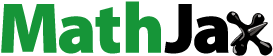
Abstract
According to the neuroprotective effects of Zn metal and Elaeagnus angustifolia leaves separately, we tried to synthesize a modern neuroprotective drug (ZnNPs in an aqueous medium by E. angustifolia leaves extract). After ZnNPs synthesizing, they were analyzed by UV-Vis. and FT-IR spectroscopy and FE-SEM. The sizes of 10–50 nm were seen by FE-SEM images in our nanoparticles. In addition, the nanoparticles were spherical morphologically. To investigate the antioxidant effects, DPPH free radicals test was used. The ZnNPs removed half of the free radicals at the 27 µg/mL concentration. In the neurological experiments, we concluded that Nicotine causes cell death in nerve-like PC12 cells by inducing apoptosis and cell inflammation. Zinc nanoparticles repressed the inflammatory cytokines (IL-1β, IL-6, and TNF-α) production, caspase 3 activity, and mitochondrial membrane disruption. These events showed that zinc nanoparticles repressed Nicotine-induced cell death in PC12 cells, in a dose-dependent manner.
1. Introduction
Nicotine is the most important component of the pharmacological action of tobacco compounds. This substance has several pharmacological effects on the nervous system. Many effects are probably due to the ability of nicotine to induce the release of various neurotransmitters [Citation1–3]. Smoking cessation often leads to a withdrawal symptoms, although specific symptoms and severity may vary from person to person. Withdrawal symptoms begin two hours after the last cigarette is consumed and usually peak around the first 24 to 48 h and may last for weeks or months. The most common symptoms are irritability, anxiety, restlessness, difficulty concentrating, and a craving for nicotine [Citation4–6]. Other symptoms include headache, drowsiness, insomnia, decreased heart rate and blood pressure, increased muscle tension, increased hostility and increased appetite. Increased appetite and fear of being overweight after quitting smoking can help reduce withdrawal motivation, especially in women [Citation7–9]. The American Psychiatric Association (APA) identifies nicotine poisoning with at least four of these symptoms, which develop within 24 h of quitting or reducing consumption. After nicotine injection, a variety of androgen opioids, such as enkephalins, are released into the brain, indicating that nicotine interferes with opioids in the central nervous system [Citation8–11]. At the cellular level, nicotine increases opioid gene expression in various brain nuclei and adrenal chromaffin cells. This may be because nicotine interferes greatly with opioids. For example, nicotine administration can reduce withdrawal syndrome in morphine-dependent mice [Citation10, Citation11]. Nicotine also induces analgesia in mice by activating nicotinic and opioid receptors. In addition, morphine can inhibit the effects of withdrawal syndrome in nicotine-dependent mice [Citation9–11]. Studies have shown that intrathecal administration of apple nicotine enhances analgesia induced by morin and beta-endorphin secretion in mice. The presence of this interaction between nicotine and morphine enhances the possibility of nicotine interference in the pleasurable properties of morphine [Citation8–11]. Given that nicotine and morphine both can induce self-expression and conditioned site preference in laboratory mice and mice and this property is due to the ability of these drugs to induce psychological dependence due to stimulation of dopamine release in the mesolimbic system [Citation7–10].
