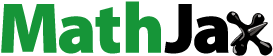
Abstract
In this study, copper nanoparticles were synthesised according to green chemistry rules using the aqueous extract of Alhagi maurorum. The green-synthesised CuNPs were characterised using different techniques such as EDX, FE-SEM, XRD and FT-IR. The FE-SEM results confirm spherical morphology for the nanoparticles with a size of 42.68–68.87 nm. In vivo, the Mycoplasma pneumonia injection was applied for inducing pneumonia in the BALB/c mice, also the treatment was with CuNPs. The serum parameters such as inflammatory mediators (IL-6, TNF-α, IL-8, TGF and IL-1), total cell counts, and total protein content levels were evaluated. CuNPs regulated the levels of the inflammatory mediators in the infected mice. The cellular arrangements at the histopathological images were ameliorated with the administration of CuNPs. At the antioxidant test, the CuNPs and BHT removed 50% of free radicals at the concentrations of 181 and 110 µg/ml. In conclusion, the results revealed the ameliorative property of CuNPs green-formulated by A. maurorum aqueous extract on M. pneumoniae infected pneumonia mice model.
1. Introduction
Pneumonia is a lung disease with high clinical signs. Many non-infectious causes, including food aspiration, hydrocarbons, foreign bodies, stomach acid, and allergic reactions, can also cause pneumonia [Citation1]. Pneumonia is the most usual death cause in children around the world, especially in developing countries, and causes about 4 million deaths among children annually. The annual prevalence of pneumonia in developing countries is 0.29% [Citation1–4]. Annually, 151.6 million new cases and 12–20 million (7–13%) of severe cases in need of hospitalisation are reported. The most common bacterial germ of all ages is Streptococcus pneumonia [Citation5–7]. Risk factors such as poverty, low family literacy, low birth weight, malnutrition, and non-breastfeeding in the development of pneumonia in developing countries have been described [Citation7, Citation8]. Environmental factors that increase the risk of developing pneumonia include going to kindergarten, smoking, exposure to secondhand smoke, and large family populations. One of the bacterial species that causes severe pneumonia is Mycoplasma pneumonia [Citation9–11]. Mycoplasmas are the smallest and simplest release objects known. These bacteria are probably derived from gram-positive bacteria and are phylogenetically related to Clostridia. Their genome is small and consists of a double-stranded cyclic dioxyribonucleic acid molecule containing 500 to 1000 genes [Citation11–13]. M. pneumonia, unlike other species of Mycoplasma, is not present as part of the natural flora, and out of ten accepted human species of the genus Mycoplasma, only this species has been proven to cause disease. This bacterium is one of the causes of lower and upper respiratory tract infections and its clinical picture is progressive as slow tracheobronchitis, with restlessness and dry cough [Citation12–14]. The pathogenicity of this bacterium ranges from mild forms of pharyngitis and tracheobronchitis to cases of acute pneumonia. A wide range of extra-respiratory manifestations such as hematologic, gastrointestinal, renal, musculoskeletal, cardiovascular, immunological, dermatological, and neurological complications have been reported in connection with this bacterium [Citation11–15]. Currently, culture, serological detection methods, and nucleic acid amplification techniques are three available methods for routine diagnosis of M. pneumonia infections. Culture-based methods are time-consuming techniques with relatively low sensitivity and require facilities as well as sufficient experience to interpret the results [Citation11–13]. Nowadays, diagnostic methods based on polymerase chain reaction are considered suitable methods for diagnosing factors such as M. pneumonia due to their sensitivity, specificity, and accuracy [Citation12–14]. Recent studies have reported that the metallic NPs green-mediated has significant antimicrobial effects against pathogenic microbes [Citation15–20].
