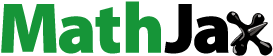
Abstract
Chitosan-based nano-sized particles increase the penetration of the drug through the narrow junction into the bloodstream and target the specific site. The objective of this study was to prepare chitosan nanoparticles to entrap a hydrophobic drug (tamoxifen citrate), followed by the alginate coating of the developed nanoparticles to decrease their degradation in the acidic pH. Drug-loaded chitosan nanoparticles were prepared by the ionic gelation method. Alginate coating was done by dissolving sodium alginate to buffer solution and drug-loaded chitosan nanoparticles drop-wise under mild agitation. The size of alginate coated chitosan nanoparticles, zeta potential, surface morphology, in-vitro drug release, and entrapment efficiency was measured. The optimised formulation of both uncoated (SH3) and coated (SH7) formulation showed the particle size, PDI, and zeta potential with values 221 & 338 nm, 0.161 & 0.230 and 36.5 & −20.7 mV, respectively. The resulted nanoparticle surface was non-porous. The percentage yield of the optimised formulation SH3 was 28% and SH6 was 33%. The entrapment efficiency of the optimised formulation SH3 (uncoated formulation) and SH6 (coated formulation) is 69.5 and 58.51%, respectively. Chitosan nanoparticles were successfully prepared to entrap tamoxifen citrate. The coating of chitosan nanoparticles decreased their degradation in the acidic pH.
1. Introduction
Breast cancer is a malignant tumour and second most cause of cancer deaths in women Worldwide, Despite of effective diagnostic procedure and treatment its mortality rate is still high [Citation1]. About 30% of patient develops metastatic breast cancer that is untreatable and their life expectancy decreased to 3 years [Citation2]. Number of breast cancer cases is increasing in Pakistan and even in males it is detected in northern areas of Pakistan [Citation3]. One out of eight females in United States and one out of twelve females in Britain suffered from breast cancer [Citation4]. According to statistics of GLOBOCAN 18.1 million of breasts cancer was diagnosed in 2018 worldwide [Citation5]. Therapeutic decisions are made on the protein biomarkers that are expressed on some breast cancers such as oestrogen receptors, ERBB2 and progesterone receptors [Citation6]. Chemotherapeutic agents are widely used for cancer therapy but its use is limited due to toxic effects on normal cells, short half-life, low solubility and bioavailability [Citation7]. In order to overcome the problem many approaches such as liposomes, dendrimers, polymeric and lipid nanoparticles are used [Citation8].
Nanotechnology is widely used to prepare nanocarriers that deliver drug in localised tissues to reduce the dosing frequency, dosage quantity with same pharmacological activity [Citation5]. Nanoparticles are small colloidal particles that have range from 10 to 1000 nm and are mostly used below 200 nm for passive delivery to treat cancers due to its nano-size [Citation9]. Many materials such as ampiphilic polymers and surfactants are widely used to deliver cancer drugs for targeted drug delivery due to excellent biological and physico-chemical properties [Citation10]. Polymeric nanoparticles due to its biodegradable property are widely used as nano-carriers for anti-cancer drugs [Citation11]. Many methods are used for preparation of nanoparticles such as ionic gelation, spray drying, complex coacervation, solvent evaporation and desolvation method [Citation12].
Chitosan is a natural biopolymer that is widely used in pharmaceutical preparation due to its non-antigenicity, non-toxicity, biocompatibility and biodegradability [Citation13]. This polymer is extracted from chitin which is found in the exoskeleton of insect cuticle, fungi and crustaceans [Citation12]. The presence of one amino group and two hydroxyl groups makes chitosan a hydrophilic macromolecule [Citation13]. It has bioadhesive property in which the positive amino group of chitosan interacts with negative charged mucous layer [Citation14].
Oral delivery of chitosan is limited due to its solubility at gastric pH that leads to loss of its penetration and mucoadhesive property [Citation15]. Alginate and chitosan complex have important development in polymer based drug delivery [Citation16]. Alginate is an anionic copolymer that is hydrophilic in nature obtained from the intracellular spaces and cell wall of bacteria and brown algae [Citation14]. Sodium alginate possess gastric resistant property and its coating on chitosan nanoparticles prevent chitosan dissolution at gastric environment and increase bio adhesive property [Citation15].
