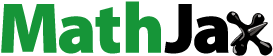
Abstract
A simple low-cost one-pot photodeposition synthesis with no hazardous reactants or products is used to make silver nanoparticles-activated carbon composite (SNPs@AC). The SNPs are homogenously and photodeposited and absorbed into the activated carbon matrix. Both SNPs and SNPs@AC composite have particle sizes around 10 nm and 100 nm, respectively. The SNPs@AC composite showed good antiviral activity to VERO (ATCC ccl-81) cells. Zeta potential of SNPs@AC composite is −25 mV, showing that this colloidal system is electrically stable and resistant to coagulation. For many Gram-positive and Gram-negative bacteria, the SNPs@AC composite demonstrated strong antibacterial efficacy. The SNPs@AC composite has 75.72 percent anti-inflammatory effect at concentration 500 µg/mL. This composite has a maximum non-toxic concentration (MNTC) of 78.125 g/mL, which corresponds to antiviral activity of up to 96.7 percent against hepatitis A. virus (HAV). It is suggested as a candidate for pharmaceutical formulations, such as integration into the manufacture of N95 masks for COVID-19 infection protection. Concentration 160 μg/mL SNPs@AC composite has antioxidant activity 42.74% percent. The SNPs@AC composite exhibited selective catalytic activity for the organosynthesis hydrazination reaction of 4-chloro-3, 5-di-nitro-benzo-triflouride, giving 1-hydroxy-4-nitro-6-trifluoro-methyl benzotriazole, a common antiviral drug for severe acute respiratory syndrome (SARS). SNPs@composite's well-defined pores provide suitable active sites for binding reactants: 4-Cl-3, 5-di-NO2-benzotriflouride, and hydrazine, which react to create 1-hydroxy-4-nitro-6-trifluoromethyl benzotriazole, which diffuses into solution away from the catalyst surface, leaving the catalyst surface unaffected.
1. Introduction
Nanotechnology is all about influencing atoms and structures. Many areas in chemistry, physics, and materials research have considerable overlap with nanoscience. These are the domains that found and understood the atom, established the science of combining atoms in precise structures, and developed methods to investigate and visualize these nanostructures. Nanotechnology differs from traditional materials science in that it deals with material modification and physical control at the atomic level [Citation1]. Nanotechnology-assisted materials synthesis could mean constructing it atom by atom [Citation1]. Silver nanoparticles (SNPs) have vast range of numerous industrial applications: biosensors, biomarkers, therapeutic agents for cancer [Citation2].
SNPs have valuable antimicrobial activity without side effects. Localized surface plasmon resonance gives SNPs unique properties such as broad-spectrum antimicrobial activity, enabled applications in optical devices in surface-enhanced Raman spectroscopy, biomedicine and biomarkers [Citation2]. SNPs are utilized for curing infections, mending of wound. Antimicrobial effect of SNPs provides a faster wound healing along with its efficacy against bacterial resistance to antibiotics. Because of non-harmful, safe inorganic nature, SNPs being utilized for quite a long time and is equipped for killing around 650 micro-organisms that cause infections [Citation3].
SNPs have high antibacterial activity to Staphylococcus aureus, Corticium salmonicolor, and Escherichia coli [Citation4]. Inhibition efficiency of SNPs for S. aureus is 99.9% at 5 ppm and its effective dose (ED50) for C. salmonicolor is 27.2 ppm [Citation5]. SNPs of particle size ranges from 1 nm to 100 nm have unique optical, electrical, catalytic, and are thermally stable based on surface nature, particle size, and shapes. Inkjet inks contain well-dispersed SNPs is used in electronic circuits. Composites containing both noble metal NPs adherent to surface of carbon nanotubes (CNTs) have potential application in optical electronics and heterogeneous electrocatalysis [Citation6]. AgCl@AC nanocomposite catalyzed photodegradation and chemical reduction of 4-nitrophenol to aminophenol but has not tested in organosynthesis of antiviral benzotriazoles yet [Citation7].
Metal NPs are efficient catalysts: silver and platinum NPs are used in photography and H2O2 decomposition respectively. Benzene to cyclohexane and CO to CO2 conversions are carried out using gold (Au) NPs. Oxide-supported AuNPs catalyzed CO-oxidation at low temperatures. Catalysis by metal NPs is applied in hydrogenations and oxidations reactions of unsaturated hydrocarbons, bond formation of C–C, C–S, C–N, photochemical reactions, synthesis of chiral organic compounds, and cycloaddition reactions. In addition to application as biosensors [Citation8], antibacterial formulations [Citation9], and eco-friendly filters for wastewater treatment [Citation10]. Quantum size dot SNPs decreasing treatment dose in the environmental and medical applications [Citation11].