Drug transfer to the central nervous system (CNS) is a challenge faced by biologists due to protective barriers. The effectiveness of the drug in the CNS depends on the drug ability to cross the BBB and reach remedial concentrations after administration in the brain [Citation12]. Thus, failure to treat CNS disorders is often not because of a drug potency lack but to difficulty in drug delivery. Nanotechnology, especially nanoparticles in drug delivery, has received promising responses in recent years. In general, drug treatments must have a good shelf life in the blood to be effective [Citation13]. Nanoparticles are typically more effective due to their small size. Numerous factors such as weight, surface charge, surface properties, size and shape affect the shelf life of nanoparticles in the blood. However, once nanoparticles enter the body, these structures may be identified and removed by phagocytes such as neutrophils and macrophages [Citation14, Citation15]. The high surface-to-volume ratio of nanoparticles allows them to bind multiple ligands to their surface. For example, solid lipid nanoparticles activated with ferulic acid were tested for antioxidant purposes in animal models of stroke [Citation16]. In another study, neuroprotective peptides for the combined treatment of Alzheimer’s were incorporated into nanosystems of the glycoside monocylate tetrahexosilgan glycoside [Citation17]. In addition, nanoparticles coated with polysorbate or polyethylene with a size of less than 80 nm can be protected from the reticuloendothelial system and circulate in the blood for a long time. Today, due to the negative charge on the surface of endothelial cells, electrostatic interaction can be caused by functionalizing the surface of nanoparticles with positively charged biomolecules, thus facilitating the passage of nanoparticles through the BBB. Nanoparticles also pass through the BBB through transferrin and lipoprotein receptors through adsorption [Citation18]. For example, cell transfer begins with a transfer of transferrin to its receptor and continues with endocytosis, which can allow a mixture of nanoparticles and drugs to cross the BBB [Citation19]. In addition, the long-term circulation of modified nanoparticles in the blood makes it easier to interact and penetrate endothelial cells and allows more control over cell function. However, despite current advances in nanoscience, drug applications for nanoparticles continue to face problems, such as unknown tissue interactions and unpredictable consequences [Citation14]. In this regard, high permeability cerium oxide nanoparticles can prevent the formation of scar tissue that prevents healing in spinal cord lesions [Citation20]. Cationic nanoparticles can be present in the bloodstream for a long time without causing toxic effects and have better permeability to the CNS than anionic nanoparticles. For example, gold cationic nanoparticles enter the cell without energy consumption and bypass methods such as endocytosis, affecting cell function [Citation21, Citation22]. Nanomaterials can be used as carriers of antioxidant enzymes. Antioxidant enzymes can reduce reactive oxygen species (ROS) but remain in the blood for a short time and then break down; therefore, it is difficult to cross the BBB due to their short presence [Citation23]. Most drug delivery systems are in the form of polymer nanoparticles, which can pass through tight cellular connections. They also have a high drug loading capacity and increase the effectiveness of combination drugs [Citation24]. In this regard, nanocapsules and nanospheres are very important in modern drug delivery, because they have a high load capacity of the drug and can protect the drug, thus raising the likelihood of the drug reaching the brain. These nanomaterials are also used to prevent retinal endothelial system macrophages from detecting the drug [Citation25].
Various studies have shown that the biological synthesis of nanoparticles with plant extracts causes the formation of particles with excellent antioxidant and neuroprotective properties [Citation26]. Herbal medicines contain various compounds, including antioxidants that can be used to eliminate or reduce the toxic effects of various oxidants. Studies show that some herbal remedies reduce the risk of developing diseases of the nervous system, including Alzheimer’s [Citation27–30]. Essential oils and plant extracts inhibit acetylcholinesterase and butyrylcholinesterase in vitro. In addition to their antioxidant activity, nicotine effects and anti-inflammatory properties have also been reported [Citation28, Citation29]. Plant antioxidants can reduce aluminum-induced neurotoxicity. Studies have shown that plant extracts improve memory. The effect of herbs on memory may improve brain function through the antioxidant properties of these herbs [Citation28–31]. These extracts may also enhance the function of central neurotransmitter systems affecting memory. Therefore, these plants for therapeutic cases of memory loss due to various factors, needs further investigation [Citation29–31].