Tamoxifen is a selective oestrogen receptor modulator (SERM) that is used to treat breast cancer for more than 20 years but it has some limitations due to its serious side effects and low solubility in the water [Citation17]. The anti-estrogenic activity of the tamoxifen depends on location of the tissue and the concentration of the dose [Citation18]. Tamoxifen shows good bioavailability upon oral administration and inhibit binding of oestradiol with the oestrogen receptor as a result oestrogen response is prevented [Citation19]. Oral delivery of tamoxifen is achieved by using its tablets or solution at a dose of 10 mg twice a day [Citation20]; however, it leads to extensive metabolism of tamoxifen in liver [Citation21]. The oral bioavailability of tamoxifen could be enhanced by using its encapsulated formulations, such as microparticles and nanoparticles using suitable polymers. Few studies have already been conducted for the preparation of nanospheres using polyethylene glycol, nanoparticles using poly(-caprolactone), and poly lactide-co-glycolide microspheres. Moreover, chitosan features could also be improved by joining it with other polymers, such as alginate and hyaluronic acid [Citation22].
Since microparticulate tamoxifen could be delivered orally to avoid first pass effect and enhance its bioavailability, the purpose of this study was to prepare pH-responsive chitosan nanoparticles to incorporate tamoxifen, an anti-cancer drug. Alginate coating on tamoxifen-loaded chitosan nanoparticles was done with an aim of protecting chitosan from stomach environment and release nanoparticles in the intestine.
2. Materials and methods
Tamoxifen was imported from ForeNutri (Wuhan) Co, Ltd, China. Chitosan, sodium tripolyphosphate (STPP), sodium alginate, and potassium phosphate monobasic (KH2PO4) were purchased from Sigma Aldrich, Germany. Potassium chloride (KCl) was purchased from UNI-CHEM Chemical Reagents. All other reagents used in this study were analytical grade.
2.1. Preparation of chitosan nanoparticles
Chitosan nanoparticles were prepared through ionic gelation method. In brief, chitosan was dissolved in 2% glacial acetic acid solution. After incorporation of drug, tween 80 was added as re-suspending agent in order to prevent aggregation. Sodium hydroxide was added to chitosan solution to raise the pH up to 4.6 − 4.8. STPP was dissolved in distilled water. All solutions were filtered through 0.22 micron filter. STPP solution was added drop-wise to chitosan solution under magnetic stirrer at room temperature. Chitosan-STPP nanoparticles were formed spontaneously via STPP-initiated ionic gelation mechanism. The concentrations of various ingredients are given in . Nanoparticles was purified by centrifugation [Citation23].
Table 1. The optimised formulations based on drug polymer ratio.
2.2. Preparation of alginate-coated chitosan nanoparticles
Sodium alginate was dissolved in buffer solution and chitosan nanoparticles were dissolved in sodium alginate solution drop-wise under mild agitation, centrifuged it at 3500 rpm for 5 min and supernatant was discarded. Alginate coated chitosan nanoparticles were re-suspended into calcium chloride [Citation15].
2.3. Characterizations
2.3.1. Hydrodynamic particle size and zeta potential
The hydrodynamic particle size and zeta potential of samples was measured by zeta sizer [Citation24]. Nano ZS, Malvern, UK was used to assess the zeta potential at 37 °C.
2.3.2. Surface morphology
Morphology of the nanoparticles was determined by scanning electron microscopy (SEM) [Citation25]. The lyophilised free-flowing powdered nanoparticle samples were put on the SEM's metal holder, which was made up of double tape. The gold sputter coating was then applied to the nanoparticles' surfaces. The shape of the drug complex was determined, as well as image was obtained with good resolution. SEM study was conducted by using VEGA 3, TE SCAN, Czech Republic SEM apparatus.