Various hazard free, eco-friendly synthesis methods of SNPs include chemical, gamma-irradiation, thermal decomposition, photochemical and biosynthesis techniques [Citation7]. Two approaches are used to stabilize SNPs against coagulation: exploitation of chemical stabilizers such as cyclodextrin, SNPs deposition on large surface area substrates such as TiO2 powders [Citation7]. Activated carbon (AC) matrix as conventional eco-friendly biosorbent is derived from: chemviron, surplus sewage sludge, mineral acid-modified plants such as Euphorbia Antiqorum, pecan nutshell and other abundant biomass agriculture wastes such as orange peels after: cleaning, drying, carbonization under vacuum, activation at 850 °C followed by soaking in HCl under specified conditions. Commercial activated carbon (AC) has BET surface area 931.9 m2 g−1 (Quantachrome Nova 2000E, Surface Area, Pore Size Analyzer, particle size (µm), X-ray diffractometer) – Philips PW 1730, [email protected], Particle size (micrometer, µm) distribution of AC expressed as: (2.75, 55.89, 16.03, 21.14, 4.19) wt.% have particle size: ((32–16 µm), (16–8 µm), (8–4 µm), (4–2 µm), (<2 µm), respectively. The predominant particle size distribution of AC ranges from (16-8µm for 55.89 wt.%). AC has amorphous structure with a large internal surface area and pore volume is used in various liquid or gas-phase applications according to pore size distribution. AC for liquid-phase has significantly more pore volume in macropore range, which permits rapid liquids diffusion into mesopores and micropores of AC. The hydrophobicity of AC allows gas adsorption in humid atmosphere. AC is a twisted network of defective carbon layer planes joined together by aliphatic bridging groups. AC is a recyclable from various plant residues of economics and market growth. AC generally presents no particular health hazard as defined by National Institute for Occupational Safety and Health (NIOSH). However, it is a nuisance and mild irritant with respect to inhalation, skin contact, eye exposure, and ingestion. On the other hand, spent carbon may contain a concentration of toxic compounds [Citation7].
Nowadays, the preparation of antiviral heterocyclic compounds is a necessity concurrent with COVID-19 pandemic. Benzotriazoles are antiviral compounds for SARS-CoV that inactivates severe acute respiratory syndrome 3CL protease enzymatic (SARS) [Citation12,Citation13]. COVID-19 harmed almost all people around the globe, rapidly spread through inhalation air [Citation14], it is a conjugated or nucleoproteins bonded to a non-protein prosthetic group: sugar, nucleic acid, lipid, or some other groups [Citation15,Citation16]. One of the most important strategies for the defense against COVID-19 is the treatment by chemotherapeutic triazoles drugs which selectively destroy the virus without simultaneously destroying the host [Citation16]. Organic synthesis is very important for synthesis of therapeutic drugs and pharmaceutical formulation; however, it can be time-consuming and costly. The challenges of the use of organic catalysts add direct and indirect costs as these catalysts may toxic and hardly recovered from the reaction media. To keep the environment, the development of recycled and reused catalysts, such as silver nanoparticles at low concentrations is a necessity [Citation9,Citation17].
Hepatitis A virus (HAV) is a DNA virus that causes acute hepatitis leading to chronic hepatitis, liver cirrhosis and hepatic cancer. About four hundred millions HAV carriers are infected and more than one million deaths worldwide are reported annually due to HAV-related complications. Effective vaccination of selective antiviral therapeutic drugs alternatives alpha interferon against HAV infection is required. In HAV life cycle, antiviral chemotherapy targets reverse transcription step of pregenome using endogenous viral reverse transcriptase enzyme to incorporate nucleotide analogues in the minus strand of DNA. Nucleotide analogues competitively inhibit reverse transcriptase enzyme. Heterocyclic compounds such as pyrimidine's and diazoles have selective antiviral activities such as Lamivudine, a retroviral inhibitor for HAV replication both in vitro and in vivo. 1-hydroxy-4-nitro-6-trifluoromethyl benzotriazole is an antiviral compound for 12 DNA and RNA viruses including SARS and middle east respiratory syndrome (MERS–CoV) via inactivation of SARS 3CL protease enzyme [Citation9]. However, the synthesis roots for these organic compounds are very complicated and involve various toxic organic compounds [Citation14,Citation16].
This study aims to prepare SNPs@AC composite by novel a modified photochemical method and evaluate its catalytic activity for very important reaction: hydrazination of 4-Cl-3, 5-di-NO2-benzotriflouride derivatives. The objective is extended to evaluate SNPs@AC composite as a low-cost antiviral for HAV. The novelty in the current study represented in the fact it is the first time for facile photodeposition synthesis of potent antimicrobial SNPs onto AC matrix as natural feasible solid support. Such low-cost SNPs@AC composite possessed potent antiviral activity to HAV and efficiently catalyzed synthesis of the promising antiviral heterocyclic compound: 1-hydroxy-4-nitro-6-trifluoromethyl benzotriazole is very useful for formulation COVID-19 vaccines patents.
2. Materials and methods
2.1. Materials
The chemicals used in this study are all of the analytical grades and are used as received without further purification: silver nitrate (AgNO3) is obtained from Alpha Chemika Co., India, the activated carbon (AC), and acetic acid are purchased from Sigma Aldrich Co.
2.2. Silver nanoparticles-activated carbon synthesis approach
Silver nanoparticles-activated carbon (SNPs@AC) composite is formulated at the room temperature (summer season) following the photodeposition approach using quartz 100 Watt UV lamp [Citation1,Citation18]. An accurate weight of 0.01 g AC is firstly ground into a fine powder and then added to 50 mL of 0.1 M AgNO3 dissolved in double-distilled water. Drops of an aqueous solution of 1.0 M CH3COOH solution were used to adjusted pH at 2.4. The mixture is exposed to UV irradiation with continuous stirring for 2 h. The obtained mixture was centrifuged and washed with double-distilled water. Finally, the precipitate is left to dry at the room temperature, then it is ground with the mortar and pestle to a fine powder in the nanoscale for further investigation.
