Abstract
Glioblastoma multiforme (GBM) is the most aggressive and deadliest type of brain tumor with a > >th he most aggressive and deadliest type of brain tumor witnear-infrared fluorescent dye, but it is hard to directly target brain tumor. Therefore, our study aimed to develop a new carrier for ICG to accurately visualize cancer tissue during surgery. Exosomes were isolated from culture U-87 MG cells, then loaded with Indocyanine green (ICG) and conjugated with RGERPPR peptide (RGE) (R-exo-ICG). The parameters of R-exo-ICG were analyzed and injected into tumor-bearing nude mice to evaluate its anti-tumor effect. R-exo-ICGs were nano size, and completely released ICG within 24 h. RGE modification increased cell uptake of exosomes, and induced cell apoptosis through activating Caspase 3 signaling pathway after laser irradiation. Moreover, R-exo-ICG was largely accumulated in the brain tumor in vivo, and inhibited tumor growth in tumor-bearing mice. RGE-conjugated exosomes that loaded ICG could target brain tumor in mice and significantly inhibited tumor growth in mice, which suggested that R-exo-ICG might serve as a novel approach for GBM treatment.
1. Introduction
Glioblastoma, also known as glioblastoma multiforme (GBM), is the most aggressive form of cancer that begins in the brain, and it is the deadliest type of brain tumor with a > >th lastoma, alsoity. GBM has an unsatisfied median survival of 12.6 months due to the limitations of current treatment, including tumor location in the brain, the high average age of patients at diagnosed, and poor current understandings of pathophysiology [Citation1]. Therefore, due to the unsatisfied outcome of current therapeutic strategies, including chemotherapy strategies, the resection technique, and radiation therapy, new improvement of GBM treatment is required.
Exosomes are membrane-bound extracellular vesicles that are produced in the endosomal compartment of most eukaryotic cells. Exosomes were found in biological fluids, including blood, urine and cerebrospinal fluid, as well as tissue matrix (matrix-bound nanovesicles) [Citation2]. Exosomes that contain variety of molecular constituments of their original cells, including protein, DNA and various RNAs, can be released from cells, and their component profiles vary based on the cell and tissue of origin. Therefore, exosomes are identified as key players in cancer and potential therapeutic strategy [Citation3].
Nanotherapy is a newly-developed nanotechnology using nanoparticles for treating various aliments [Citation4]. As endogenous panovesicles, exosomes have strong carrying ability and can smoothly cross the blood-brain barrier, becoming a promising drug delivery vehicle in the brain, which is attracting more and more attention [Citation5]. However, most of the unmodified exosomes cannot be efficiently aggregated in tumor tissue after entering the circulating system, the efficacy of the loaded drug will be affected, and the biological safety of the treatment is difficult to guarantee. For most cell-derived exosomes, after entering into the circulating system, most of the exosomes rapidly accumulate in the monocyte-macrophage system and are cleared from the blood circulation by the macrophages. Previous studies have shown that the exosome membrane is rich in phosphatidylserine (PS), which makes the surface of the exosome membrane negatively charged, and can also promote the binding of PS receptors on the surface of the exosome and macrophage membrane, and then mediates the phagocytosis and clearance of exosomes by macrophages [Citation6]. Therefore, the targeted modification of exosomes is crucial.
Fluorescent probes for in vivo optical imaging are an emerging technology that can accurately observe tumors, such as GBM, breast cancer, head, and neck squamous cell carcinoma (HNSCC), hepatocellular carcinoma [Citation7–14], and even observe residues cells during surgery.
Indocyanine green (ICG) is a clinical near-infrared fluorescent dye that has high active oxygen quantum yield under the induction of near-infrared laser. It not only has strong photodynamic performance, but also has certain photothermal performance. Therefore, it can be used for photodynamic therapy of tumors and auxiliary photothermal therapy. Previous study discovered that tumors absorb more ICG than other tissues, as evidenced by 2.5 times the light responses of tumor than neighboring tissues when ICG was injected near tumor [Citation15]. However, due to its unstable property in a liquid environment, ICG can be easily cleared in the blood circulation, contributing to lack of targeting, which results in insufficient bioavailability at tumor sites and limiting its application in cancer therapy. Therefore, our current study aimed to develop a new carrier for ICG to direct target brain tumor for the improvement of GBM treatment.