2.3.3. Fourier transmission infra-red spectroscopy
The chemical composition of the chitosan nanoparticles both loaded with and without drug was characterised with Fourier transmission infra-red spectroscopy [Citation26]. FTIR was conducted to verify the hydrogel network development and tentative interaction using Tensor 25 series FTIR (Germany).
2.3.4. In vitro drug release
In vitro release of drug from chitosan nanoparticles and alginate coated chitosan nanoparticles performed in gradient pH media such as simulated gastrointestinal environment (simulated gastric fluid pH 1.2, simulated intestinal fluid pH 6.8, simulated intestinal fluid pH 7.4) and release was conducted at room temperature. pH-gradient biphasic dissolution test was used in this study. This test was performed in dissolution I. Firstly, dissolution was done in gastric buffer (pH 1.2) for 30 min. After enhancing volume, the pH of the medium was changed by using 5 M NaOH to get pH 6.8 and 7.4 of the intestinal mediums [Citation15].
2.3.5. Entrapment efficiency
Entrapment efficiency is calculated by the difference between total drug feed and free drug in the supernatant by following formula [Citation27]:
2.3.6. Stability study
As discussed above, particle size, zeta potential, entrapment efficiency and in vitro dissolution of the samples was done at the predetermined time intervals to assess the product’s stability.
3. Results
describes various parameters of the prepared nanoparticles.
Table 2. Various parameters of the prepared nanoparticles.
3.1. Hydrodynamic diameter, zeta potential and PDI
Formulation SH3 (Chitosan to STPP = 6:1) shows the least hydrodynamic diameter of the nanoparticles while another uncoated formulation SH1 (Chitosan to STPP = 4:1) also shows a small hydrodynamic diameter. On the other hand, hydrodynamic diameter and zeta potential of SH5 and SH7 formulations are the lowest out of the coated formulations, as given in . In addition, PDI of the optimised formulation SH3 and SH7 was 0.161 and 0.230, respectively.
3.2. Surface morphology
shows the SEM images of SH3 and SH7 formulations. Surface morphology of the obtained nanoparticles shows that the resulted nanoparticle surface was spherical, smooth and non-porous.
3.3. Fourier transmission infra-red spectroscopy
According to FTIR spectra () of the chitosan, hydroxyl group is detected at the peak of 3284 cm−1, while stretched CH bond was noted at 2852.68 cm−1. Peaks of the carbonyl as well as amide group is detected at 1686 and 1374 cm−1. FTIR spectra for the cross linker shows peaks at 1130.21 and 890 cm−1. That stretching exhibit the phosphate groups. According to FTIR spectra of the tamoxifen loaded nanoparticles, three peaks in the spectra are considered to characterise the formulation. Peak for the hydroxyl group in the spectra are at 3357.49 cm−1, while peak that is noticed for the (C–O–C) was at 1602 cm−1 and peak for the (–NH) was observed at 1062.20 cm−1.
3.4. In vitro drug release
In vitro release of uncoated formulations and coated formulations is shown in . Both graphs clearly describe a difference in the rate of drug release, revealing that the coated formulation release the drug in a more controlled manner, especially during initial 15 h. The uncoated formulation showed a maximum drug release in approximately first 10 h. About 10–18% of drug release occurred from the uncoated formulations during first half hour, while the coated formualtions allowed the release of approximately 2–3% of drug during the same duration. Similar fidnings have been reported by Md et al. [Citation28]. Moreover, dissolution priofiles of formulations SH1–SH4 were considered similar, as f1 (similarity factor) was between 0 and 15. Same finding was noted in case of formulations SH5-SH8. However, all the uncoated formulations showed f1 > 15 when compared with that of formulations SH5-SH8, it revealed that the compared profiles were different from ewach other.
R2 value of both optimised formulation were highest (0.9633 and 0.9703) in Korsmeyer-Peppas model. It explains the release of the drug from nanoparticles follows the diffusion mechanism. The “n” value of the SH3 nano-formulation was 0.302 which shows diffusion that follows Fick’s law. The value of the ‘n’ of optimised SH7 formulation was 1.448 which follows the erosion of the polymeric chain and release of the drug. Both formulations follow the first order kinetics when data is put into this model ().