2.3. Synthesis of antiviral: 1-hydroxy-4-nitro-6-trifluoromethyl benzotriazole
The 1oorder kinetic reaction of reactive substrate 4-Cl-3, 5-di-NO2-benzotriflouride with hydrated hydrazine in the absence and the presence of 5% SNPs@AC composite was monitored spectrophotometrically under hydrazine concentration in a large excess [Citation12]. Water solvent is used in the presence of 0.001 M anionic surfactant (sodium dodecyl sulphate, SDS above critical micelle concentration (CMC) at 298oK. The SDS at 0.01 M solubilizes the reactants in water without use of any toxic organic solvents. The substrate 4-chloro-3, 5-dinitro-benzo trifluoride (4 × 10−5 M) is refluxed with large excess hydrazine (1.6 × 10−4 M) at 25 °C ± 0.2. Reaction products are monitored spectrophotometrically by measuring absorption UV–Vis. band of 1-hydroxy-4-nitro-6-trifluoromethyl benzotriazole at 700 nm maximum wavelength of the full curve of the product 1-hydroxy-4-nitro-6-trifluoromethyl benzotriazole yield (4.8 g, 91%).
The first order rates constant is calculated by using the following equation:
(1)
(1)
where Ao, At,
is the absorbance of the product at time zero time, any time (t), and infinity (at end of the reaction (absorbance becomes completely constant). Plots log (
–At) against time (t) gave good straight lines, the values of
for each kinetic run are experimentally determined and kobs. is calculated from slope of the obtained straight line. Pseudo-first-order rate constant (k) for the reaction is measured at the temperatures 25, 30, 35, 40, and 45 °C. Activation energy (Ea) of the reaction is calculated using Arrhenius equation (Equation2
(2)
(2) ).
(2)
(2)
where kT1, kT2 are the apparent pseudo-first-order rate constants at the temperatures T1 and T2, respectively
Activation energy, Ea of the reaction is calculated from slope (–Ea/2.303 R) of the straight lines obtained from plot (log kA versus reciprocal of absolute temperature (1/T).
The activation parameters for the titled reaction: enthalpy of activation (ΔH#), and entropy of activation (ΔS#) and free energy of activation (ΔG#) are calculated as follows:
ΔS# and ΔH# are calculated using rearranged Eyring equation
(3)
(3)
ΔG# is calculated using the equation:
(4)
(4)
3. Results and discussions
3.1. Surface analysis of SNPs (control sample) and SNPs@AC composite
,b showed TEM micrographs and the particle size of SNPs and composite SNPs@AC respectively. showed SNPs (a control sample prepared by photoreduction of AgNO3 using acetic acid in the absence of AC. SNPs are formed in well-dispersed spherically shaped nanoparticles with no aggregation or agglomeration. The particles of SNPs as quantum dots with are homogeneously distributed at a nearly regular inter-particle distance and no pores appeared and the average particle size distribution is shown to be less than 21 nm. On the other hand, the TEM micrograph, of SNPs@AC composite indicated that SNPs have been successfully loaded and incorporated homogenously and well dispersed onto the AC matrix.
Figure 1. TEM micrographs of a) SNPs (control sample), b) SNPs@AC and c) particle size distribution of SNPs and SNPs@AC.
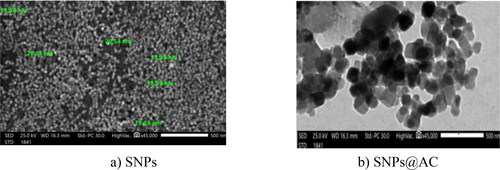
The particle size distribution of SNPs and the composite determined by intensity, showed two peaks around 10, and 80 nm attributed to particle size distribution of SNPs and the composite respectively. The increment between particle size distribution of SNPs and the composite reflects the successful loading of well dispersed SNPs on the AC biomass matrix.
3.2. The suggested photodeposition mechanism of silver nanoparticles on activated carbon matrix
Based on the principles of photochemistry and quantization of energy, the mechanism underlying the formation of SNPs@AC composite can be suggested as follows:
The quantized energy of UV–Vis. electromagnetic radiation light photon is absorbed by acetic acid (CH3COOH, reducing agent for AgNO3) containing active carbonyl (C = O) bond that easily undergoes heterolytic fission into two free radical species.
(5)
(5)
Acetic acid acts as a radical initiator. The slow step is the initiation of acetyl and hydroxyl free species radicals. Also, the photon energy causes photolysis of water molecules (solvent for silver nitrate) giving hydrogen and hydroxide radicals:
(6)
(6)
The formation of SNPs (Ag0) is attributed to the reduction of silver ion (Ag+) by the free radicals species either R· from acetic acid or (H·and OH·) from water.
(7)
(7)
The SNPs@AC showed good crystallinity, Figure S1, and has orthorhombic crystal system and P21 2121 space group, Table S1. SNPs are extensively loaded on AC matrix, Figure S2.