Camelthorn with the scientific name of Alhagi maurorum is a plant of the Fabaceae family. Camelthorn grows in different places around the world from North Africa to Europe and Asia [Citation21, Citation22]. The plant is known as an herbal medicine with a wide variety of applications in folklore medicine such as laxative, diuretic, and expectorant properties [Citation23]. Camelthorn is known as an effective remedy for reducing acidity in the gastric problem [Citation24]. A. maurorum is used to heal wounds and also as an agent for diaphoretic and antiseptic [Citation25]. A. maurorum essential oil is an effective remedy for rheumatism [Citation26]. Plants' secondary metabolites play a basic role in herbal medicinal uses, according to earlier studies, Camelthorn is rich in different classes of compounds such as phenolic, alkaloids, flavonoids, fatty acids, and vitamins. Certainly, the existence of various compounds in the plant is the essential factor for the wide variety of pharmaceutical uses of the plant [Citation24, Citation27, Citation28]
In this project, copper NPs were formulated according to pharmacology rules by A. maurorum aqueous extract to treat the mouse model of M. pneumonia.
2. Methods and materials
2.1. Preparation and extraction of aqueous extract
At the first step, 100 g of A. maurorum grounded leaves were boiled in 1000 ml for 10 min. Then, the extract was filtrated and concerted using a rotary evaporator. Next, the concerted extract was dried using a freeze drier. The obtained extract was a powder brown colour and hold in a refrigerator.
2.2. Green synthesis and chemical characterisation of CuNPs
The CuNPs was synthesised using a reported assay [Citation29, Citation30]. First, 50 ml of the plant extract (with a concentration of 0.01 g/L) was added to 50 ml of Cu(NO3)2·3H2O (0.3 M). Next, the mixture was stirred at 70 °C over a night. A dark brown participation (CuNPs) was formed during the time. Deionised water and ethanol were used to wash CuNPs three times. The final residue was centrifuged at 14,000 rpm for 12 min. In the end, the precipitate was put in an oven at 55 °C to dry.
2.3. Antioxidant activities determination of CuNPs
1,1-Diphenyl-2-picryl-hydrazyl (DPPH) was performed to assess the nanoparticles' antioxidant activities. 2 ml of 0.2 mM DPPH solution was mixed by 5 ml of NPs suspension at dilations of 1–1000 μg/ml. The incubation was done for 30 min at room temperature and absorbed at 517 nm in the spectrophotometer. The free radical adsorption percentage was calculated by the below formula so that A0 was the control sample adsorption and A1 was the desired sample adsorbent [Citation19, Citation20]:
2.4. In vivo design
The animals (n = 24) were divided following groups:
Normal control.
Pneumonia infection control: Infected with M. pneumonia solution.
T1: Infected with M. pneumonia solution + Cu NPs (50 μg/kg).
T2: Infected with M. pneumonia solution + Azithromycin (AZM), 200 mg/kg.
After 3 days, the chloroform was used for anaesthetising the mice. By tracheal cannulation of PBS into the right side lingual or middle lobe thrice, the BALF was gained, and immediately it was centrifuged at 8000 rpm for 15 min to remove the debris. The total cell numbers of BALF were gained by hemocytometer. The total protein level was determined by the Bradford technique and protein assay kit. The BALF inflammatory cytokines levels such as IL-6, TGF, IL-8, TNF-α and IL-1 were inspected by the ELISA technique. The eosin and hematoxylin staining was performed to stain the lung sections, and then the histopathological changes were investigated under light microscopy [Citation31].
The data were assessed by SPSS ver22 statistical software and a one-way ANOVA test. p < 0.01 was considered remarkable.
3. Results and discussion
The XRD diagram of CuNPs is presented in . XRD is an effective method to study nanoparticle crystallinity. The results approve the formation of CuNPs in small size and well crystallising. The different signals at 2θ values were compared with the standard database of PDF card No. 04-012-7238. The signals at 32.40, 35.48, 38.65, 48.75, 58.19, 61.27, 68.07 and 74.88 are indexed as (110), (11-1), (111), (20-2), (202), (−113), (221) and (22-2). The data are matched to those of copper oxide signals [Citation30]. The crystal size of CuNPs was obtained using Scherer’s equation with an amount of 31.21 nm. According to previous reports on the green synthesis of CuNPs, the nanoparticles were in the range size of 12–40 nm [Citation32–36].