Table 3. Models of drug dissolution for optimised formulation SH3 and SH7.
3.5. Entrapment efficiency
Entrapment efficiency is calculated by the difference between total drug feed and free drug in the supernatant by following formula [Citation27]:
The formulation was obtained by consideration of the drug–polymer ratio. Four formulations were obtained. The optimisation parameters for CS-NP and Alginate-coated CS-NP are narrated in the and .
Table 4. Optimisation parameters for CS-NP.
Table 5. Optimisation parameters for Alg-CS-NP.
3.6. Stability study
The stability study data revealed that there was non-significant (p > 0.05) variation in the particle size, zeta potential, entrapment efficiency and in vitro dissolution profiles of the samples stored in different environmental conditions.
4. Discussion
4.1. Zeta sizer and zeta potential
Zeta size, zeta potential and PDI are important factors in the characterisation of the polymeric nanoparticle. Size range of the polymeric nanoparticles should be in range of 1–1000 nm, nanoparticles with this size easily penetrate the physiological barriers. Zeta potential is the charge difference between the two surfaces that is very important in nanoformulation stability.
Zeta sizer was used to evaluate the zeta potential and average size of the chitosan nanoparticles and sodium alginate coated chitosan nanoparticles. Formulation SH3 and SH4 (Chitosan to STPP = 6:1) shows the least particle size of the nanoparticles while the formulations SH2 and SH6 (Chitosan to STPP = 5:1) also shows the small particle size. When concentration of the STPP is increased in the formulation with respect to the chitosan as a result particle size is increased. When there is increase in concentration of the STPP in the chitosan formulation there is increase in the turbidity of the formulation due to formation of the big size nanoparticles. These nanoparticles aggregate that result in the turbidity of the formulation. When the chitosan to STPP ratio is less to 4:1 and STPP amount is increased in the formulation that causes flocculation of the aggregates and formulation becomes unstable [Citation29].
Positive charge of protonated amino group on chitosan results in high zeta potential due to which polymeric nanoparticles in the nanosuspension repel each other. But presence of large amount of the STPP negative ions causes decrease in zeta potential of the formulations. This is the reason that optimised formulations SH3 and SH7 shows the smallest mean particle sizes [Citation30]. Zeta potential of the polymeric nanoparticles causes the protonation of the TPP at low pH ≤ 3. Similarly pH of the formulation that is higher than the caused aggregates of the nanoparticles and as a result unstable formulations are formed [Citation31]. So that is the reason pH of the all formulations should be maintained at 4.6. Zeta potential of the optimised chitosan nanoparticles is 36.5 mV and zeta potential of the sodium alginate coated chitosan nanoparticles is −20.7 mV. Similarly, zeta size of optimised formulation SH3 was 221.9 nm and SH7 was 338 nm.
4.2. Scanning electron microscopy
The resulting nanoparticle surface was non-porous, which could inhibit the diffusion of drug from the nanoparticles and allow control release of the drug from these nanoparticles. Some of the nanoparticles are irregular in shape with different sizes. According to the previous studies if there is increase in the reaction time of the STPP and chitosan on magnetic stirrer there is production of the irregular nanoparticles that are attached to one another and form cluster like structure. There is another reason for the irregular shape of the nanoparticles that are crystallisation of the STPP anions. When STPP is added into chitosan solution it should be stirred approximately for one hour to get regular as well as uniform shape nanoparticles [Citation32].
4.3. Entrapment efficiency
The entrapment efficiency of the optimised formulation SH3 and SH6 is 69.5 and 58.51% respectively. That describes, there is increase in the entrapment efficiency of the drug when STPP to chitosan ratio is increased. When quantity of the STPP is increased larger nanoparticles are formed with increased entrapped drug. That results in production of large particles and big pellets on centrifugation [Citation33]. The previous studies shows when there is increase in mass ratio of chitosan to STPP there was decrease in the entrapment efficiency of the formulation. Percentage entrapment efficiency of this study is related to the previous studies that are conducted. This study shows that when there is increase in the STPP ratio there is increase in the particle size of the nanoparticles that result in increase of the entrapment efficiency [Citation34].