3.3. The bioactivity of SNPs@AC composite
The SNPs@AC composite sample exhibited excellent antibacterial activity against the tested bacterial pathogens both Gram-positive and Gram-negative bacteria as represented in . The inhibition zones of the bacterial growth on using the tested samples are collected in . Concentration 60 µg/mL inhibited all tested microbial pathogens except Bacillus subtilis (ATCC 6633) and Candida albicans (ATCC 10231). The most sensitive pathogens to the concentration 100 µg/mL are Enterococcus fecalis (ATCC 29212) and Pseudomonas aeruginosa (ATCC 9027). The inhibition zones relative to the control antibiotics showed a high potent performance of SNPs@AC composite than that is reported for SNPs [Citation13]. The SNPs@AC sample would be a promising candidate for developing antibacterial formulations for combating bacterial plant diseases and pathogens because its wide-spectrum antimicrobial activity more superior to many synthetic bactericides [Citation14,Citation15].
Figure 3. Antagonistic effects of different concentrations of SNPs@AC against Enterococcus fecalis (ATCC 29212).
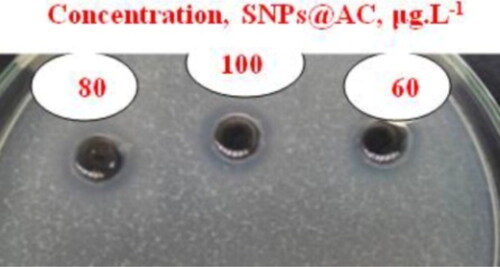
Table 1. Inhibition zone (mm) of 50 µL SNPs and SNPs@AC composite against some microbial pathogenic cultures.
The composite SNPs@AC is more active as an antibacterial agent but less active as an antifungal agent in comparison with SNPs. The relevant mechanism of biological activity of SNPs@AC composite showed potent antibacterial activity via inhibition of the main enzymes that are responsible for biosynthesis in the bacterial cell or linked to protein and lipid macromolecules constituted the cell wall. The SNPs may stimulate cytokine expression by intestinal epithelial cells [Citation7].
The anti-inflammatory activity of SNPs@AC composite sample is tested for in vivo denaturation of tissue proteins that is one of the well-documented cases of inflammatory and arthritic diseases. Production of auto antigens in certain arthritic diseases is due to the protein denaturation [Citation19]. Anti-inflammatory agents that inhibit protein denaturation would be worthwhile for the development of anti-inflammatory therapeutic drugs [Citation20].
The results presented in showed that concentrations: 100, 300, and 500 µg/mL SNPs@AC composite sample can protect the tested bovine serum albumin protein from denaturation with different percentages, and 500 µg/mL SNPs@AC suspension showed 75.72% protection efficiency against protein denaturation. Besides, the composite sample effectively reduced the inflammatory response in the eosinophilic chronic rhinosinusitis mouse model.
Figure 4. Anti-inflammatory activity of SNPs@AC sample represented in inhibition albumin–denaturation.
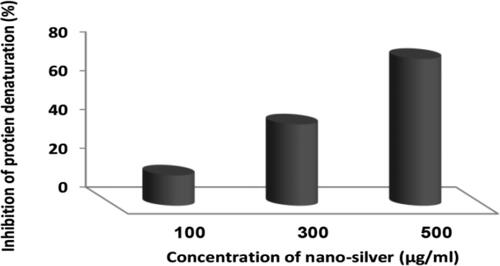
The cytotoxicity of SNPs@AC composite sample on VERO cell line (ATCC ccl-81), Vero cell was initiated in kidney tissue derived from a normal, adult African green monkey, certificate analysis explained that maximum nontoxic concentration (MNTC) equals 78.125 µg/mL that is used for antiviral assay against HAV corresponding to 96.666% antiviral activity HAV (; ). Hence, SNPs@AC composite has good preventative and therapeutic characteristics that effectively reduce viral infection [Citation21], probably by blocking the cell–virus interaction depends on the particle size and zeta potential [Citation9].
Table 2. Effect of composite sample against HAV on VERO cell line (ATCC ccl-81).
The physiological effect of SNPs@AC composite sample versus HAV on a sample of Vero cell line is shown in . showed an irregular outline, cytoplasmic projections, and cytoplasmic vacuolization in case of HAV-infected cells; while SNPs@AC composite sample showed that the nuclear membrane of HAV cells disintegrated, . Some nucleoli are difficult to be seen. The cytoplasm appears as mottled, lumps diffuse mass distributed throughout the cytosol, with intense lysosomes and myelin morphology.
Figure 5. Effect of (a): HAV, and (b) 78.125 µg/mL SNPs@AC composite on VERO cell line (ATCC ccl-81).
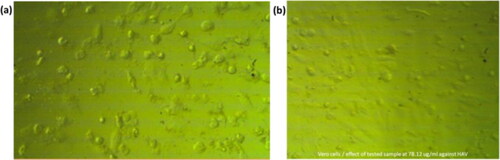
showed Pie plot of SNPs@AC antivirial activity. A law composite concentration 78.125 µg/mL inhibited HAV (10,000 cells/200 µL) by 96.666% percentage and HAV activity decreased to 3.33%.