The elemental study of CuNPs was evaluated using EDX analysis. The EDX diagram of CuNPs is presented in . The successful synthesis of copper nanoparticles is approved by the signals that belong to the copper element (0.95 Kev, CuLα; 8.1 Kev, Cu Kα, 8.8 Kev, Cu Kβ) [Citation37]. The presence of oxygen is confirmed by the peak around 0.5 keV (OLα) which corroborates the formation of copper oxide, this is in agreement with previous studies on the formation of copper oxide in the green synthesis of CuNPs [Citation38]. Another signal at 0.20 keV belongs to CLα that exhibits the presence of carbon in CuNPs. The carbon and oxygen signals are correlated to the secondary metabolites of A. maurorum that are bound to the CuNPs surface.
The morphology investigation of CuNPs was carried out using the FE-SEM technique. presents the FE-SEM images of CuNPs. The result shows that CuNPs are formed in spherical morphology with 42.68–68.87 nm for particle size. CuNPs are also aggregated which is a common characteristic for synthetic metallic nanoparticles using green approaches [Citation32, Citation39–43]. In the literature, the range size of 5–100 nm has been reported for the green-synthesised copper nanoparticles [Citation44–47].
The FT-IR spectra of A. maurorum extract and CuNPs are exhibited in . The bands at the wavenumbers of 444 and 609 cm−1 belong to Cu–O approving the formation of CuNPs as copper oxide. This is in agreement with EDX and XRD results. Cheirmadurai et al have reported the bands for green synthetic of CuNPs with a little difference in wavenumber [Citation30, Citation48]. Furthermore, the peaks at 3423 and 2928 cm−1 for O–H and C–H, 1453 to 1679 cm−1 for C = C and C = O, and 1057 cm−1 for –C–O belong to the different functional groups of A. maurorum secondary metabolites that play the reducing and capping role to CuNPs synthesis. These constituents include different classes of compounds including phenols, flavonoids, and triterpenes, which were found as A. maurorum secondary metabolites previously [Citation49, Citation50]. The FT-IR spectra of the plant extract and CuNPs are similar together approves the linkage of secondary metabolites of the plant extract to the CuNPs surface.
Extraction from biological organisms can be done both as capping and reducing agents in the metal nanoparticle synthesis. Metal ions reduction combined with biomolecules present in cellular extracts such as vitamins, polysaccharides, amino acids, proteins, and enzymes is environmentally friendly, whereas synthesis with chemicals is not [Citation16–19]. Extensive volumes of reports indicate that the synthesis of metal nanoparticles using bio-organic compounds is successful. Various proteins, enzymes, secondary metabolites, or other capping and reducing agents have a unique role in nanoparticle production by plants [Citation19–21, Citation42]. Recovery of nanoparticles from plant tissue is expensive and tedious and needs enzymes to destroy plant cellulose tissue [Citation44, Citation45]. Therefore, it is so easier to use extracts of plants in large and small-scale processing to prepare several metal nanoparticles. Recently, researchers used plant extracts rather than physical and chemical methods to synthesise the metal nanoparticles [Citation42–45]. The extract proteins make the dual function of shape control and metal reduction in the metal nanoparticle synthesis [Citation17–19]. Carboxyl groups in aspartic residues, glutamine, and hydroxy groups in tyrosine residues in proteins have been suggested as metal ion reducing agents [Citation42, Citation45]. The reduction process is performed by a simple dual-function tripeptide that is mostly identified in the amino acid residue. This synthesis process produces small metal nanoplates with very low dispersion [Citation44, Citation45]. Green-synthesizing the nanoparticles by plants increase their antioxidant properties of them in comparison to other methods [Citation16–20].
In the current experiment, the effects of BHT and Cu NPs on the common free radicals (DPPH) were evaluated (). In the free radical examination, the IC50 of Cu NPs and BHT against DPPH were 181 and 110 µg/ml, respectively.