2. Materials and methods
2.1. Cell culture
U-87 MG cell (ATCC, Manassas, VA) is a cell line from human glioblastoma astrocytoma and cultured in DMEM medium with 10% exosome-depleted fetal bovine serum (FBS, Gibco, Grand Island, NY). To deplete exosomes, FBS were centrifuged at 120,000 g for 18 h.
2.2. Isolation and purification of exosomes
After culturing for 48 h, around 1000 ml of supernatant were collected for different centrifugation several times as previously described [Citation16]. After the last centrifugation, pelleted exosomes were resuspended in phosphate-buffered saline (PBS) and kept at −80 °C for the following studies.
2.3. Loading ICG or Cy5 into exosomes by electroporation
To load ICG or Cy5 into exosomes, we did pre-experiments to explore the optimal reaction parameters and ratio of components in the reaction system. Finally, we found the best condition under the optimal electroporation condition (voltage: 400 V, electric capacity: 150 mF and discharging time: 1 ms). Exosomes that loaded ICG or Cy5 were generated as previously described [Citation16].
2.4. Conjugation of RGE-peptide to ICG-loading exosomes
RGE conjugated into exosomes by a cycloaddition reaction of sulfonyl azide as previously described [Citation16]. Briefly, the size and morphology were measured using ZetaView nanoparticle tracking analyzer (Particle Metrix, Germany) and the HT7700 TEM Hitachi; western blot procedures.
R-exo-ICG: RGE conjugated exosomes that loaded ICG;
F-exo: free exosomes;
R-exo-Cy5: RGE conjugated exosomes that loaded Cy5;
F-exo-Cy6: Free exosomes that loaded Cy5.
2.5. Western blot
Purified exosomes or cells that uptake different exosomes were lysed in radioimmunoprecipitation buffer to extract protein, and protein concentration was measured using BCA kit. Western blot was performed as previously described [Citation17]. GAPDH was used as loading control.
2.6. Co-localization analysis
Purified exosomes were added into medium in the dish, then a super-resolution fluorescence microscope was used to observe the colocalization of FITC-labeled RGE and Cy5 loaded exosomes. Exosomes were uptake by U-87 MG cells, then cells were observed co-localization by fluorescent microscope, and were analyzed the efficacy of U-87 cells uptake of different exosomes by flow cytometry.
2.7. Cell apoptosis
U-87 MG cells were treated with different concentrations of exosomes with or without laser irradiation for 48 h, then cell apoptosis was measured using cell counting kit 8 [Citation18].
2.8. Tumor mouse model
We injected U-87 MG cells into BALB/c nude mice to induce brain tumor in vivo, then mice were injected with PBS,1.5 mg/kg ICG or R-exosomes that loaded 1.5 mg ICG through tail vein. After 24 h, mice were placed under IVIS Spectrum in vivo imaging systems to observe in vivo imaging.
Mice were weighted every three days, and survival rate was recorded. After 12 days mice were sacrificed, and tumors were collected and weighted. Animal studies were approved by The Second Hospital of Hebei Medical University.
2.9. Statistical analysis
Data were expressed as means ± SD, Statistical analysis was performed using a one-way analysis of variance (ANOVA) test, with ***p < 0.001, **p < 0.01, and *p < 0.05.
3. Results
3.1. Characterization of F-exos and R-exo-ICGs
We first evaluated the characterizations of our modified exosomes. TEM images showed that the diameters of both the free-exosomes (F-exo, ) and RGERPPR peptide (RGE-modified exosomes that loaded ICG (R-exo-ICG, ) were around 80 nm. DLS results indicated that the diameters of two exosomes were about 120 nm (). The difference on the diameters was caused by the different principles from these two methods. The stabilities of R-exo0ICG in different buffers, including water, PBS, FBS and blood, were shown in . Western Blot detected the specific membrane proteins, including CD63 and CD81, in R-exo-ICG and F-exo (). Then we observed drug release by R-exo-ICGs and found that R-exo-ICG released about 80% if ICG at 12 h, and basically completed the release within 24 h ().
Figure 1. Characterization of F-exos and R-exo-ICGs. Representative TEM images of F-exos (a) and R-exo-ICGs (b), scale bar =100 nm; Size distributions of F-exos (c) and R-exo-ICGs (d); (e) CD63 and CD81 expression in F-exos and R-exo-ICGs were analyzed by western blot. GAPDH was used as loading control. (f) Drug release of R-exo-ICGs.
