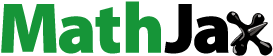
Abstract
In recent days, the green synthesized nanomagnetic biocomposites have been evolved with tremendous potential as the future catalysts. This has encouraged us to design and synthesis of a novel Cu NPs fabricated potato starch functionalized magnetic nanomaterial (Fe3O4@starch/Cu nanocomposite). The prepared nanocomposite were characterized using advanced analytical techniques like FT-IR, FESEM, TEM, EDX, elemental mapping and ICP-OES. The biogenic synthesized Fe3O4@starch/Cu nanocomposite was explored in the anti-ovarian cancer investigations against ovarian cancer cell lines (PA-1, Caov-3, SW 626, and SK-OV-3). The material exhibited significant cytotoxicities against the ovarian cancer cell lines. However, it was considerably inactive against the normal HUVEC cell line. The antioxidant potential of Fe3O4@starch/Cu nanocomposite was also investigated by DPPH method. The Fe3O4@starch/Cu nanocomposite revealed the significant antioxidant potentials according to the IC50 value.
1. Introduction
Cancer is one of the death leading causes, characterized by uncontrolled growth and abnormal cell proliferation. Cancer is caused by external factors such as chemicals and tobacco, as well as internal factors such as genetic mutations and hormones. Cancer is usually diagnosed 10 years or more after exposure to chemicals. Cancer is treated with various methods such as surgery, radiation therapy and chemotherapy [Citation1–4]. The main high cancer mortality cause is the inability to deliver targeted drugs to cancer cells without side effects on healthy cells. It seems that nanotechnology can be an excellent solution to this problem. Nanotechnology is one of the new and convenient branches that has been considered by researchers today and has created many opportunities for progress in medical sciences [Citation5–9]. Nanotechnology, as its name implies, deals with nanoscale objects. In addition to medical sciences, this technology is also used in military, agricultural, diagnostic methods, magnetic imaging, sensors and rapid material detection [Citation6–10]. Researchers use this technology to diagnose and treat many diseases. So that this science has been accepted in recent years as an important branch that has made great progress by focusing on molecular methods. In nanotechnology, which is based on atoms and molecules, materials can be made that are used in surgery, dentistry, various experiments in experimental sciences, biomechanical systems, fight against microorganisms, etc [Citation11–14]. Although many studies have been done on the effect of nanoparticles, scientists still do not have complete control over the range of their effects. In the discussion of imaging methods, less invasive methods are much more appropriate, so nanoparticles are ideal candidates for developing such imaging methods [Citation8–12]. Nanotechnology uses engineered materials to produce physiological responses and nerve stimulation while minimizing side effects. Unlike conventional systems such as tablets and solutions, nanoparticles intelligently control and perpetuate the distribution of drugs in different parts and make them more effective [Citation15–19]. Today, nanoparticles are used in various fields and have shown good potential to increase the quality of human life. Much research has been done on nanomaterials in medical, health and pharmaceutical issues [Citation16–21]. Different types of nanoparticles have been studied in these fields, one of the most widely used of which is AgNPs, which have gained much attention because of their low cost, various roles in the body and easy preparation. Metallic nanoparticles have been applied in many therapeutic fields including diabetes, antibacterial and cancer, wound healing, and positive results have been reported [Citation22,Citation23]. Recently, biological methods have been used in the preparation of nanoparticles to reduce the environmental effects of their production and to increase their medicinal effects. In these methods, bacteria, fungi and even plants are used in the preparation of these materials [Citation20–23]. Using plant extracts to synergize the effects of medicinal plants is expanding. In these methods, with the help of plant extracts used in traditional medicine to control various diseases, the desired nanoparticles are prepared [Citation15–19]. Plant extracts through active ingredients such as flavonoids, steroids, alkaloids, etc. reduce the size of nanomedicines and thus better and faster penetration into cells and compounds prepared with a synergistic effect cause better control of diseases [Citation21–23].
Being encouraged, we demonstrate herein the designing and bio-inspired synthesis of a magnetically isolable bio-nanocomposite (Fe3O4@starch/Cu) as a novel hybrid material for the use as a nanoformulated therapeutic drug against human ovarian cancer (Scheme 1). In order of that, cytotoxicity studies were carried out against standard human ovarian cancer cell lines, PA-1, Caov-3, SW 626, and SK-OV-3.