Today, bacterial resistance has caused problems in the treatment of patients suffering from Mycoplasma infections. So that the antibiotics used in the common treatments of urinary infection gradually lose their effectiveness [Citation51–53]. Nanoparticles have unique physical, chemical, optical and biological properties, which are good candidates for use inside the body and in laboratory research due to their small size [Citation54–56]. Among their uses, it can be mentioned as a substitute for antibiotics or together with them to treat drug-resistant bacteria. Copper nanoparticles have a spherical and crystalline appearance and are used in various industries. The shape and size of nanoparticles are important for their activity so that smaller particles have stronger and more antimicrobial activity than larger particles [Citation53–57]. These nanoparticles have antibacterial activity against drug-resistant isolated clinical samples, including gram-positive bacteria. The size of these particles is approximately three to one hundred nanometres and they have been used to detect proteins and microorganisms and design biosensors [Citation51–53]. Due to the wide application of copper nanoparticles in the treatment of diseases, few reports have been reported on their side effects on living cells and tissues in the body and cases with contradictory results [Citation52–56]. In general, today, excessive use of common antibiotics has increased antibiotic resistance among Mycoplasma pathogens. Therefore, due to the wide application of copper nanoparticles in the treatment of diseases, little information is available on their effect on gram-negative bacilli that cause Mycoplasma infections [Citation54–57].
In a recent study, the levels of inflammatory cytokines and cells and total protein increased due to the M. pneumonia infection. The histopathological findings indicated increasing the levels of the lymphocytes and plasmocytes of the lung due to the immune responses against the infection ().
Administration of the CuNPs regulated the levels of inflammatory cytokines and cells and total protein in serum and reduced the levels of the lymphocytes and plasmocytes of the lung in the lung ().
4. Conclusion
In conclusion, the aqueous extract of A. maurorum was used as the reducing agent to synthesise CuNPs. The common chemical characterisation methods such as FT-IR, XRD, FE-SEM and EDX were used to chemical and physical properties of copper nanoparticles. The obtained results confirmed the synthesis of copper oxide nanoparticles with spherical morphology and with a particle e range size of 42.68–68.87 nm. At the free radical examination, CuNPs and BHT removed 50% of free radicals at concentrations of 181 and 110 µg/ml. The cellular arrangements at the histopathological images were ameliorated with the administration of CuNPs. CuNPs regulated the levels of the inflammatory mediators in the infected mice.
Disclosure Statement
No potential conflict of interest was reported by the author(s).
Data availability statement
Data is available on request from the authors.
Additional information
Notes on contributors
Tian Liyuan
Tian Liyuan was appointed as the director of area A of the Respiratory of Hebei Children's Hospital, has completed his Master's in the University of Milano Bicocca in Italy and Hebei Medical University. He is the leader of Futang Medical Development Center of Beijing Children's Hospital Group and the respiratory professional discipline leader of Hebei Children's Hospital, and serves as the vice president and member of many medical societies in Hebei Province. He has Published a number of SCI papers, domestic core journal papers, the paper “gestational age, weight and hypoxia on neonatal fibrinolytic activity” won the third prize of the Provincial Department of Science and Technology.
Zhang Lijun
Zhang Lijun is an attending physician working in the Hebei Children's Hospital. Her research mainly focuses on the diagnosis and treatment of severe respiratory diseases in children.
Hou Wei
Hou Wei is an attending physician working in the Hebei Children's Hospital.Good at the diagnosis and treatment of severe respiratory diseases in children, the main research direction of children viral pneumonia and fungal pneumonia.
Jia Meixuan
Jia Meixuan has completed his Master's in Shanxi Medical University and is an attending docter, currently working in the Hebei Provincial Children's Hospital. His research interests include Mycoplasma pneumoniae pneumonia and respiratory tract related allergic diseases.