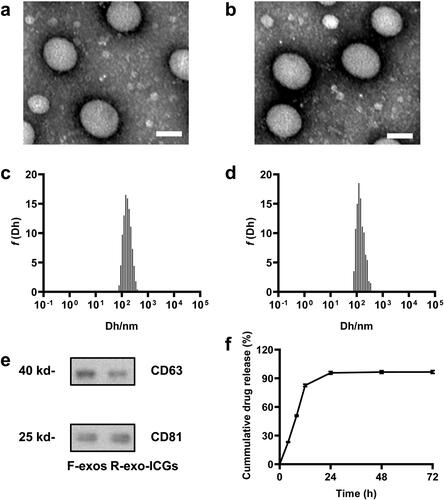
3.2. RGE-modified exosomes increased its uptake by cells
To verify the modification of RGE in R-exo-ICG, we did a series of experiments. First, FITC-labeled RGE was co-localized with Cy5-labeled exosomes, which confirmed that exosomes were modified by RGE (). FITC-labeled RGE was still co-localized with Cy5-exosomes at the cellular level (). In addition, RGE was also co-localized in PKH26 (), which further confirmed the co-localization of RGE on the membrane. Then we used flow cytometry to measure the effect of RGE modification on cell uptake of exosomes. The efficiency of U-87 MG cells uptake of R-exo-Cy5 was significantly higher than that of F-exo-Cy5 (). These data confirmed that exosomes modified by RGE can significantly increase its uptake by cells.
Figure 2. Verification of RGE conjugating on the membrane of Exos and RGE effects on cellular uptake. (a) R-exo-ICGs directly measured by a super-resolution fluorescence microscope the green fluorescence represents FITC; (b) Confocal microscopy images of R-exo-ICGs, the red arrow indicated the ICG, the green fluorescence (FITC-RGE), and the yellow fluorescence (merged) respectively; (c) Flow cytometric analysis of F-exos-ICGs and R-exo-ICGs.
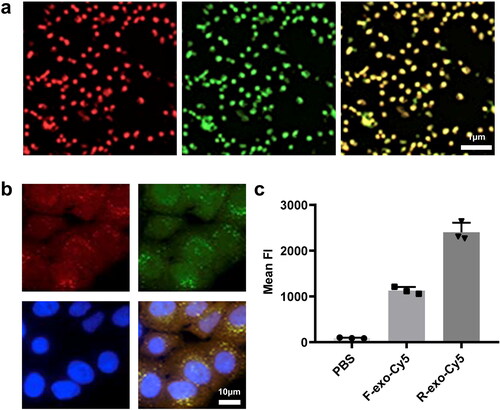
3.3. R-exo-ICG promoted cell apoptosis
U-87 MG cells were treated with different concentrations of R-exo-ICG for 48 h with or without laser irradiation. Without laser irradiation, R-exo-ICG did not alter cell apoptosis (), but laser irradiation significantly increased cell apoptosis in a dose-dependent manner (), which suggested that after laser irradiation, R-exo-ICG uptake by cancer cells dose-dependently increased its ability to kill cancer cells. Furthermore, compared to WT or ICG-treated cells, R-exo-ICG-treated cells increased the expressions of caspase3 and cleaved caspase 3 (), which suggested that R-exo-ICG activated caspase3-mediated signaling pathway in cancer cells to induce the apoptosis of cancer cells.
Figure 3. Effect of R-exo-ICGs in killing U-87 MG cells in vitro. The cell viability percent of different concentrations of R-exo-ICGs on U-87 MG cells without (a) or with Laser irradiation (b), n = 6. Western blot data (c) and statistics (d) of caspase 3 and cleaved caspase 3. n = 3. Data were expressed as means ± SD, Statistical analysis was performed using a one-way ANOVA test, with ***p < 0.001, **p < 0.01, and *p < 0.05.
