol. 2021;172:104–113.
- Budarin VL, Clark JH, Luque R, et al. Palladium nanoparticles on polysaccharide-derived mesoporous materials and their catalytic performance in C–C coupling reactions. Green Chem. 2008;10(4):382–387.
- Shaabani A, Rahmati A, Badri Z. Sulfonated cellulose and starch: New biodegradable and renewable solid acid catalysts for efficient synthesis of quinolines. Catal Commun. 2008;9(1):13–16.
- Zhou CH. An overview on strategies towards clay-based designer catalysts for green and sustainable catalysis. Appl Clay Sci. 2011;53(2):87–e96.
- Lin Y, Li L, Hu L, et al. Multifunctional poly(dopamine)-assisted synthesis of silver nano particles/carbon nanotubes nanocomposite: toward electrochemical sensing of hydrogen peroxide with enhanced sensitivity. Sensor Actuat B-Chem. 2014;202:527–535.
- Ramprakash Upadhyay P, Srivastava V. Clays: an encouraging catalytic support. CCAT. 2016;5(3):162–181.
- He K, Zeng Z, Chen A, et al. Advancement of Ag-graphene based nanocomposites: an overview of synthesis and its applications. Small. 2018;14(32):1800871.
- Ma H, Wang B, Wang Y. Application of molybdenum and phosphate modified kaolin in electrochemical treatment of paper mill wastewater. J Hazard Mater. 2007;145(3):417–423.
- Kurtan U, Amir M, Yıldız A, et al. Synthesis of magnetically recyclable MnFe 2 O 4 @SiO 2 @Ag nanocatalyst: its high catalytic performances for azo dyes and nitro compounds reduction. Appl Surf Sci. 2016;376:16–25.
- Ai L, Zeng C, Wang Q. One-step solvothermal synthesis of Ag-Fe3O4 composite as a magnetically recyclable catalyst for reduction of rhodamine B. Catal Commun. 2011;14(1):68–e73.
- Lu Y, Wan X, Li L, et al. Synthesis of a reusable composite of graphene and silver nanoparticles for catalytic reduction of 4-nitrophenol and performance as anti-colorectal carcinoma. J Mater Res Technol. 2021;12:1832–1843.
- Yang F, Jin C, Jiang Y, et al. Liposome based delivery systems in pancreatic cancer treatment: from bench to bedside. Cancer Treat Rev. 2011;37(8):633–642.
- Xinli DHZS. Applications of nanocarriers with tumor molecular targeted in chemotherapy. Chemistry. 2012; 75(7):621–627.
- Allen TM. Ligand-targeted therapeutics in anticancer therapy. Nat Rev Cancer. 2002;2(10):750–763.
- Byrne JD, Betancourt T, Brannon-Peppas L. Active targeting schemes for nanoparticle systems in cancer therapeutics. Adv Drug Del Rev. 2008;60(15):1615–1626.
- Gao J, Wang Z, Liu H, et al. Liposome encapsulated of temozolomide for the treatment of glioma tumor: preparation, characterization and evaluation. Drug Discov Ther. 2015;9(3):205–212.
- Mohammed MI, Makky AM, Teaima MH, et al. Transdermal delivery of vancomycin hydrochloride using combination of nano-ethosomes and iontophoresis: in vitro and in vivo study. Drug Deliv. 2016;23(5):1558–1564.
- Li YN, G F. Recent progress in doxorubicin nano-drug delivery systems for reserving multidrug resistance. 2014;11(3):177–181.
- Torchilin VP. Targeted pharmaceutical nanocarriers for cancer therapy and imaging. AAPS J. 2007;9(2):E128–E147.
- Deshpande PP, Biswas S, Torchilin VP. Current trends in the use of liposomes for tumor targeting. Nanomedicine (Lond)). 2013;8(9):1509–1528.
- Zhang Y, Huang Y, Li S. Polymeric micelles: nanocarriers for cancer-targeted drug delivery. AAPS PharmSciTech. 2014;15(4):862–871.
- Gao Z, Lukyanov AN, Singhal A, et al. Diacyllipid-polymer micelles as nanocarriers for poorly soluble anticancer drugs. Nano Lett. 2002;2(9):979–982.
- Davis ME, Chen Z, Shin DM. Nanoparticle therapeutics: an emerging treatment modality for cancer. Nat Rev Drug Discov. 2008;7(9):771–782.