Using herbal remedies against various diseases has been common since ancient times. Early humans used plants as healing agents. Nature provides many plants that have medicinal values. The medicinal properties of some plants have been identified, but the properties of many plants remain unknown [Citation31, Citation32]. Therefore, it is necessary to conduct studies and identify their therapeutic properties. Elm with the scientific name of Elaeagnus angustifolia has been introduced in traditional medicine as an analgesic and anti-inflammatory [Citation33, Citation34]. Decoction and extract of Elm fruit is used to treat fever, jaundice, asthma, tetanus and rheumatoid arthritis. Since the effects of Elm have been considered in this study, the composition and medicinal use of Elm are described in such a way that its fruits and leaves have significant amounts of flavonoids (Flavonoids), terpenoids (Terpenoids), Sitosterol, Alkaloids and Carvacrol [Citation35]. Elecampane contains potassium, magnesium, sodium, iron, calcium, zinc and copper. The fruit contains various vitamins including A, B and K, and its core contains linoleic fatty acids, phospholipids, glycolipids and beta cytosterols [Citation36]. Extracts of some plants can show their effect during pregnancy or during childbirth, which indicates the importance of maternal diet during pregnancy [Citation37]. Research has shown that elm fruit compounds easily cross the placental barrier and reach the fetus [Citation38]. Flavonoids are the most important substances in plant extracts. They increase the series-tail length by increasing the absorption of calcium compounds in the bone diaphysis tissue [Citation39]. Carvacrol is also a very effective antimicrobial component in essential oils [Citation40], whose antimicrobial effect is due to the permeability of the cell membrane, which can be chelated with membrane surface cations and vital activities. On the other hand, due to the presence of various vitamins in the extract of Elm fruit, the increase of these vitamins also causes teratogenic effects on the development of fetal organs, especially the central nervous system [Citation39, Citation40].
In the recent research, we tried to synthesize a modern neuroprotective drug (ZnNPs in an aqueous medium by E. angustifolia leaves extract). The novelty of the recent study is formulating a novel neuroprotective drug from a biological source or plant extract (E. angustifolia leaves extract).
2. Experimental
2.1. Material
Dimethyl sulfoxide (DMSO), Antimycotic antibiotic solution, hydrolysate, decamplmaneh fetal bovine serum, Ehrlich solution, 4-(Dimethylamino)benzaldehyde, 2,2-diphenyl-1-picrylhydrazyl (DPPH), carbazole reagent, borax-sulphuric acid mixture, Dulbecco’s Modified Eagle Medium (DMED), and phosphate buffer solution (PBS) all were achieved from Sigma-Aldrich company of USA.
2.2. Synthesis of zinc nanoparticles
To prepare the aqueous extract, 200 g of the dried plant leaves were powdered and boiled in water for five hours. The extract was evaporated after filtration [Citation41, Citation42]. After concentrating the crude extract, 50 mL was added to 15 mL of Zn(NO3)2.6H2O (0.04 M). After 24 h stirring, the ZnNPs was made. By three times washing, then centrifuging, the precipitate was dried. Then, the recent nanoparticles were used for characterizing and in vitro experiments.
In this study, to identify the chemical bonds created in the structure of nanoparticles that originated from plant extract, Fourier transform infrared spectroscopy (FTIR, Thermo Nicolet model Nexus 870, made in the USA) with a range of 100 to 1000 cm was used.
To obtain the size and morphology of the biosynthesized nanoparticles with plant extracts, they were examined by electron microscopy (FE-SEM) (Zeiss, Germany) after coating with gold. For this purpose, the nanoparticles were dissolved in some water and the resulting suspension on the grid was examined microscopically at a voltage of 25 kV.
The solution containing biosynthesized gold nanoparticles was analyzed by UV-visible spectrophotometry (Biotech EPOCH, US) between 200 and 600 nm after one hour of reaction time.