2. Experimental
2.1. Synthesis of Fe3O4@starch nanocomposite
Ferrite NPs were synthesized following earlier reference [Citation23]. The pre-synthesized support was suspended into 100 mL water by sonication for 20 min. Then an aqueous starch solution (0.5 g in 100 mL H2O) was slowly added into the suspension and sonicated again for complete mixing. The whole mixture was then vigorously stirred under heating (80 °C) for 5 h. Finally, the nanocomposite was retrieved magnetically followed by thorough washing (H2O/EtOH) and drying under vacuum at 60 °C.
2.2. Synthesis of Fe3O4@starch/Cu
In order to impregnate Cu into the final material, the Fe3O4@starch nanocomposite (0.5 g) was initially dispersed over 30 mL H2O by sonication for 20 min. Then the Cu precursor, Cu(OAc)2.2H2O solution (0.03 g in 10 mL) was introduced slowly and the mixture was made alkaline by addition of 1 M NaOH solution (1 mL). The mixture was sonicated again for well mixing and subsequently refluxed at 100 °C for 2 h. The oxygenated functions of starch facilitated the in situ reduction of Cu2+ ions into the NPs. The as-synthesized material (Fe3O4@starch/Cu) was collected using a magnet, washed thrice with aqueous acetone and finally dried in air. The Cu content was estimated by ICP-OES analysis, being 0.09 mmol/g.
2.3. Therapeutic effects analysis
DPPH (1,1-Diphenyl-2-picryl-hydrazyl) was applied to determine the nanoparticles antioxidant properties. 2 ml of 0.2 mM DPPH solution was mixed by 5 ml of NPs suspension at dilations of 1-1000 μg/ml. The incubation was done for 30 min at room temperature and absorbed at 517 nm in the spectrophotometer. The free radical adsorption percentage was calculated by the below formula so that A0 was the control sample adsorption and A1 was the desired sample adsorbent [Citation24–28]:
In this study, the anticancer effects of Fe3O4@starch/Cu nanocomposite samples against the ovarian cancer cells (PA-1, Caov-3, SW 626, and SK-OV-3) were investigated.
MTT test determines the induced toxicity on cancer cells and the vitality of the cells and their survival rate. The method of this experiment is as follows: in the first stage, we add 100 μl of the relevant culture medium to the 96-cell plate inside each of its wells. Then we prepare the cell suspension from the desired cell inside the flask. From this cell suspension, sludge 10 microliters into each well and transfer the plate to the incubator after finishing work. After 24 h, remove the plate from the incubator and transfer it to the hood. Gently drain the entire culture medium, doing this carefully so the cells are not removed. Refill the inside of each well from the culture medium. Then we treat the concentrations of nanoparticles synthesized to the cells to perform all these steps on three separate plates. The plates were evaluated for periods of 24, 48 and 72 h during which they were incubated. For example, after the expiration of 24 h, we take the plate out of the incubator. Slowly drain all the contents of the wells so as not to damage the cells. After this, 15 μl of MTT solution was added to each of the wells and the plate was re-incubated at 37° C for 4 h. Then, at the end of the desired time, the plate was removed from the incubator, all MTT contents were removed from the wells, and 100 μl of DMSO was added. At this stage, the amount of dye produced was evaluated using an ELISA plate reader device set at 570 nm. The basis of this experiment is based on the fact that living cells, tetrazolium crystals are regenerated by mitochondrial succinate dehydrogenase enzyme and cause the formazan crystals formation. These blue crystals are only capable of dissolving in organic solvents such as isopropanol and DMSO. The absorption spectrophotometer is then read and the number of living cells that have the enzymatic activity of succinate dehydrogenase is determined [Citation24–28].
3. Results and discussion
The hybrid magnetic nanocomposite was engineered in a stepwise modification method. The core ferrite NPs were functionalized initially by starch followed by the immobilization of Cu2+ ions. Starch biofunctions used to reduce the metal ions in a toxic-free environment following a green-metric biogenic pathway (Scheme 1). The as-synthesized material was subsequently characterized over a range of analytical techniques like FT-IR, SEM, TEM, EDX, elemental mapping and ICP-OES method.
displays the comparative FT-IR data for Fe3O4@starch/Cu nanocomposite and its components in order to justify its sequential synthesis. The unmodified ferrite NPs could be characterized by the vibrational peaks at 582 and 635 cm−1 attributed to Fe-O-Fe stretching and ferrite spinel bonding respectively (). The surface O-H function of Fe3O4 was identified by the broad vibrational band at 3417 cm−1. shows the typical organo-functional vibrations of starch, appeared at 1649 cm−1, 1375 cm−1, 1159 cm−1 and 1083 cm−1 corresponding to O-H bending, C–O stretching, glycosidic C–O–C stretching and C–C stretching respectively. The spectrum of Fe3O4@starch/Cu nanocomposite appears to be an amalgam of the two previous spectra, validating their successful conjugation in the final material (). However, some of the important peaks like Fe–O–Fe stretching have found to be slightly shifted and the surface hydroxyl peak intensity was reduced in the final material due to strong co-ordination between the composite organic functions and Cu NPs.