Zhang Man
Zhang Man is a senior attending physician in area A of the Respiratory Department of Hebei Children's Hospital and has been engaged in pediatric clinical work for 17 years. She has accumulated rich experience in the diagnosis and treatment of pediatric diseases, and is often invited to consult in other departments. He is especially good at the diagnosis and treatment of children's recurrent cough, pneumonia, bronchiolitis, bronchial asthma, cough variant asthma, interstitial lung diseases and respiratory diseases. And she has participated in a number of provincial projects and a number of GCP projects.
Yan Zhihui
Yan Zhihui has completed her Master's degree in internal medicine from the Research Institute of Hebei Medical University, and she is currently working in the Respiratory Department of Hebei Children's Hospital. Her main research directions are respiratory syncytial virus infection and children's bronchial asthma.
Li Qingtao
Li Qingtao, Deputy Chief Physician, Hebei Children's Hospital, Member of Hebei Pediatric Society, Member of Expert Committee of China Children's Asthma Action Plan, Member of Hebei Provincial Association of Integrated Traditional Chinese and Western Medicine Pediatric Cardiovascular Society. Focus on the diagnosis and treatment of common diseases, frequently-occurring diseases and critical illnesses of children's respiratory system, and skilled in the technology of fiberoptic bronchoscopy.
Qian Xiaona
Qian Xiaona, a master's degree and resident, graduated from Hebei Medical University and is good at frequent respiratory diseases and common diseases in children.
Wang Qian
Wang Qian, master of clinical medicine, resident doctor of Hebei Province Children's Hospital, focus on the diagnosis and treatment of common diseases and frequently-occurring diseases of children's respiratory system.
Tian Jiangling
Tian Jiangling is a resident at Hebei Children's Hospital and has completed her Master's in Hebei Medical University and is good at the diagnosis and treatment of serious respiratory diseases in children.
You Dianping
You Dianping was appointed as the secretary of the party committee of Hebei Children's Hospital, and was awarded the title of outstanding contribution worker of the National Health Commission to improve medical service, winner of Hebei Provincial Government quality Award (individual Award) and Hebei Province advanced individual fighting against COVID-19 epidemic situation. He served as the president or vice president of many societies, associations, councils and medical federations in Hebei Province, mainly engaged in social medicine and health management. He has published many articles and won the second prize of science and technology in Hebei Province.
Wang Yakun
Wang Yakun is a deputy chief physician in the Children's Hospital of Hebei Provence, in the Department of Respiratory. Her area of interest includes the diagnosis and treatment of respiratory diseases in children. She also specializes in fibroptic bronchoscopy.
References
- Hausner M, Schamberger A, Naumann W, et al. Development of protective anti-Mycoplasma pneumonia antibodies after immunization of guinea pigs with the combination of a P1-P30 chimeric recombinant protein and chitosan. Microb Pathog. 2013;64:23–32.
- Zhu Z, Xu W, Abernathy ES, et al. Comparison of four methods using throat swabs to confirm rubella virus infection. J Clin Microbiol. 2007;45(9):2847–2852.
- Yamazaki T, Narita M, Sasaki N, et al. Comparison of PCR for sputum samples obtained by induced cough and serological tests for diagnosis of mycoplasma pneumonia infection in children. Clin Vaccine Immunol. 2006;13(6):708–710.
- Pitcher D, Chalker VJ, Sheppard C, et al. Real-time detection of mycoplasma pneumonia in respiratory samples with an internal processing control. J Med Microbiol. 2006;55(Pt 2):149–155.
- Saharan P, Dhingolia S, Khatri P, et al. Loop-mediated isothermal amplification (LAMP) based detection of bacteria: a review. Afr J Biotechnol. 2014;13(19):1920–1928.
- Buist AS, McBurnie MA, Vollmer WM, et al. International variation in the prevalence of COPD (the BOLD study): a population-based prevalence study. Lancet. 2007;370(9589):741–750.
- Barker BL, Haldar K, Patel H, et al. Association between pathogens detected using quantitative polymerase chain reaction with airway inflammation in COPD at stable state and exacerbations. Chest. 2015;147(1):46–55.