4.4. Percentage yield
Percentage yield of the optimised formulation SH3 is 28% and SH6 is 33%. The STPP to chitosan ratio had direct effect on the percentage yield of the formulation. As the amount of the STPP to chitosan is increased, the percentage yield of the formulations was increased. There is competitive chemical reaction between phosphate group of the STPP and hydroxyl group of the chitosan that leads to the less percentage yield of the formulations. Due to the competition between the STPP and chitosan there was low formulation of the nanoparticles that leads to the less percentage yield.
4.5. In vitro drug dissolution studies
The dissolution profile of the tamoxifen loaded chitosan nanoparticles and coated polymeric nanoparticles were performed in acidic and phosphate buffer. Different buffer were considered (1.2 pH, 6.8 pH and 7.4 pH) for the dissolution studies using USP dissolution apparatus-II at 37 C ± 0.5 C. Rotating speed of the dissolution was kept at 50 rpm. After 24 h percentage drug release of the both coated and uncoated formulations were determined by DDSolver “adds in” of Microsoft Excel [Citation35]. Percentage drug release of the uncoated and coated formulations are given in . Optimised formulation such as SH3 and SH7 shows maximum drug release with good particle size.
Polymeric chitosan nanoparticles have the advantage of increased formulation stability, increased solubility of the hydrophobic drugs and controlled drug release [Citation36]. Oral delivery of the polymeric chitosan nanoparticles is limited due to solubility at acidic pH that causes dissolution of chitosan at the acidic pH of the gastric media. Coating of sodium alginate resists the dissolution of chitosan at that pH [Citation15]. Release rate of the coated chitosan nanoparticles were slower as compared to the uncoated nanoparticles. This was due to the fact that, in uncoated nanoparticles drug release was faster in the gastric pH due to destruction of the chitosan at acidic pH. Release of the drug from nanoparticles were slower in the phosphate buffer due to the fact that chitosan have mucoadhesive property and adhere to the mucous lining of the colon were it slowly release the drug [Citation37]. In coated nanoparticles the outer layer of the nanoparticles contains sodium alginate that have the gastric resistant property. Sodium alginate coating protect chitosan nanoparticle release in the stomach environment and pass-through acidic environment to the phosphate environment where at 7–8 pH sodium alginate is dissolved and chitosan nanoparticles containing drug released. Due to mucoadhesive property of the chitosan it get attached to the mucous lining and slowly release drug [Citation15].
5. Conclusion
From the study performed, it was concluded that pH responsive tamoxifen loaded nanoparticles coated with sodium alginate were successfully prepared. In vitro drug release studies justified that little amount of drug was released in the acidic medium, rather an appreciable amount of drug was released at the intestinal pH because sodium alginate is a pH-responsive polymer which specifically allows drug release at colon pH in a controlled manner.
Disclosure Statement
No potential conflict of interest was reported by the author(s).
Data availability statement
All the data has been added to this article.
Additional information
Notes on contributors
Muhammad Khurram Waqas
Muhammad Khurram Waqas is Assistant Professor at UVAS in the field of Pharmaceutics. His research is focused on skin treatments.
Shees Safdar
Shees Safdar is a Pharmacist and M. Phil. researcher.
Manal Buabeid
Manal Buabeid is an Associate Professor in the field of neuropharmacology.
Akram Ashames
Akram Ashames is Pharmaceutical Chemist and Associate Professor at Ajman University.
Muhammad Akhtar
Muhammad Akhtar is Professor at IUB and works on nanomedicines.
Ghulam Murtaza
Ghulam Murtaza is tenured Associate Professor and works on oral transdermal/topical DDS.