2.3. Neuroprotective analyses of ZnNPs
In the recent test, PC12; ATCC® CRL-1721TM (adrenal phaeochromocytoma) cells were cultured in Gibco RPMI1640 cell culture medium according to the protocols. Nicotine changes the cells morphology, so apoptotic cells lead to the cell treatment with 100 mM of water diluted-Nicotine. For Zn(NO3)2, E. angustifolia leaf extract, and zinc nanoparticles solutions in RPMI1640 water cell culture medium, at first, it was solved in DMSO and added to culture medium by 0.1% final volume. Then, 18 h after plating, the PBS (37 °C) was used to wash the cells and they divided into eight groups under the following conditions: 0.1 M Nicotine, Control without 001 M Nicotine, 0.1 M nicotine and 8 μg of Zn(NO3)2, 0.1 M nicotine and 16 μg of Zn(NO3)2, 0.1 M nicotine and 8 μg of E. angustifolia, 0.1 M nicotine and 16 μg of E. angustifolia. 0.1 M nicotine and 8 μg of nanoparticles. 0.1 M nicotine and 16 μg of nanoparticles.
T plate the PC12 cells, the PRMI1640 medium was used. After 15 h, PBS (37 °C) was used to wash the cells. Then, the various treatments were added to the cells. To separate the cells from the plate, Trypsin was applied. To remove the supernatant, the sample centrifuging was done by adding lysate buffer for 15 min. After transferring the cells to the plates, 5 μL N-acetyl-Asp-Glu-Val-Asp-p-nitroanilineDEVD-pNA was added incubated in 37 °C for 3 h. Then, pNA releasing related to the caspase-3 activity was received by Biotek (USA) Spectrophotometer [Citation43–46].
Various groups were exposed to 10 mg/mL rhodamine-123 for 45 min. Then, PBS (37 °C) was used to wash the cells. In continuing, 950 μL triton X-100 was added to each well. After 2 h, the solutions were moved to microtubes to centrifuge at 15,000 rpm for 30 min. A fluorescence microplate reader (488 nm excitation and 520 nm emission) measured the cells fluorescence absorbance [Citation46].
The pro-inflammatory cytokines concentrations of TNFα, IL-6 and IL-1β were determined by Rat V-Plex Kit.
To determine the PC12 cell death index, the TUNEL staining was applied. Eight random wells were chosen to count the TUNEL positive cells by an Olympus AX-70 fluorescent microscope. The cell death index is the apoptotic cells to total cells ratio [Citation45].
Trypan blue was applied to evaluate the cell viability. The cells (5 × 104 cells/mL) were cultured by various treatments of I-VIII and incubated at 37 °C in 5% CO2 for 48 h. After the cells trypsinization, 250 μL of cell suspension was mixed with 40 μL of Trypan blue 0.4% and suspended after 4–5 min by the Neubauer Lamela. The samples cell viability was determined by the following formula [Citation44]:
2.4. Determination of the antioxidant property of zinc nanoparticles by DPPH
The antioxidant effects of the recent nanoparticles were determined according to the Hosseinimehr et al (2011) study. First, the solutions of nanoparticles with dilations of 1–1000 µg/ml were prepared. The BHT was considered as a positive control. Then, 3 ml of the DPPH were mixed with the 1 ml of the nanoparticles with several concentrations. After 30 min of incubating at room temperatures, the exact wavelengths were measured at 517 nm according to the below formula [Citation43]:
2.5. Qualitative measurement
The obtained results were analyzed by SPSS (version 20) software using one-way ANOVA, followed by Tukey test (p ≤ 0.01).
3. Results and discussion
The previous studies have indicated that the ZnNPs have a range sizes of 10 to 100 nm [Citation41, Citation42]. The sizes of 10–90 nm were seen by FE-SEM images in our nanoparticles (). In addition, the nanoparticles were spherical morphologically. The recent morphology of ZnNPs was observed in the previous studies [Citation32].
The FT-IR spectra of ZnNPs are shown in . The peaks are as an organic compounds present evidence that are due to the ZnNPs surface. The peaks at 2907 and 3389 cm−1 are for the aliphatic C-H and O-H stretching bands; the peaks at the (609 and 692), 1618 and 1394 cm−1 are for Zn-O, C = O and C = C stretching bands. The various FT-IR bands are due to the several functional groups presence. The previous research have proved the recent peaks [Citation31, Citation32].