Structural morphology, size and shape of the Fe3O4@starch/Cu material were visualized by electron microscopic studies (SEM and TEM). SEM image clearly displays the material of spherical shaped having average dimension of ∼20-40 nm (). The surface analogy of a continuous and homogeneous layer provides an idea of the biomolecular modification. Due to manual sample preparation the sample looks aggregated.
The SEM data were further justified and more intrinsically studied by TEM investigations. displays the resultant images of Fe3O4@starch (3a) and Fe3O4@starch/Cu (3 b). Two kinds of homomorphic particles can be viewed there. The grey and black colored particles represent the Fe3O4 and Cu NPs respectively. The dark colored Cu NPs are also round shaped and are slightly larger than Fe3O4 NPs. Size of the particles of Fe3O4 and Cu NPs are 15-20 and 20-30 nm respectively. A thin layer of starch polymers over the particle surface can be observed in both the images.
SEM analysis was accompanied by EDX investigation, being equipped with the instrument. This study tells us about the elemental constitution of the nanocomposite. depicts the related profile, representing the occurrence of Fe, Cu as metal and C, O as non-metals. The non-metals undoubtedly validate the biopolymer association. These outcomes were additionally warranted by elemental mapping study (). X-ray scanning of a section of the SEM image produces the compositional map that supports the SEM data. Moreover, the distribution of atomic species over the surface can also be viewed in the form of colored dots. evidently shows the homogeneous dispersion of all the elemental species.
The extent and variety of cancer outbreaks during previous periods have led to the development of various treatment methods that depending on the progress, extent, type of the disorder and the condition of the patient, a several methods combination is applied to treat cancer [Citation16, Citation29,Citation30]. Despite many efforts to prevent and treat cancer, the disease is growing rapidly and remains a global killer. Today, various methods are used to treat cancer, including surgery, radiation and chemotherapy therapy, which in most cases destroy healthy cells, which can cause toxic effects in the patient. So, it seems necessary to find modern methods of cancer treatment to reduce side effects [Citation17, Citation29–32]. Metal nanoparticles can be used as a suitable option for cancer treatment. Metal nanoparticles disrupt cellular message transmission, disrupting the cellular communication network [Citation17, Citation31,Citation32]. In addition, metal nanoparticles are involved in DNA damage and induction of programmed cell death, which is the main in cancer treatment. Recent research has shown that metal nanoparticles produced by the green method have more inhibitory effects on cancer cells than commercial nanoparticles [Citation17, Citation30–32]. Over the years, research has shown that nanoparticles have been creatively designed to orient and treat tumors. These nanoparticles have been considered in various sciences such as medicine due to their positive and useful applications. There are several methods, such as chemical and biological methods for synthesizing metal nanoparticles [Citation17, Citation32]. In this way, fungi also can synthesize metal nanoparticles, which these nanoparticles synthesized with the help of fungi have many benefits. One of these benefits is that the amount produced from these nanoparticles is very large and have environmental compatibility, and these metal nanoparticles can act as antimicrobial agents against disease-causing microorganisms. At present, science states that the tendency to create and use nanometer-sized materials is significantly increasing [Citation17, Citation30–32].
The outcomes of antioxidant capacity investigation of Fe3O4@starch/Cu nanocomposite and BHT at various concentrations, being expressed as % inhibition, have been documented in and . Markedly, the % inhibition gradually increases with the increase in sample concentrations.
Table 1. The IC50 of Fe3O4@Starch/Cu and BHT.
In the cytotoxic studies, the processed ovarian malignancy cell lines i.e. PA-1, Caov-3, SW 626, and SK-OV-3 were treated with the various concentrations of Fe3O4@starch/Cu nanocomposite. The material was also studied over normal HUVEC cell line. Notably, there was no significant toxicity caused by Fe3O4@starch/Cu nanocomposite on them. The results have been demonstrated in and .