References
- Ghahremani F, Shahbazi-Gahrouei D, Kefayat A, et al. AS1411 aptamer conjugated gold nanoclusters as a targeted radiosensitizer for megavoltage radiation therapy of 4T1 breast cancer cells. RSC Adv. 2018;8(8):4249–4258.
- Cabeza L, Ortiz R, Arias JL, et al. Enhanced antitumor activity of doxorubicin in breast cancer through the use of poly (butylcyanoacrylate) nanoparticles. Int J Nanomed. 2015;10:1291–1306.
- Bhurgri Y. Karachi cancer registry data–implications for the national cancer control program of pakistan. Asian Pac J Cancer Prev. 2004;5(1):77–82.
- Akram M, Iqbal M, Daniyal M, et al. Awareness and current knowledge of breast cancer. Biol Res. 2017;50(1):33.
- Tagde P, Kulkarni G, Mishra DK, et al. Recent advances in folic acid engineered nanocarriers for treatment of breast cancer. J Drug Delivery Sci Technol. 2020;56:101613.
- Yezhelyev MV, Gao X, Xing Y, et al. Emerging use of nanoparticles in diagnosis and treatment of breast cancer. Lancet Oncol. 2006;7(8):657–667.
- Nag M, Gajbhiye V, Kesharwani P, et al. Transferrin functionalised chitosan-PEG nanoparticles for targeted delivery of paclitaxel to cancer cells. Colloids Surf B Biointerfaces. 2016;148:363–370.
- Abriata JP, Turatti RC, Luiz MT, et al. Development, characterisation and biological in vitro assays of paclitaxel-loaded PCL polymeric nanoparticles. Mater Sci Eng C Mater Biol Appl. 2019;96:347–355.
- Martinez A, Arana P, Fernández A, et al. Synthesis and characterisation of alginate/chitosan nanoparticles as tamoxifen controlled delivery systems. J Microencapsul. 2013;30(4):398–408.
- Prabaharan M. Chitosan-based nanoparticles for tumour-targeted drug delivery. Int J Biol Macromol. 2015;72:1313–1322.
- Sun Y, Yu B, Wang G, et al. Enhanced antitumor efficacy of vitamin E TPGS-emulsified PLGA nanoparticles for delivery of paclitaxel. Colloids Surf B Biointerfaces. 2014;123:716–723.
- Gogoi P, Dutta A, Ramteke A, et al. Preparation, characterisation and cytotoxic applications of curcumin‐(±) α‐lipoic acid coloaded phosphorylated chitosan nanoparticles in MDA MB 231 breast cancer cell line. Polym Adv Technol. 2020;31(11):2827–2841.
- Naskar S, Koutsu K, Sharma S. Chitosan-based nanoparticles as drug delivery systems: a review on two decades of research. J Drug Target. 2019;27(4):379–393.
- Katuwavila NP, Perera A, Samarakoon SR, et al. Chitosan-alginate nanoparticle system efficiently delivers doxorubicin to MCF-7 cells. J Nanomater. 2016;2016:1–12.
- Bagre AP, Jain K, Jain NK. Alginate coated chitosan core shell nanoparticles for oral delivery of enoxaparin: in vitro and in vivo assessment. Int J Pharm. 2013;456(1):31–40.
- Criado-Gonzalez M, Fernandez-Gutierrez M, San Roman J, et al. Local and controlled release of tamoxifen from multi (layer-by-layer) alginate/chitosan complex systems. Carbohydr Polym. 2019;206:428–434.
- Martinez A, Muniz E, Teijon C, et al. Targeting tamoxifen to breast cancer xenograft tumours: preclinical efficacy of folate-attached nanoparticles based on alginate-cysteine/disulphide-bond-reduced albumin. Pharm Res. 2014;31(5):1264–1274.
- Ravikumara NR, Madhusudhan B. Chitosan nanoparticles for tamoxifen delivery and cytotoxicity to MCF-7 and vero cells. Pure Appl Chem. 2011;83(11):2027–2040.