- Marin A, Monso E, Garcia-Nunez M, et al. Variability and effects of bronchial colonization in patients with moderate COPD. Eur Respir J. 2010;35(2):295–302.
- Fricker M, Deane A, Hansbro PM. Animal models of chronic obstructive pulmonary disease. Expert Opin Drug Discov. 2014;9(6):629–645.
- Bebear C, Pereyre S, Peuchant O. Mycoplasma pneumonia: susceptibility and resistance to antibiotics. Future Microbiol. 2011;6(4):423–431.
- Bebear C, Raherison C, Nacka F, et al. Comparison of mycoplasma pneumonia infections in asthmatic children versus asthmatic adults. Pediatr Infect Dis J. 2014;33(3):e71–e5.
- Becker A, Kannan TR, Taylor AB, et al. Structure of CARDS toxin, a unique ADP-ribosylating and vacuolating cytotoxin from mycoplasma pneumonia. Proc Natl Acad Sci USA. 2015;112(16):5165–5170.
- Daxboeck F, Blacky A, Seidl R, et al. Diagnosis, treatment, and prognosis of mycoplasma pneumonia childhood encephalitis: systematic reviewof58cases. J Child Neurol. 2004;19(11):865–871.
- Domenech C, Leveque N, Lina B, et al. Role of mycoplasma pneumonia in pediatric encephalitis. Eur J Clin Microbiol Infect Dis. 2009;28(1):91–94.
- Dorigo-Zetsma JW, Wilbrink B, Nat H, et al. Results of molecular detection of mycoplasma pneumonia among patients with acute respiratory infection and in their household contacts reveals children as human reservoirs. J Infect Dis. 2001;183(4):675–678.
- Hemmati S, Rashtiani A, Zangeneh MM, et al. Green synthesis and characterization of silver nanoparticles using fritillaria flower extract and their antibacterial activity against some human pathogens. Polyhedron. 2019;158:8–14.
- Seydi N, Mahdavi B, Paydarfard S, et al. Preparation, characterization, and assessment of cytotoxicity, antioxidant, antibacterial, antifungal, and cutaneous wound healing properties of titanium nanoparticles using aqueous extract of Ziziphora clinopodioides Lam leaves. Appl Organometal Chem. 2019;33(9):e5009.
- Zhaleh M, Zangeneh A, Goorani S, et al. In vitro and in vivo evaluation of cytotoxicity, antioxidant, antibacterial, antifungal, and cutaneous wound healing properties of gold nanoparticles produced via a green chemistry synthesis using Gundelia tournefortii L. as a capping and reducing agent. Appl Organometal Chem. 2019;33(9):e5015.
- Zangeneh MM, Ghaneialvar H, Akbaribazm M, et al. Novel synthesis of falcaria vulgaris leaf extract conjugated copper nanoparticles with potent cytotoxicity, antioxidant, antifungal, antibacterial, and cutaneous wound healing activities under in vitro and in vivo condition. J Photochem Photobiol B Biol. 2019;197:111556.
- Zangeneh MM, Joshani Z, Zangeneh A, et al. Stachys lavandulifolia flower, and their cytotoxicity, antioxidant, antibacterial and cutaneous wound‐healing properties. Appl Organometal Chem. 2019;33(9):e5016.
- Hamed A, Perrone A, Mahalel U, et al. Oleanane glycosides from the roots of alhagi maurorum. Phytochem Lett. 2012;5(4):782–787.
- M. Al-Saleem MS, Al-Wahaib LH, Abdel-Mageed WM, et al. Antioxidant flavonoids from alhagi maurorum with hepatoprotective effect. Phcog Mag. 2019;15(65):592.
- Akbar S. Handbook of 200 medicinal plants: a comprehensive review of their traditional medical uses and scientific justifications. 2020. Cham, Switzerland: Spriger.
- Olas B, Hamed AI, Oleszek W, et al. Comparison of biological activity of phenolic fraction from roots of alhagi maurorum with properties of commercial phenolic extracts and resveratrol. Platelets. 2015;26(8):788–794.