Table 2. The IC50 values of Fe3O4@Starch/Cu against the normal and ovarian cancer cell lines.
The % cell viability of ovarian cancer cell lines (PA-1, Caov-3, SW 626, and SK-OV-3) decreases dose-dependently in the presence of Fe3O4@starch/Cu nanocomposite. clearly display the corresponding outcomes. The IC50 values of Fe3O4@starch/Cu nanocomposite were calculated to be 145, 191, 294, and 197 µg/mL against the four cell lines, respectively ().
4. Conclusion
In summary, we demonstrate herein the design and stepwise synthesis of Cu NP decorated starch modified Fe3O4 nanocomposite material (Fe3O4@starch/Cu). The biopolymer was used as a protective layer over the Fe3O4 NPs. It also carried out the function to reduce the anchored Cu2+ ions in a toxic-free green pathway towards Cu NPs. The polar organofunctions of starch also provided significant stability to the in situ synthesized Cu NPs by encapsulation or capping. This in turn also helped the NPs not to self-aggregate. A broad range of analytical methods were used to characterize the material features. Subsequently, it was subjected to biological application in the determination of antioxidant capacity as well as cytotoxicity against human ovarian cancer cell lines (PA-1, Caov-3, SW 626, and SK-OV-3) in vitro. The nanocomposite exhibited significant antioxidant potential as validated by DPPH assay. Towards cytotoxicity studies the material showed decreased % cell viability against the three ovarian cancer cell lines, dose dependently. The findings of the current research definitely add significant impact towards the inhibition of human ovarian cancer.
Disclosure statement
No potential conflict of interest was reported by the author(s).
Data availability statement
Data available on request from the authors.
Additional information
Notes on contributors
Ping Hou
Ping Hou and Hongyi Kuang are co-first authors, they contributed equally to this work. Also, all authors have had similar role at the all research parts of the recent project.
Hongyi Kuang
Ping Hou and Hongyi Kuang are co-first authors, they contributed equally to this work. Also, all authors have had similar role at the all research parts of the recent project.
References
- Johannsen M, Thiesen B, Wust P, et al. Magnetic nanoparticle hyperthermia for prostate cancer. Int J Hyperthermia. 2010;26(8):790–795.
- Bañobre-López M, Teijeiro A, Rivas J. Magnetic nanoparticle-based hyper-thermia for cancer treatment. Rep Pract Oncol Radiother. 2013;18(6):397–400.
- Klein S, Sommer A, Distel LV, et al. Superparamagnetic iron oxide nanoparticles as novel X-ray enhancer for low-dose radiation therapy. J Phys Chem B. 2014;118(23):6159–6166.
- Chatterjee DK, Fong LS, Zhang Y. Nanoparticles in photodynamic therapy: an emerging paradigm. Adv Drug Deliv Rev. 2008;60(15):1627–1637.
- Zhang AP, Sun YP. Photocatalytic killing effect of TiO2 nanoparticles on Ls- 174-t human Colon carcinoma cells. WJG. 2004;10(21):3191–3193.
- Thevenot P, Cho J, Wavhal D, et al. Surface chemistry influences cancer killing effect of TiO2 nanoparticles. Nanomedicine. 2008;4(3):226–236.
- Colon J, Hsieh N, Ferguson A, et al. Cerium oxide nanoparticles protect gastrointestinal epithelium from radiation-induced damage by reduction of reactive oxygen species and upregulation of superoxide dismutase 2. Nanomedicine. 2010;6(5):698–705.
- Wason MS, Colon J, Das S, et al. Sensitization of pancreatic cancer cells to radiation by cerium oxide nanoparticle-induced ROS production. Nanomedicine. 2013;9(4):558–569.
- Tarnuzzer RW, Colon J, Patil S, et al. Vacancy engineered ceria nanostructures for protection from radiation-induced cellular damage. Nano Lett. 2005;5(12):2573–2577.
- Ali D, Alarifi S, Alkahtani S, et al. Cerium oxide nanoparticles induce oxidative stress and genotoxicity in human skin melanoma cells. Cell Biochem Biophys. 2015;71(3):1643–1651.
- Neri D, Supuran CT. Interfering with pH regulation in tumors as a therapeutic strategy. Nat Rev Drug Discov. 2011;10(10):767–777.
- Seo JW, Chung H, Kim MY, et al. Development of watersoluble single-crystalline TiO2 nanoparticles for photocatalytic cancer-cell treatment. Small. 2007;3(5):850–853.