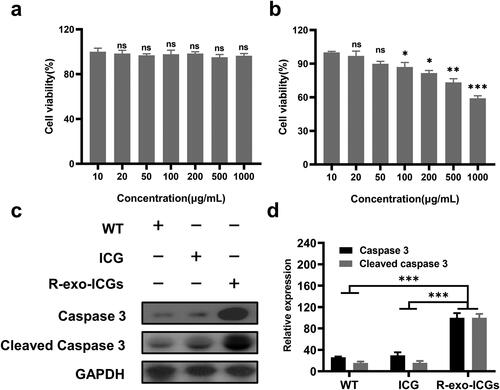
3.4. R-exo-ICG was accumulated in the tumor of mice
Next, we performed fluorescent imaging after 1 day of injecting R-exo-ICG in mice. As shown in , we observed the special accumulation of R-exo-ICG in brain tumors as evidenced by the enhanced fluorescence in the tumor location. At the same time, the fluorescent intensity in the tumor was weaker in ICG-injected mice. It was further found that R-exo-ICG could preferably accumulate in the brain other than other tissues when compared to ICG, as evidenced by the fluorescence in the organs (). These data indicated that exosomes modified by RGE can significantly increase its uptake by brain tumor in mice.
3.5. R-exo-ICG effectively inhibited tumor growth in tumor-bearing mice
To determine the anti-tumor effect of R-exo-ICG on the progression of GBM in vivo, we subcutaneously injected U-87 MG cells into nude mice to induce tumor. Compared to PBS or ICG injection, R-exo-ICG injection significantly reduced tumor weight and enhanced survival rate without the alteration of body weight (), which suggested that R-exo-ICG could effectively inhibit tumor growth in vivo.
Figure 5. Antitumor efficacy of ICG and R-exo-ICGs. The changes (a) tumor tissue weight, (b) body weight, and (c) survival rates of the three groups of tumor-bearing mice during the 12-d treatment. Data were expressed as means ± SD, n = 8. Statistical analysis was performed using a one-way ANOVA test, with ***p < 0.001, **p < 0.01, and *p < 0.05.
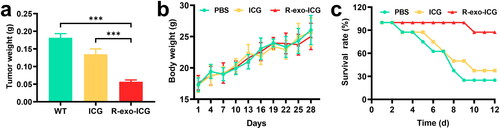
4. Discussion
As a heterogeneous and complex tumor, GBM is considered impossible to cure. In the past 10–15 years, the management of GBM, including chemotherapic strategies, the resection technique, and radiation therapy has slowly evolved, however these improvements have not translated into remarkable increase in the survival of GBM patients. Therefore, better management of GBM is needed, which dependents on improvement in comprehensive understanding of oncology and surgical techniques. In contrast, optical imaging can achieve non-invasive real-time in-vivo visualization with high temporal and spatial resolution, in which patients can’t be affected by ionizing radiation like X-rays from CT. Therefore, it can be long-term used to visualize living organisms and tissues safely and repeatedly without any harmful effects. As well known, accurate visualization of cancer tissue can help surgeons distinguish diseased and healthy tissues during surgery. The optical imaging strategy to improve GBM scribing is ideal for guiding surgeons to identify and remove cancerous tissue to achieve a negative resection margin, while retaining healthy and valuable brain tissue. Although surgical treatment may not exist alone, to achieve the purpose of complete resection, targeted tumor visualization and progress in surgical guidance are necessary and may increase postoperative survival. At present, the four fluorophores, including methylene blue, sodium fluorescein, ICG and 5-aminolevulinic acid (5-ALA) have been approved by the US Food and Drug Administration (FDA) to use in humans [Citation19, Citation20].
Exosomes are nano-sized lipid bilayer microcapsules with a diameter in the range of 40–150 nanometers. They are secreted by almost all types of cells, and secreted large amounts of proteins, coding and non-coding RNA and lipids, which play an important role in crosstalk, communication, and transportation between different cells. Exosomes have several advantages than other carriers, including low immunogenicity, biodegradable, non-toxic, strong loading and carrier protective capabilities, and strong ability to cross blood-brain barrier (BBB). However, if there is no selection of cell source and membrane modification, exosomes lack natural targeting ability, so it is necessary to do some modification on exosomes. There are many ways to achieve this, one of which is the in vivo method that selects a suitable source cell or transfects the target protein into the source cell through plasmid. However, these methods are limited by the source cells with low and uncontrollable efficiency. Recently, emerging studies have confirmed that it is feasible to directly modify the surface of exosomes, called an in vitro method, and its targeting ability has been confirmed in animal experiments [Citation21]. This method is more direct and effective than the in vivo method and may become a promising approach.