- Ahmad N, Shinwari ZK, Hussain J, et al. Phytochemicals, antibacterial and antioxidative investigations of alhagi maurorum medik. Pak J Bot. 2015;47:121–124.
- Laghari AH, Ali Memon A, Memon S, et al. Determination of free phenolic acids and antioxidant capacity of methanolic extracts obtained from leaves and flowers of camel thorn (alhagi maurorum). Nat Prod Res. 2012;26(2):173–176.
- Awaad Amani A, Maitland D, Soliman G. Antiulcerogenic activity of alhagi maurorum. Pharm Biol. 2006;44(4):292–296.
- Laghari AH, Memon S, Nelofar A, et al. Alhagi maurorum: a convenient source of lupeol. Ind Crops Prod. 2011;34(1):1141–1145.
- Kumar PV, Shameem U, Kollu P, et al. Green synthesis of copper oxide nanoparticles using aloe vera leaf extract and its antibacterial activity against fish bacterial pathogens. BioNanoSci. 2015;5(3):135–139.
- Liu H, Wang G, Liu J, et al. Green synthesis of copper nanoparticles using cinnamomum zelanicum extract and its applications as a highly efficient antioxidant and anti-human lung carcinoma. J Exp Nanosci. 2021;16(1):410–423.
- Liu R, Ma SG, Liu YX, et al. Albizosides D and E, two new cytotoxic triterpenesaponins from albiziachinensis. Carbohydr Res. 2010;345(13):1877–1881.
- Ghidan AY, Al-Antary TM, Awwad AM. Green synthesis of copper oxide nanoparticles using punica granatum peels extract: Effect on green peach aphid. Environ Nanotechnol Monit Manag. 2016;6:95–98.
- Rajesh KM, Ajitha B, Reddy YAK, et al. Assisted green synthesis of copper nanoparticles using Syzygium aromaticum bud extract: Physical, optical and antimicrobial properties. Optik. 2018;154:593–600.
- Ijaz F, Shahid S, Khan SA, et al. Green synthesis of copper oxide nanoparticles using abutilon indicum leaf extract: Antimicrobial, antioxidant and photocatalytic dye degradation activitie. Trop J Pharm Res. 2017;16(4):743–753.
- Sorbiun M, Mehr ES, Ramazani A, et al. Green synthesis of zinc oxide and copper oxide nanoparticles using aqueous extract of oak fruit hull (jaft) and comparing their photocatalytic degradation of basic violet 3. Int J Environ Res. 2018;12(1):29–37.
- Gu J, Aidy A, Goorani S. Anti-human lung adenocarcinoma, cytotoxicity, and antioxidant potentials of copper nanoparticles green-synthesized by calendula officinalis. J Exp Nanosci. 2022;17(1):285–296.
- Sebeia N, Jabli M, Ghith A. Biological synthesis of copper nanoparticles, using nerium oleander leaves extract: characterization and study of their interaction with organic dyes. Inorg Chem Commun. 2019;105:36–46.
- Punniyakotti P, Panneerselvam P, Perumal D, et al. Anti-bacterial and anti-biofilm properties of green synthesized copper nanoparticles from cardiospermum halicacabum leaf extract. Bioprocess Biosyst Eng. 2020;43(9):1649–1657.
- Mahdavi B, Paydarfard S, Rezaei‐Seresht E, et al. Green synthesis of NiONPs using trigonella subenervis extract and its applications as a highly efficient electrochemical sensor, catalyst, and antibacterial agent. Appl Organomet Chem. 2021;35(8):e6264.
- Mahdavi B, Paydarfard S, Zangeneh MM, et al. Assessment of antioxidant, cytotoxicity, antibacterial, antifungal, and cutaneous wound healing activities of green synthesized manganese nanoparticles using ziziphora clinopodioides lam leaves under in vitro and in vivo condition. Appl Organometal Chem. 2020;34(1):e5248.