Antiviral activity of SNPs@AC sample is evaluated, excluding all side effects on host VERO cells to clarify only the effect of safe concentration and subsequently nontoxic concentration to ensure virus viability because any toxic concentration of composite sample for VERO cells is unreasonable and inapplicable. Antiviral test is carried as follows: composite cytotoxicity on VERO cell line and then using MNTC to evaluate antiviral activity. The highest concentration of the tested sample cannot be used because it would be toxic to host cells. explained composite cytotoxicity on VERO cell line and safe concentration for antiviral activity.
The significant antioxidant potential of SNPs@AC composite is evaluated by diphenylpicryl hydrazine (DPPH) radical scavenging assay (IC50 201 µg/mL), while IC50 of reference vitamin C ascorbic acid is 14.2 µg/mL. The results confirmed that composite sample has antioxidant activity as compared to vitamin C.
showed that SNPs@AC composite sample exhibited significant 42.74% percentage antioxidant activity at 160 µg/mL concentration. This finding is in good agreement with the reported work about in vitro antioxidant activity of biosynthesized SNPs nanoparticles as DPPH radical scavenging (IC50 385.87 μg/mL) [Citation22]. The AC biomass matrix in agreement with algal biomass decrease IC50 of SNPs up to 201 µg/mL [Citation23].
Table 3. Antioxidant activity of SNPs@AC using DPPH radical scavenging assay.
3.4. Heterogeneous catalysis of SNPs@AC for hydrazination of 4-chloro-3, 5-dinitro-benzotrifluoride at 25 °C
Figures S3 and S4 confirmed that SNPs@AC composite sample is a highly porous solid material having large internal surface 626 m2/g enabled its application as heterogeneous catalyst [Citation3]. The reactive substrate compound: 4-chloro-3, 5-dinitro-benzo trifluoride reacts with hydrazine hydrate (NH2NH2) yielding the reaction product: 1-hydroxy-4-nitro-6-trifluoromethyl benzotriazole) antiviral aromatic compound, Scheme 1.
The reaction conditions are: water solvent (0%, 5% SNPs@AC composite, 0.02 M sodium dodecyl sulphate (SDS) and 298oK. This reaction is very useful in organic synthesis of chemotherapeutic antiviral benzotriazole compounds. The nitro (NO2) and hydroxyl (OH) group as well as 3N-triazoles heterocyclic ring are responsible for its antiviral activity. Chloro-nitro-trifluoro benzene (reactive starting compound) reacted with hydrazine (NH2–NH2) via aromatic nucleophilic substitution reactions, (SNAr), and addition–elimination (Ad–E) mechanism [Citation24]. SNAr may be a unimolecular substitution reaction (SN1) or bimolecular substitution reaction (SN2). The SN1 mechanism is ignored in hydrazination reaction because of extra strong phenyl –chloride bond. The Ad–E mechanism involved consecutive steps: dechlorination, nucleophilic attack, ring closure followed by dehydration. Hydrazine hydrate (NH2–NH2) is strong nucleophilic (Nu) due to the available lone pair of electrons on each nitrogen atom and nitro group at O-position facilitates hydrazination rate.
3.5. Kinetic data and the conversions of reactants into the product
SNPs@AC composite possessed (high porosity, Figure S3, surface area up to 626 m2 g−1, and thermally stable, Figure S5a,b. this highly porous and thermally stable composite catalyzed hydrazination reaction presented in Scheme 1. The steric hindrance in the reactant substrate is neglected due to small size of CF3 substituent group in para-position. The full curve for reaction product, is recorded at the start of the reaction, at λmax 700 nm, hence UV–Vis. absorbance of the reaction product is monitored at different time intervals at 700 nm, .
Figure 8. (a) Full curve for reaction product. (b) Representative curves for UV–Vis. absorption bands of the reaction product.
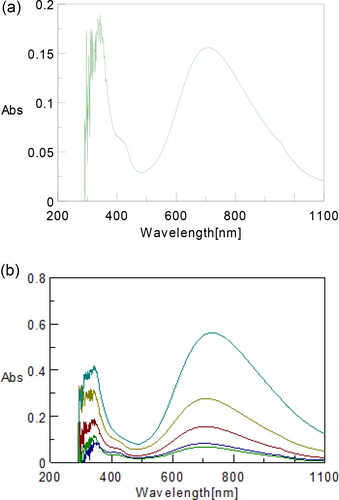
The reaction product is followed by UV–Vis. spectroscopy at 700 nm based on the full curves, .
For obtaining precious results, hydrazination reaction in Scheme 1 is carried out in duplicates runs at the same experimental conditions, where the absorbance of the reaction product (At) is recorded at different time intervals.
Fitting the kinetic data collected in Table S2 to the rate equation of first-order reaction, log(A∞–At) versus time for each kinetic run, is shown in in the absence and the presence of SNPS@AC composite, The plots: log(A∞–At) versus time give a straight line confirmed that the reaction follows pseudo-first-order kinetic as hydrazine concentration excess is in excess. Applying the least square method on the linear fitting of the straight line gave good correlation coefficient (R2) approach the unity, and kobserved (min.−1) for all reaction trails are listed in . Triplicates kinetic runs for catalyzed reaction by SNPs@AC composite showed that the presence of this composite in the reaction media increased the reaction rate.
Table 4. Pseudo first-order rate constant for the catalyzed and uncatalyzed hydrazination reaction.