Neuropilin-1 (NRP-1) is a transmembrane glycoprotein that is overexpressed in glioma cells and tumor vascular endothelium with no expression in normal nerve cells and other tissues, suggesting that it can be used as a targeting ligand for glioma [Citation16]. RGE is a specific ligand of NPR-1 and can penetrate tumor tissues to significantly enhance the anti-tumor effect of modified drugs. That is the reason we designed a RGE-modified exosome-loaded ICG system to locate and kill gliomas [Citation22, Citation23].
There are three advantages of ICG-loaded exsomes over traditional liposome ICG. Compared with existing artificial liposomes, good biocompatibility is one of its advantages as drug carriers. Currently, liposomes are the primary delivery device for siRNA and other RNAs, which can cause toxic immune responses in the human body and cause accumulation of toxicity to organs such as liver, eventually unsatisfied outcome. Exosomes have better biocompatibility and can overcome the shortcomings of liposome-induced immunogenicity and accumulated toxicity in vivo. Another advantage of exosomes as drug carriers is their biological stability. Antigen-presenting cell-derived exosomes can express membrane-bound complement regulators CD55 and CD59 to enhance the stability of circulation in vivo. Previous studies have shown that exosomes have a longer circulating time in vivo even when exposed to inflammatory conditions [Citation24]. Numerous studies have demonstrated that nanoparticles can achieve targeted aggregation to tumor tissue through enhanced penetration and retention (EPR) due to their small size (≤100 nm). The size of exosomes is between 30 and 100 nm, and the targeting effect on tumor tissue also follows the EPR effect [Citation25]. Moreover, certain cell-derived exosomes have intrinsic targeting functions. For example, exosomes derived from central nervous cells can cross the blood-brain barrier and target specific neurons [Citation26]. Exosomes derived from hypoxic tumor cells are prone to be recruited into hypoxic tumor tissues [Citation27]. The results of biodistribution studies also showed that the accumulation of exosomes in tumor tissue depending on the parent cell type. Therefore, when studying exosomes that target specific tissues or cells, it is necessary to consider the effect of their source cells on the targeting efficiency.
Our study generated a new exosome that conjugated with RGE and loaded with ICG that effectively targeted brain tumor and inhibited tumor growth in mice. RGE modification significantly increased the efficacy of uptake by cancer cells. Moreover, this RGE-modified exosome strategy significantly enhanced ICG's glioma localization, and this strategy also improved the photothermal killing effect of ICG on glioma. Therefore, our study might provide a new aspect for treating GBM.
5. Conclusion
Our study generated a new exosome that conjugated with RGE and loaded with ICG that effectively targeted brain tumor and inhibited tumor growth in mice. RGE modification significantly increased the efficacy of uptake by cancer cells. Moreover, this RGE-modified exosome strategy significantly enhanced ICG's glioma localization, and this strategy also improved the photothermal killing effect of ICG on glioma. Therefore, our study might provide a new aspect for treating GBM.
Supplemental Material
Download MS Word (302.2 KB)Acknowledgements
The authors would like to thank Dr Guo-zhu Sun, Dr Bao-hua Jiao and Dr Zong-mao Zhao for their technical advice.
Disclosure statement
The authors declare that they have no known conflicts of interest.
Data availability statement
Data could be obtained upon reasonable request to the corresponding author.
Additional information
Funding
References
- Carlsson SK, Brothers SP, Wahlestedt C. Emerging treatment strategies for glioblastoma multiforme. EMBO Mol Med. 2014;6(11):1359–1370.
- Huleihel L, Hussey GS, Naranjo JD, et al. Matrix-bound nanovesicles within ECM bioscaffolds. Sci Adv. 2016;2(6):e1600502.
- Dai J, Su Y, Zhong S, et al. Exosomes: key players in cancer and potential therapeutic strategy. Signal Transduct Target Ther. 2020;5(1):145.
- Ingle AP, Gupta I, Duran N, et al. Nanotherapy: a next generation hallmark for combating cancer. Nanostructures for cancer therapy. Amsterdam: Elsevier; 2017. p. 811–830.
- Sun D, Zhuang X, Zhang S, et al. Exosomes are endogenous nanoparticles that can deliver biological information between cells. Adv Drug Deliv Rev. 2013;65(3):342–347.
- Skotland T, Hessvik NP, Sandvig K, et al. Exosomal lipid composition and the role of ether lipids and phosphoinositides in exosome biology. J Lipid Res. 2019;60(1):9–18.