The UV–Vis. spectrum of the green-synthetic nanoparticles of zinc nanoparticles is shown in . The creation of the green synthetic zinc nanoparticles was observed. There were advanced surface plasmon resonance band appearances at the wavelength ranges of 264, 287 and 321 nm that approved the formation of the nanoparticles.
In a recent study, the standard (butylated hydroxytoluene) revealed higher antioxidant activities than ZnNPs. The IC50 of E. angustifolia, butylated hydroxytoluene, and zinc nanoparticles were 217, 23, and 27 µg/mL, respectively ().
Our experiment findings showed that Nicotine reduced significantly (p ≤ 0.01) the cell viability and raised the caspase-3 activity and inflammatory cytokines concentrations. Treatment with both doses of ZnNPs raised the cell viability and cell proliferation potentials due to the cell cytotoxicity reduction ().
Figure 5. The caspase 3 absorption in different treatments after 48 h. G1: Nicotine, G2: Control, G3: 0.1M nicotine and 5 μg of Zn(NO3)2, G4: 0.1M nicotine and 10 μg of Zn(NO3)2, G5: 0.1M nicotine and 5 μg of E. angustifolia, G6: 0.1M nicotine and 10 μg of E. angustifolia, G7: 0.1M nicotine and 5 μg of nanoparticles, G8: 0.1M nicotine and 10 μg of nanoparticles. *Reveal the notable difference (p ≤ 0.01) between other treatments with nicotine treatment.
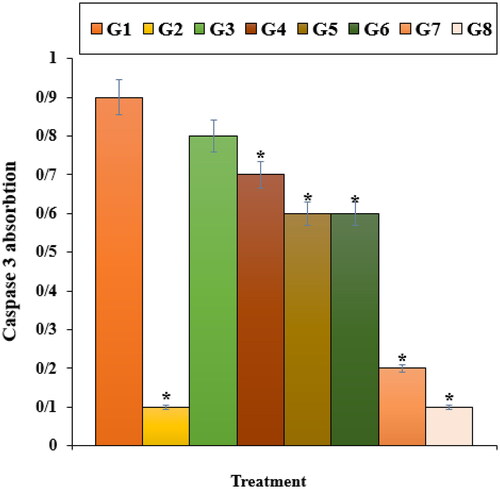
Figure 6. The cytokine concentration in different treatments after 48 h. G1: Nicotine, G2: Control, G3: 0.1M nicotine and 5 μg of Zn(NO3)2, G4: 0.1M nicotine and 10 μg of Zn(NO3)2, G5: 0.1M nicotine and 5 μg of E. angustifolia, G6: 0.1M nicotine and 10 μg of E. angustifolia, G7: 0.1M nicotine and 5 μg of nanoparticles, G8: 0.1M nicotine and 10 μg of nanoparticles. *Reveal the notable difference (p ≤ 0.01) between other treatments with nicotine treatment.
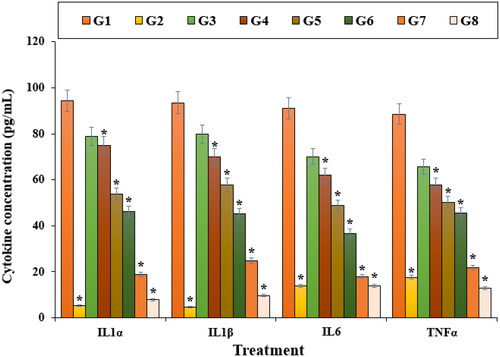
Figure 7. The apoptosis index of different treatments after 48 h. G1: Nicotine, G2: Control, G3: 0.1M nicotine and 5 μg of Zn(NO3)2, G4: 0.1M nicotine and 10 μg of Zn(NO3)2, G5: 0.1M nicotine and 5 μg of E. angustifolia, G6: 0.1M nicotine and 10 μg of E. angustifolia, G7: 0.1M nicotine and 5 μg of nanoparticles, G8: 0.1M nicotine and 10 μg of nanoparticles. *Reveal the notable difference (p ≤ 0.01) between other treatments with nicotine treatment.