The pseudo first rate constants kobs., min−1 of the reaction in the absence and the presence of SNPs@AC are measured under pseudo first-order rate conditions (excess hydrazine compared to that of the substrate, satisfying the molar ratio 10:1.
The observed rate constant (k, min.−1) for the catalyzed reaction in the triplicates kinetic runs showed nearly two folds increase compared to the rate constant of the unanalyzed reaction. This finding confirmed that catalysis by SNPs@AC composite enhanced the reaction that has the kinetic rate equation:
(8)
(8)
The SNPs@AC composite is a low-cost efficient catalyst that can remain unchanged for a long storage time due to its antibacterial activity, and act by lowering the activation energy of the reaction. The activated carbon is a catalyst support promoted the catalytic activity of SNPs. The catalytic activity of SNPs@AC or AgNPs composite for synthesis of 1-OH-4-NO2-6-trifluoromethyl benzotriazole starts by forming Ag-aryl species that is captured by NH2NH2 nucleophile molecules. The key intermediate is silver-hydride (Ag-H) generated by water absorption on AgNPs@AC without requiring a vacant coordination site on the composite surface.
The starting aryl chloride binding SNPs on AC solid support form Ag–C bond of catalytically active species (Ar–Ag) that further insert more NH2NH2 molecules. The catalytic active species Ar–Ag being regenerated by oxidative addition of Ar–Cl. Silver nanoparticles (Ag0) coordinated to aryl halide ArCl) forming coordinate-type bond, AgNPs is electron donor Lewis base and Ar–Cl is an electron acceptor Lewis acid. NH2NH2 nucleophile interacts with an electrophilic carbon atom on Ar–Cl.
The zero valent silver nanoparticle (Ag0) binding Ar–Cl giving organometallic (Ag0–Ar) complexes that are thermally stable due to the presence of fluoro atom at para position and absence of H atom in β position. Activated carbon is a conventional adsorbent [70], while AgNPs@AC is a nonconventional adsorbent but it is effective catalyst. For the above nucleophilic substitution reaction: addition of hydrazine (double nucleophile and removal of chloride ion leaving group occurs following mechanism of Zwitter ion and Meisenheimer complex (MC) depending on structural geometry of reactants and products. Bond length (C–Cl) reaction center, make hydrazination reaction followed second-order kinetics. Chloride abstraction occurs in rapid step, hydrazine attack ipso carbon carry chloride ion. In NO2 group, the nitrogen atom is more electron negative than the oxygen atom. This SNAr of the activated substrate occurs through addition–elimination or nucleophilic substitution mechanism (Ad–E, SNAr), Schemes 2 and 3.
In this nucleophilic aromatic substitution (SN Ar) reaction, the chloride atom is replaced by the neutral nucleophilic hydrazine molecule [Citation24].
Chloride ion leaving group created positively charged intermediates. The negatively charged composite SNPs@AC composite (zeta potential −25 mV) stabilized all the transition states encountered in the reaction mechanism [Citation7]. The reaction mechanism was controlled by the potential energy of: reactants, intermediates, transition states, and products.
Comparison of the rate constant, k1 revealed that the reaction rate in the presence of SNPs@AC is much rapid showed twofold increase than the same reaction in its absence. The composite SNPs@AC acted by decrease activation energy (Ea) of the reaction and stabilizing the positively charged transition states and the reactive intermediates.
Activation energy and activation parameters of the reaction are collected using Arrhenius, , and Eyring plot and using the collected data in about the absorbance for hydrazination reaction of 4-chloro-3,5-dinitro-benzotrifluoride at 30 °C, 35 °C, 40 °C, 45 °C in the presence of SNPs@AC composite.
Figure 9. Plot (A∞–At) vs. time for reaction: 4-chloro-3, 5-dinitro-benzotrifluoride and hydrazine at different temperatures.
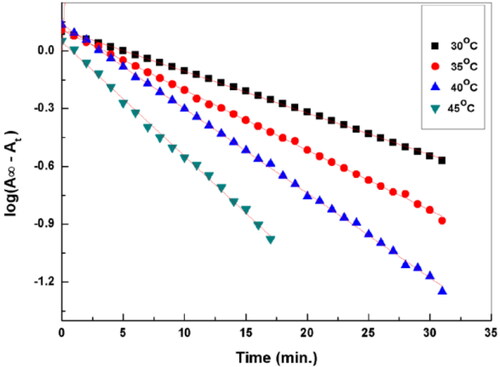
Figure 10. Arrhenius plot: lnk versus 1/T for reaction: 4-chloro-3, 5-dinitro-benzotrifluoride and hydrazine.
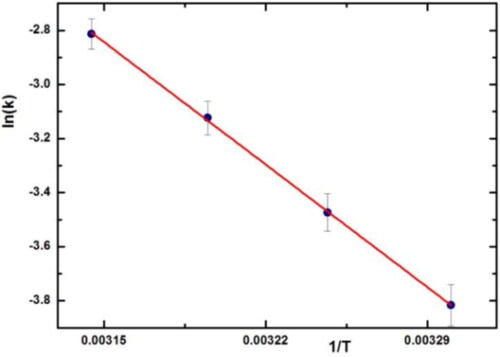
The reaction is carried out at a different temperature and activation parameter (ΔH#, ΔS# and ΔG# (are calculated using Eyring equation. Activation energy (Ea) of reaction is calculated using Arrhenius equation and all these parameters were collected in .