- Hou Z, Zhang Y, Deng K, et al. UV-emitting upconversion-based TiO2 photosensitizing nanoplatform: near-infrared light mediated in vivo photodynamic therapy via mitochondria-involved apoptosis pathway. ACS Nano. 2015;9(3):2584–2599.
- Cui S, Yin D, Chen Y, et al. In vivo targeted deep-tissue photodynamic therapy based on near-infrared light triggered upconversion nanoconstruct. ACS Nano. 2013;7(1):676–688.
- Lucky SS, Idris NM, Li Z, et al. Titania coated upconversion nanoparticles for near-infrared light triggered photodynamic therapy. ACS Nano. 2015;9(1):191–205.
- Idris NM, Lucky SS, Li Z, et al. Photoactivation of core-shell titania coated upconversion nanoparticles and their effect on cell death. J Mater Chem B. 2014;2(40):7017–7026.
- Caputo F, de Nicola M, Ghibelli L. Pharmacological potential of bioactive engineered nanomaterials. Biochem Pharmacol. 2014;92(1):112–130.
- Danhier F, Feron O, Preat V. To exploit the tumor microenvironment: Passive and active tumor targeting of nanocarriers for anti-cancer drug delivery. J Control Release. 2010;148(2):135–146.
- Laurent S, Dutz S, Häfeli UO, et al. Magnetic fluid hyperthermia: Focus on superparamagnetic iron oxide nanoparticles. Adv Colloid Interface Sci. 2011;166(1-2):8–23.
- Maier-Hauff K, Ulrich F, Nestler D, et al. Efficacy and safety of intratumoral thermotherapy using magnetic iron-oxide nanoparticles combined with external beam radiotherapy on patients with recurrent glioblastoma multiforme. J Neurooncol. 2011;103(2):317–324.
- Kolosnjaj-Tabi J, di Corato R, Lartigue L, et al. Heat-generating iron oxide nanocubes: Subtle “destructurators” of the tumoral microenvironment. ACS Nano. 2014;8(5):4268–4283.
- Bhattacharyya S, Kudgus RA, Bhattacharya R, et al. Inorganic nanoparticles in cancer therapy. Pharm Res. 2011;28(2):237–259.
- Veisi H, Ghorbani M, Hemmati S. Sonochemical in situ immobilization of Pd nanoparticles on green tea extract coated Fe3O4 nanoparticles: an efficient and magnetically recyclable nanocatalyst for synthesis of biphenyl compounds under ultrasound irradiations. Mater Sci Eng C Mater Biol Appl. 2019;98:584–593.
- Chen J, Patil S, Seal S, et al. Rare earth nanoparticles prevent retinal degeneration induced by intracellular peroxides. Nat Nanotechnol. 2006;1(2):142–150.
- Das M, Patil S, Bhargava N, et al. Auto-catalytic ceria nanoparticles offer neuroprotection to adult rat spinal cord neurons. Biomaterials. 2007;28(10):1918–1925.
- Korsvik C, Patil S, Seal S, et al. Superoxide dismutase mimetic properties exhibited by vacancy engineered ceria nanoparticles. Chem Commun. 2007;(10):1056–1058.
- Hong R, Han G, Fernandez JM, et al. Glutathione-mediated delivery and release using monolayer protected nanoparticle carriers. J Am Chem Soc. 2006;128(4):1078–1079.
- Zangeneh MM, Zangeneh A, Pirabbasi E, et al. Falcaria vulgaris leaf aqueous extract mediated synthesis of iron nanoparticles and their therapeutic potentials under in vitro and in vivo condition. Appl Organometal Chem. 2019c;33:e5246.
- Stewart B, Wild CP. World Cancer Report 2014. International Agency for Research on Cancer World Health Organization: Lyon, France; 2014. [(accessed on 24 March 2015)]. http://www.iarc.fr/en/publications/books/wcr/wcr-order.php.
- Rasmussen JW, Martinez E, Louka P, et al. Zinc oxide nanoparticles for selective destruction of tumor cells and potential for drug delivery applications. Exp Opin Drug Deliv. 2010;7(9):1063–1077.
- Felice B, Prabhakaran MP, Rodríguez AP, et al. Drug delivery vehicles on a nano-engineering perspective. Mater Sci Eng C. 2014;41:178–195.
- Fernandes E, Ferreira JA, Peixoto A, et al. New trends in guided nanotherapies for digestive cancers: a systemic review. J Control Release. 2015;209:288–307.