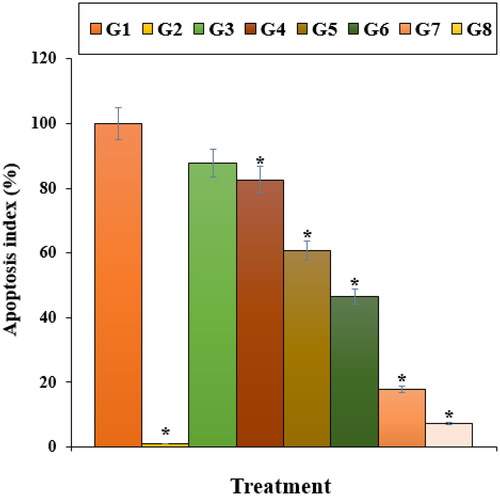
Figure 8. The cell viability of different treatments after 48 h. G1: Nicotine, G2: Control, G3: 0.1M nicotine and 5 μg of Zn(NO3)2, G4: 0.1M nicotine and 10 μg of Zn(NO3)2, G5: 0.1M nicotine and 5 μg of E. angustifolia, G6: 0.1M nicotine and 10 μg of E. angustifolia, G7: 0.1M nicotine and 5 μg of nanoparticles, G8: 0.1M nicotine and 10 μg of nanoparticles. *Reveal the notable difference (p ≤ 0.01) between other treatments with nicotine treatment.
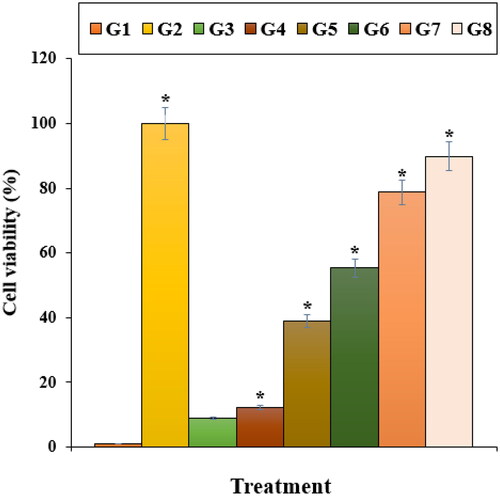
The release of free radicals and inflammatory cytokines is one of the events that occur during neuronal destruction and neurological diseases. Reactive Nitrogen Species (RNS) such as Nitric oxide (NO); also the production of reactive nitrogen species can lead to apoptosis and cell death [Citation47–52]. The production of many free radicals, which are produced by various factors such as damage to the nervous system, can cause cell damage such as damage to cellular proteins, damage to DNA (Deoxy ribonucleic acid), membrane oxidation and changes in lipoproteins and cause various diseases [Citation53–58]. The small size of the nanoparticles increases the surface-to-volume ratio and as a result can absorb more free radicals, and it can be an excellent solution to deal with free radicals secreted after nerve damage. It should be noted that peripheral nerve researchers typically use the sciatic nerve as a study method for nerve repair using different doses, as this modeling is relatively inexpensive in mice [Citation54–57]. Soluki et al. investigated the cerium oxide nanoparticles effects on improved tissue changes and motor function following sciatic nerve injury in rats. The speed of the repair process and the motor function improvement in the groups treated with cerium oxide significantly increased than control group [Citation55]. In another study on oxidative stress-induced in endothelial cells and neurons, it was found that cerium oxide nanoparticles reduced cell apoptosis and were effective in repairing peripheral nerves [Citation56]. Increasing the survival of neuron cells also occurs by reducing oxidative damage due to the antioxidant properties of cerium oxide, in which cerium oxide nanoparticles can act quickly and absorb active species of oxygen [Citation57]. The effect of this substance in angiogenesis, modulation of the nervous system, anti-cancer applications, inhibition of hypertension, antibacterial effects, lowering cholesterol levels, reducing damage to damaged tissue have been confirmed [Citation58]. Due to the properties of nanoparticles, there is great hope for diagnostic and therapeutic possibilities in medicine. One of the leading molecules for diagnostic and therapeutic applications is magnetic nanoparticles that can deliver drugs to preferred locations using a magnetic field [Citation53–55]. Metal nanoparticles prevent the accumulation and inflammation of damaged tissue, and are also used as biomarkers to evaluate the effectiveness of the drug. With the effective delivery of growth factors and regeneration stimulation, the role of magnetic nanoparticles in peripheral nerve repair has been confirmed. The researchers also showed that the covalent combination of neurotrophic factors with ZnNPs increased the stability and improved peripheral nerves [Citation56–58].