Table 5. Activation energy and activation parameters.
Entropy of activation, ΔS# is negative indicated high ordered reaction intermediates. The higher reaction rate in the presence of AgNPs@AC composite indicated that the porosity of the catalyst is not the key parameter in catalyzing the reaction. However, the high electron plasmon SNPs stabilized the reaction intermediate. The positive value energy of activation ( is attributed to the positive value of
and a comparison of the rate constants, k, revealed that the rate of hydrazination reaction is much greater than the same reaction in the absence of AgNPS@AC composite. The proposed reaction mechanism may be an addition–elimination mechanism (Ad–E, SNAr) involving formation of Zwitterionic and Meisenheimer complexes. Hydrazine (NH2–NH2) is the nucleophile, chloride ion (Cl–) is a leaving group, and AgNPs@AC catalyst stabilized Zwitterionic intermediate and represents solid support for the adsorbed reactants. Entropy of activation is positive consistent with a unimolecular mechanism. The enthalpy of activation (ΔH#), free energy change of activation (ΔG#) are quite equal. The negative ΔS# indicated possible hydrogen bonds formations between hydrazine hydrogen and the oxygen atom of o-NO2 group and the interaction of these atoms with the hydrogen atom of OH group as well as the transition state is more ordered than the starting reactants. The values ΔH#, and Ea are quite equal, but ΔG# is larger than Ea as ΔG# represented the heat converted into useful work during this organosynthesis reaction. The following factors affect the values of k1 for nucleophilic attack: ring activation values of k1 increase with increasing electron withdrawal group by ring substituents (CF3) at the para position which has no have serious steric effects. Also, no steric effects effect at the reaction center values due to the small steric size of the leaving group chloride ion (Cl–) [Citation24].
The scanning electron microscope (SEM) micrograph, of SNPs@AC composite showed adequate porosity of the composite for binding the reactants: 4-chloro-3, 5-dinitro-benzotrifluoride, and hydrazine to the active sites, forming the products which is then diffuse away from the surface of SNPs@AC composite into the bulk solution.
The absence fibrous structure of ultrathin channels is observed in the SEM micrograph image of SNPs@AC composite indicating that this composite catalyzed hydrazination reaction by porosity and electrostatic stabilization of the positively charged transition states and intermediates of the reaction.
4. Conclusion
Silver nanoparticles (SNPs)-activated carbon (AC) composite (SNPs@AC) is prepared by low-cost photodeposition method and showed high catalytic activity. This photochemical method produced pure, well-defined SNPs using convenience and simple equipment. In addition to the facile synthesis of SNPs colloidal dispersions in water under simple and mild conditions compared to preparation of SNPs with by chemical reduction. of silver ions in water yields ultra-small SNPs. Herein SNPs is an efficient eco-friendly catalyst for hydrazination of heterocyclic compound: 4-Cl-3, 5-di into benzotriflouride with in water 50% SNPs@AC at 25 °C to produce: 1-hydroxy-4-nitro-6-trifluoromethyl benzotriazole that is recommended: as antiviral reagent and precursors for many therapeutic formulations for defense against COVID-19. SNPs@AC composite material has shown superior biological activity against VERO cells than pure SNPs and exhibited antioxidant activity which established its great potential as an antioxidant agent. SNPs@AC were dispersed on the activated carbon of high surface area and the composite is well characterized using PXRD, SEM, EDX, TEM, TGA, and gas adsorption analysis.
In addition to their ease of separation, this SNPs@AC composite also demonstrated better selectivity, higher turnover frequency, and greater stability when compared to unsupported counterparts of SNPs. Comparison of the rate constants, k, revealed that the reaction rate in the presence of AgNPs@AC is much greater than the same uncatalyzed reaction. On investigation of the: reusability, activity, and stability of the solid SNPs@AC catalyst, it was washed with ethanol three times and reused in a new run, it showed high stability after 5 runs after reused for 12 cycles with a stable conversion of more than 99%. Also, SNPs@AC showed very high catalytic activity (about 97%) even after 12 cycles SNPs@AC composite insoluble in the reaction solutions and easily separated from the reaction solution by filtration. The SNPs@AC composite is a low-cost and efficient catalyst kept its catalytic activity for at least five cycles of reusing. The composite itself undergoes no change on storage for about one year due to its antibacterial activity, lowering the activation energy of the reaction and the activated carbon matrix promoted the catalytic activity of SNPs. Finally, the key points in this study are: The SNPs@AC composite would be useful as an antibacterial, antioxidant, and inflammatory agent; The SNPs@AC is an efficient catalyst for the synthesis the antiviral benzotriazole compound; the SNPs@AC composite would be easily prepared and commercialized.
Supplemental Material
Download MS Word (1.4 MB)Disclosure statement
There is no conflict of interest to declare.
Data availability statement
The data and materials that support the results or analyses presented in my paper are freely available upon request.
Correction Statement
This article has been republished with minor changes. These changes do not impact the academic content of the article.
References
- Hussain MS. Advances in nanoscience and nanotechnology Malaysia – an investment into the future. J Exp Nanosci. 2014;9(1):1–18.