- Mahdavi B, Saneei S, Qorbani M, et al. Ziziphora clinopodioides lam leaves aqueous extract mediated synthesis of zinc nanoparticles and their antibacterial, antifungal, cytotoxicity, antioxidant, and cutaneous wound healing properties under in vitro and in vivo conditions. Appl Organometal Chem. 2019;33(11):e5164.
- Baghayeri M, Mahdavi B, Hosseinpor‐Mohsen Abadi Z, et al. Green synthesis of silver nanoparticles using water extract of salvia leriifolia: Antibacterial studies and applications as catalysts in the electrochemical detection of nitrite. Appl Organometal Chem. 2018;32(2):e4057.
- Mahendran D, Kavi Kishor PB, Geetha N, et al. Efficient antibacterial/biofilm, anti-cancer and photocatalytic potential of titanium dioxide nanocatalysts green synthesised using gloriosa superba rhizome extract. J Exp Nanosci. 2021; 16(1):11–30.
- Vaidehi D, Bhuvaneshwari V, Bharathi D, et al. Antibacterial and photocatalytic activity of copper oxide nanoparticles synthesized using solanum lycopersicum leaf extract. Mater Res Express. 2018;5(8):085403.
- Khani R, Roostaei B, Bagherzade G, et al. Green synthesis of copper nanoparticles by fruit extract of ziziphus spina-christi (L.) willd.: application for adsorption of triphenylmethane dye and antibacterial assay. J Mol Liq. 2018;255:541–549.
- Sulaiman GM, Tawfeeq AT, Jaaffer MD. Biogenic synthesis of copper oxide nanoparticles using olea europaea leaf extract and evaluation of their toxicity activities: an in vivo and in vitro study. Biotechnol Prog. 2018;34(1):218–230.
- Kaur P, Thakur R, Chaudhury A. Biogenesis of copper nanoparticles using peel extract of punica granatum and their antimicrobial activity against opportunistic pathogens. Green Chem Lett Rev. 2016;9(1):33–38.
- Cheirmadurai K, Biswas S, Murali R, et al. Green synthesis of copper nanoparticles and conducting nanobiocomposites using plant and animal sources. RSC Adv. 2014;4(37):19507–19511.
- Yuan C, Jiang B, Xu X, et al. Anti-human ovarian cancer and cytotoxicity effects of nickel nanoparticles green-synthesized by alhagi maurorum leaf aqueous extract. J Exp Nanosci. 2022;17(1):113–125. (2020)
- Bai J, Gongsun X, Xue L, et al. Introducing a modern chemotherapeutic drug formulated by iron nanoparticles for the treatment of human lung cancer. J Exp Nanosci. 2021;16(1):397–409.
- Shi S, Zhang X, Zhou Y, et al. Immunosuppression reduces lung injury caused by mycoplasma pneumonia infection. Sci Rep. 2019;9(1):7147.
- Guo L, Liu F, Lu MP, et al. Increased T cell activation in BALF from children with mycoplasma pneumoniapneumonia. Pediatr Pulmonol. 2015;50(8):814–819.
- Chen Z, Shao X, Dou X, et al. Role of the mycoplasma pneumonia/interleukin-8/neutrophil axis in the pathogenesis of pneumonia. PLoS One. 2016;11(1):e146377.
- Boddeke EW. Involvement of chemokines in pain. Eur J Pharmacol. 2001;429(1–3):115–119.
- Hassan I, Husain FM, Khan RA, et al. Ameliorative effect of zinc oxide nanoparticles against potassium bromate-mediated toxicity in Swiss albino rats. Environ Sci Pollut Res Int. 2019;26(10):9966–9980.
- Mahmoud ARH, Shalaby NMM. Ameliorative effect of zinc oxide nanoparticles on nicotine induced testicular dysfunction; biochemical and histological study. Toxicol Environ Health Sci. 2019;11(2):104–113.
- Afifi M, Almaghrabi OA, Kadasa NM. Ameliorative effect of zinc oxide nanoparticles on antioxidants and sperm characteristics in streptozotocin-induced diabetic rat testes. Biomed Res Int. 2015;2015(2015):153573.