- Albert EL, Shirosaki Y, Abdullah CAC. Drug release and kinetic study of tamoxifen citrate conjugated with magnetite nanoparticle for drug delivery application. IJAER. 2018;13:5360–5369.
- Buckley MM, Goa KL. Tamoxifen. A reappraisal of its pharmacodynamic and pharmacokinetic properties, and therapeutic use. Drugs. 1989;37(4):451–490. Apr
- Tukker JJ, Blankenstein MA, Nortier JW. Comparison of bioavailability in man of tamoxifen after oral and rectal administration. J Pharm Pharmacol. 1986;38(12):888–892.
- Bhagwat GS, Athawale RB, Gude RP, et al. Formulation and development of transferrin targeted solid lipid nanoparticles for breast cancer therapy. Front Pharmacol. 2020;11:614290. PMID: 33329007; PMCID: PMC7729133.
- Tığlı Aydın RS, Pulat M. 5-Fluorouracil encapsulated chitosan nanoparticles for pH-stimulated drug delivery: evaluation of controlled release kinetics. J Nanomater. 2012;2012:1–10.
- Wei W, Lv P-P, Chen X-M, et al. Codelivery of mTERT siRNA and paclitaxel by chitosan-based nanoparticles promoted synergistic tumour suppression. Biomaterials. 2013;34(15):3912–3923.
- Yang R, Yang S-G, Shim W-S, et al. Lung-specific delivery of paclitaxel by chitosan-modified PLGA nanoparticles via transient formation of microaggregates. J Pharm Sci. 2009;98(3):970–984.
- Li F, Li J, Wen X, et al. Anti-tumour activity of paclitaxel-loaded chitosan nanoparticles: an in vitro study. Mater Sci Eng C. 2009;29(8):2392–2397.
- Vivek R, Babu VN, Thangam R, et al. pH-responsive drug delivery of chitosan nanoparticles as tamoxifen carriers for effective anti-tumour activity in breast cancer cells. Colloids Surf B Biointerfaces. 2013;111:117–123.
- Md S, Abdullah S, Alhakamy NA, et al. Development, optimization, and in vitro evaluation of novel oral Long-Acting resveratrol nanocomposite in-Situ gelling film in the treatment of colorectal cancer. Gels. 2021;7(4):276.
- Rampino A, Borgogna M, Blasi P, et al. Chitosan nanoparticles: preparation, size evolution and stability. Int J Pharm. 2013;455(1–2):219–228.
- Konecsni K, Low N, Nickerson M. Chitosan–tripolyphosphate submicron particles as the carrier of entrapped rutin. Food Chem. 2012;134(4):1775–1779.
- Nasti A, Zaki NM, De Leonardis P, et al. Chitosan/TPP and chitosan/TPP-hyaluronic acid nanoparticles: systematic optimisation of the preparative process and preliminary biological evaluation. Pharm Res. 2009;26(8):1918–1930.
- Yang W, Fu J, Wang T, et al. Chitosan/sodium tripolyphosphate nanoparticles: preparation, characterisation and application as drug carrier. J Biomed Nanotechnol. 2009;5(5):591–595.
- Grenha A, Seijo B, Remunán-López C. Microencapsulated chitosan nanoparticles for lung protein delivery. Eur J Pharm Sci. 2005;25(4-5):427–437.
- Hu B, Pan C, Sun Y, et al. Optimisation of fabrication parameters to produce chitosan − tripolyphosphate nanoparticles for delivery of tea catechins. J Agric Food Chem. 2008;56(16):7451–7458.
- Chen G, Zhao Y, Xu Y, et al. Chitosan nanoparticles for oral photothermally enhanced photodynamic therapy of Colon cancer. Int J Pharm. 2020;589:119763.
- Wang JJ, Zeng ZW, Xiao RZ, et al. Recent advances of chitosan nanoparticles as drug carriers. Int J Nanomed. 2011;6:765–774.
- Patel BK, Parikh RH, Aboti PS. Development of oral sustained release rifampicin loaded chitosan nanoparticles by design of experiment. J Drug Deliv. 2013;2013:370938.