- Daniel SK, Banu BN, Harshiny M, et al. Ipomea carnea-based silver nanoparticle synthesis for antibacterial activity against selected human pathogens. J Exp Nanosci. 2014;9(2):197–209. 1
- Gudikandula K, Charya Maringanti S. Synthesis of silver nanoparticles by chemical and biological methods and their antimicrobial properties. J Exp Nanosci. 2016;11(9):714–721.
- Praveena SM, Han LS, Than LT, et al. Preparation and characterisation of silver nanoparticle coated on cellulose paper: evaluation of their potential as antibacterial water filter. J Exp Nanosci. 2016;11(17):1307–1319.
- Phu DV, Lang VT, Kim Lan NT, et al. Synthesis and antimicrobial effects of colloidal silver nanoparticles in chitosan by γ-irradiation. J Exp Nanosci. 2010;5(2):169–179.
- Daoush WM, Hong SH. Synthesis of multi-walled carbon nanotube/silver nanocomposite powders by chemical reduction in aqueous solution. J Exp Nanosci. 2013;8(5):742–751.
- Sallam ER, Khairy HM, Elnouby MS, et al. Sustainable electricity production from seawater using spirulina platensis microbial fuel cell catalyzed by silver nanoparticles-activated carbon composite prepared by a new modified photolysis method. Biomass Bioenergy. 2021;148:106038..
- Venkatesan P, Santhanalakshmi J. Synthesis, characterisation and catalytic activity of gold and silver nanoparticles in the bio-sensor application. J Exp Nanosci. 2014;9(3):293–298.
- Ahmadi S, Fazilati M, Mousavi SM, et al. Anti-bacterial/fungal and anti-cancer performance of green synthesized Ag nanoparticles using summer savory extract. J Exp Nanosci. 2020;15(1):363–380.
- Santhoshkumar R, Hima Parvathy A, Soniya EV. Phytosynthesis of silver nanoparticles from aqueous leaf extracts of Piper colubrinum: characterisation and catalytic activity. J Exp Nanosci. 2021;16(1):294–308.
- Fetouh HA, Hefnawy A, Attia AM, et al. Facile and low-cost green synthesis of eco-friendly chitosan-silver nanocomposite as novel and promising corrosion inhibitor for mild steel in chilled water circuits. J Mol Liq. 2020;319:114355.
- Verschueren KHG, Pumpor K, Anemüller S, et al. A structural view of the inactivation of the SARS coronavirus main proteinase by benzotriazole esters. Chem Biol. 2008;15(6):597–606.
- Wu C-Y, King K-Y, Kuo C-J, et al. Stable benzotriazole esters as mechanism-based inactivators of the severe acute respiratory syndrome 3CL protease. Chem Biol. 2006;13(3):261–268.
- Alhazzani W, Møller MH, Arabi YM, et al. Surviving sepsis campaign: guidelines on the management of critically ill adults with coronavirus disease 2019 (COVID-19). Intensive Care Med. 2020 Mar 28;46(5):854–834.
- Amin SA, Banerjee S, Ghosh K, et al. Protease targeted COVID-19 drug discovery and its challenges: insight into viral main protease (mpro) and papain-like protease (PLpro) inhibitors. Bioorg Med Chem. 2021;29:115860.
- Dyall J, Gross R, Kindrachuk J, et al. Middle east respiratory syndrome and severe acute respiratory syndrome: current therapeutic options and potential targets for novel therapies. Drugs. 2017;77(18):1935–1966.
- Hiba K, Sreekumar K. Multi-arm dendronized polymer as a unimolecular micelle: synthesis, characterization and application as organocatalyst in the synthesis of N-unsubstituted 1, 2, 3-triazoles. React Funct Polym. 2021;160:104827.
- Cai WF, Pu KB, Ma Q, et al. Insight into the fabrication and perspective of dendritic Ag nanostructures. J Exp Nano-Sci. 2017;12(1):319–337.
- Saleem A, Saleem M, Akhtar MF. Antioxidant, anti-inflammatory and antiarthritic potential of Moringa oleifera lam: an ethnomedicinal plant of Moringaceae family. S Afr J Bot. 2020;128:246–256.
- Chandra S, Chatterjee P, Dey P, et al. Evaluation of in vitro anti-inflammatory activity of coffee against the denaturation of protein. Asian Pacific J Trop Biomed. 2012;2(1):S178–S180.
- Gaikwad S, Ingle A, Gade A, et al. Antiviral activity of mycosynthesized silver nanoparticles against herpes simplex virus and human parainfluenza virus type 3. Int J Nanomed. 2013;8:4303–4314.
- Ravichandran S, Paluri V, Kumar G, et al. A novel approach for the biosynthesis of silver oxide nanoparticles using aqueous leaf extract of callistemon lanceolatus (myrtaceae) and their therapeutic potential. J Exp Nanosci. 2016;11(6):445–458.
- Massironi A, Morelli A, Grassi L, et al. Ulvan as novel reducing and stabilizing agent from renewable algal biomass: application to green synthesis of silver nanoparticles. Carbohydr Polym. 2019;203:310–321.
- Fathalla MF, Elmarassi YR, Omer OF, et al. The reaction of 2-chloroquinoxaline with piperidine in DMSO–H2O and DMF–H2O mixtures: kinetics and solvent effects. J Sol Chem. 2019;48(8–9):1287–1308.