In this study, the apoptosis experiment by the TUNEL test revealed that Nicotine induced apoptosis and caused DNA fragmentation in nerve-like PC12 cells. Further tests presented that Nicotine causes apoptosis in these cells by decreasing the mitochondrial membrane potential. Our findings also revealed that ZnNPs significantly (p ≤ 0.01) raised the mitochondrial membrane potential and reduced the DNA fragmentation rate (). The previous studies revealed that Nicotine yielded the free radicals [Citation59–61]. The free radicals cause apoptosis and cellular with DNA molecules degradation [Citation59]. Also, free radicals directly damage the DNA and increase neuron apoptosis [Citation59, Citation60].
Figure 9. The mitochondrial membrane potential of different treatments after 48 h. G1: Nicotine, G2: Control, G3: 0.1M nicotine and 5 μg of Zn(NO3)2, G4: 0.1M nicotine and 10 μg of Zn(NO3)2, G5: 0.1M nicotine and 5 μg of E. angustifolia, G6: 0.1M nicotine and 10 μg of E. angustifolia, G7: 0.1M nicotine and 5 μg of nanoparticles, G8: 0.1M nicotine and 10 μg of nanoparticles. *Reveal the notable difference (p ≤ 0.01) between other treatments with nicotine treatment.
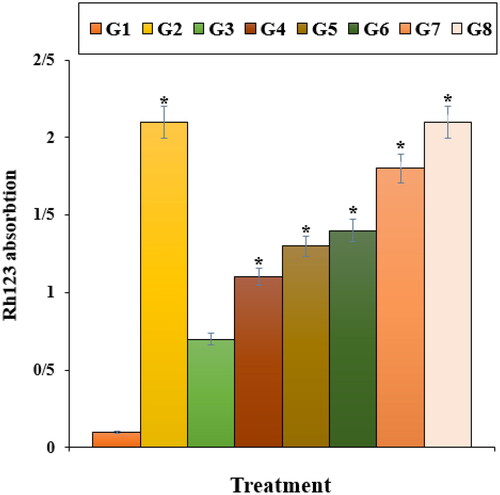
It looks that zinc nanoparticles, significantly (p ≤ 0.01) raised cell viability and mitochondrial membrane potential, and decreased inflammatory cytokines concentrations, caspase-3 activity, and DNA fragmentation due to its antioxidant potential. Recently, antioxidants have been used as a cell cytotoxicity reducer because they prevent oxidative stresses and ROS production in the cells [Citation48–51].