- Matsumura Y, Hamaguchi T, Ura T, et al. Phase I clinical trial and pharmacokinetic evaluation of NK911, a micelle-encapsulated doxorubicin. Br J Cancer. 2004;91(10):1775–1781.
- Nie S, Xing Y, Kim GJ, et al. Nanotechnology applications in cancer. Annu Rev Biomed Eng. 2007;9:257–288.
- Dykman L, Khlebtsov N. Gold nanoparticles in biomedical applications: Recent advances and perspectives soc. Chem Soc Rev. 2012;41(6):2256–2282.
- Dreaden EC, Alkilany AM, Huang X, et al. The golden age: gold nanoparticles for biomedicine. Chem Soc Rev. 2012;41(7):2740–2779.
- Abraham GE, Himmel PBJ. Management of rheumatoid arthritis: rationale for the use of colloidal metallic gold. J Nutr Environ Med. 1997;7:295–305.
- Abraham GE. Gold nanoparticles in biology and medicine: recent advances and prospects. Acta Nat. 2008;15:132–158.
- Tsai CY, Shiau AL, Chen SY. Methotrexate conjugated to gold nanoparticles inhibits tumor growth in a syngeneic lung tumor model. Mol Pharm. 2007;56:544–554.
- Brown CL, Whitehouse MW, Tiekink ERT, et al. Colloidal metallic gold is not bio-inert. Inflammopharmacology. 2008;16(3):133–137.
- Paciotti GF, Myer L, Weinreich D, et al. Colloidal gold: a novel nanoparticle vector for tumor directed drug delivery. Drug Deliv. 2004;11(3):169–183.
- Paciotti GF, Kingston DGI, Tamarkin L. Colloidal gold nanoparticles: a novel nanoparticle platform for developing multifunctional tumortargeted drug delivery vectors. Drug Dev Res. 2006;67(1):47–54.
- Park H, Tsutsumi H, Mihara H. Cell penetration and cell-selective drug delivery using α-helix peptides conjugated with gold nanoparticles . Biomaterials. 2013;34(20):4872–4879.
- Dreaden EC, Austin LA, Mackey MA, et al. Size matters: gold nanoparticles in targeted cancer drug delivery. Ther Deliv. 2012;3(4):457–478.
- Remant Bahadur KC. Gold nanoparticle-based gene delivery: promises and challenges. Nanotechnol Rev. 2014;3(3):269–280.
- Mendes R, Fernandes A, Baptista P. Gold nanoparticle approach to the selective delivery of gene silencing in cancer the case for combined delivery? Genes. 2017;8(3):94.
- Huang X, Jain PK, El-Sayed IH, et al. Plasmonic photothermal therapy (PPTT) using gold nanoparticles. Lasers Med Sci. 2008;23(3):217–228.
- Cruz LJ, Tacken PJ, Rueda F, et al. Targeting nanoparticles to dendritic cells for immunotherapy. Methods Enzymol. 2012;509:143–163.
- Andersson HA, Kim Y-S, O'Neill BE, et al. HSP70 promoterdriven activation of gene expression for immunotherapy using gold nanorods and near infrared light. Vaccines. 2014;2:216–227.
- Blagoi Yu P, Galkin VL, Gladchenko GO, et al. Metal complexes of nucleic acids in solutions. Naukova Dumka: Kiev; 1991. p. 272.
- Sriram MI, ManiKanth SB, Kalishwaralal K, et al. Antitumor activity of silver nanoparticles in Dalton’s lymphoma ascites tumor model. Int J Nanomed. 2010;5:753–762.
- Martins D, Frungillo L, Anazzetti MC, et al. Antitumoral activity of L-ascorbic acid-poly- D,L-(lactide-co-glycolide) nanoparticles containing violacein. Int J Nanomed. 2010;5:77–85.
- Nam J, Won N, Jin H, et al. pH-Induced aggregation of gold nanoparticles for photothermal cancer therapy. J Am Chem Soc. 2009;131(38):13639–13645.
- Devi JS, Bhimba BV. Anticancer activity of silver nanoparticles synthesized by the seaweed Ulva lactuca in vitro. Sci Rep. 2012;1(4):242.
- Holt JG, Krieg RN, Sneath PHA, et al. Bergey’s manual of determinative bacteriology. 9th ed. Baltimore: Williams and Wilkins;1994.
- Rajeshkumar S, Malarkodi C, Vanaja M, et al. Anticancer and enhanced antimicrobial activity of biosynthesizd silver nanoparticles against clinical pathogens. J Mol Struct. 2016;1116:165–173.