- Liu L, Lv RJ, Leung JK, et al. A near-infrared biothiol-specific fluorescent probe for cancer cell recognition. Analyst. 2019;144(16):4750–4756.
- Fan L, Wang X, Ge J, et al. A lysosome-targeting and polarity-specific fluorescent probe for cancer diagnosis. Chem Commun (Camb). 2019;55(32):4703–4706.
- Siriwibool S, Kaekratoke N, Chansaenpak K, et al. Near-Infrared fluorescent pH responsive probe for targeted photodynamic cancer therapy. Sci Rep. 2020;10(1):1283.
- Cui H, Wang R, Zhou Y, et al. Dual-function fluorescent probe for cancer imaging and therapy. Luminescence. 2016;31(3):813–820.
- Fu S, Wan X, Du C, et al. A novel fluorescent probe for the early detection of prostate cancer based on endogenous zinc sensing. Prostate. 2019;79(12):1378–1385.
- Yu Q, Huang S, Wu Z, et al. Label-Free visualization of early cancer hepatic micrometastasis and intraoperative Image-Guided surgery by photoacoustic imaging. J Nucl Med. 2020;61(7):1079–1085.
- Lv J, Xu Y, Xu L, et al. Quantitative functional evaluation of liver fibrosis in mice with dynamic contrast-enhanced photoacoustic imaging. Radiology. 2021;300(1):89–97.
- Lv J, Li S, Zhang J, et al. In vivo photoacoustic imaging dynamically monitors the structural and functional changes of ischemic stroke at a very early stage. Theranostics. 2020;10(2):816–828.
- Ramírez Backhaus M, Calatrava-Fons A, Gómez-Ferrer Á, et al. ICG lymphography and fluorescence in pelvic lymphadenectomy for bladder and prostate cancer. Arch Esp Urol. 2019;72(8):831–841.
- Jia G, Han Y, An Y, et al. NRP-1 targeted and cargo-loaded exosomes facilitate simultaneous imaging and therapy of glioma in vitro and in vivo. Biomaterials. 2018;178:302–316.
- Liu Z, Luo H, Zhang L, et al. Hyperhomocysteinemia exaggerates adventitial inflammation and angiotensin II-induced abdominal aortic aneurysm in mice. Circ Res. 2012;111(10):1261–1273.
- Yu B, Liu Z, Fu Y, et al. CYLD deubiquitinates nicotinamide adenine dinucleotide phosphate oxidase 4 contributing to adventitial remodeling. Arterioscler Thromb Vasc Biol. 2017;37(9):1698–1709.
- Kurbegovic S, Juhl K, Chen H, et al. Molecular targeted NIR-II probe for Image-Guided brain tumor surgery. Bioconjug Chem. 2018;29(11):3833–3840.
- Wen Q, Zhang Y, Li C, et al. NIR-II fluorescent Self-Assembled peptide nanochain for ultrasensitive detection of peritoneal metastasis. Angew Chem Int Ed Engl. 2019;58(32):11001–11006.
- Arrighetti N, Corbo C, Evangelopoulos M, et al. Exosome-like nanovectors for drug delivery in cancer. Curr Med Chem. 2019;26(33):6132–6148.
- Antaris AL, Chen H, Cheng K, et al. A small-molecule dye for NIR-II imaging. Nat Mater. 2016;15(2):235–242.
- Jia Y, Wang X, Hu D, et al. Phototheranostics: active targeting of orthotopic glioma using biomimetic proteolipid nanoparticles. ACS Nano. 2019;13(1):386–398.
- Bonjoch L, Casas V, Carrascal M, et al. Involvement of exosomes in lung inflammation associated with experimental acute pancreatitis. J Pathol. 2016;240(2):235–245.
- Hong Y, Nam GH, Koh E, et al. Exosome as a vehicle for delivery of membrane protein therapeutics, PH20, for enhanced tumor penetration and antitumor efficacy. Adv Funct Mater. 2018;28(5):1703074.
- Khongkow M, Yata T, Boonrungsiman S, et al. Surface modification of gold nanoparticles with neuron-targeted exosome for enhanced blood–brain barrier penetration. Sci Rep. 2019;9(1):9.
- Park JE, Dutta B, Tse SW, et al. Hypoxia-induced tumor exosomes promote M2-like macrophage polarization of infiltrating myeloid cells and microRNA-mediated metabolic shift. Oncogene. 2019;38(26):5158–5173.