4. Conclusion
E. angustifolia leaf was used for synthesizing the ZnNPs. The organometallic chemistry tests such as FT‐IR, UV-Vis and FE‐SEM were used to characterize the ZnNPs. In the neurological part, nicotine significantly (p ≤ 0.01) decreased the mitochondrial membrane potential and cell viability and enhanced the DNA fragmentation, caspase-3 activity and inflammatory cytokines concentrations. Zinc nanoparticles-treated cell cutlers significantly (p ≤ 0.01) enhanced the mitochondrial membrane potential and cell viability and reduced the DNA fragmentation, caspase-3 activity and inflammatory cytokines concentrations at the high concentration against Nicotine-treated adrenal phaeochromocytoma (PC12) cells. To investigate the antioxidant effects, DPPH free radicals test was used. The ZnNPs removed half of the free radicals at the 27 µg/mL concentration. In the recent experiment, we concluded that ZnNPs green-formulated by E. angustifolia leaves extract suppressed nicotine-induced cell death in PC12 cells and can be used as a potent neuroprotective drug in humans after doing clinical trial studies.
Disclosure statement
No potential conflict of interest was reported by the authors.
Data availability statement
Data available on request from the authors.
Additional information
Notes on contributors
Mingzong Yan
Mingzong Yan is an Assistant Professor. Mingzong affiliation is Department of Brain Diseases, Penglai City Hospital of Traditional Chinese Medicine, Penglai, Shandong, 265600 China. Research interest is Nanoscience and Neurology.
Junmei Li
Junmei Li is an Assistant Professor. Junmei affiliation is Department of Internal Medicine, Penglai Traditional Chinese Medicine Hospital, Yantai Penglai, Shandong, China 265600 China. Research interest is Nanoscience and Neurology.
References
- Centers for Disease Control and Prevention. Quitting smoking among adults—United States, 2001–2010. Morb Mortal Wkly Rep. 2011;60(44):1513–1519.
- Terry L, et al. Smoking and health: report of the advisory committee to the surgeon general of the United States U-23. Department of Health, Education, and Welfare Public Health Service Publication No 1103; 1964.
- Le Moal M, Koob GF. Drug addiction: pathways to the disease and pathophysiological perspectives. Eur Neuropsychopharmacol. 2007;17(6–7):377–393.
- Glassman AH, Koob GF. Psychoactive smoke. Nature. 1996;379(6567):677–678.
- Fiore MC, Jaen CR, et al. Clinical practice guideline. In: Treating tobacco use and dependence: 2008 update. Liz Fix format problem. Rockville, MD: U.S. Department of Health and Human Services, Public Health Service; 2008.
- Manley MW, Epps RP, Glynn TJ. The clinician’s role in promoting smoking cessation among clinic patients. Med Clin North Am. 1992;76(2):477–494.
- U.S. Department of Health and Human Services. Public health service. Clinical Practice Guideline: Treatment Tobacco Use and Dependence. 2008.
- Jorenby DE, Leischow SJ, Nides MA, et al. A controlled trial of sustained-release bupropion, a nicotine patch, or both for smoking cessation. N Engl J Med. 1999;340(9):685–691.
- Cahill K, Stead LF, Lancaster T. Nicotine receptor partial agonist for smoking cessation. Cochrane Database Syst Rev. 2007;24(1):CD006103.
- Liechti ME, Markou A. Role of the glutamatergic system in nicotine dependence: implications for the discovery and development of new pharmacological smoking cessation therapies. CNS Drugs. 2008;22(9):705–724.
- D'Souza MS, Markou A. Neuronal mechanisms underlying development of nicotine dependence: implications for novel Smoking-Cessation treatments. Addict Sci Clin Pract. 2011;6(1):4–16.
- Liu G, Garrett MR, Men P, et al. Nanoparticle and other metal chelation therapeutics in alzheimer disease. Biochim Biophys Acta. 2005;1741(3):246–252.
- Hider RC, Roy S, Ma YM, et al. The potential application of iron chelators for the treatment of neurodegenerative diseases. Metallomics. 2011;3(3):239–249.
- Zhang C, Zheng X, Wan X, et al. The potential use of H102 peptide-loaded dual-functional nanoparticles in the treatment of Alzheimer’s disease. J Control Release. 2014;192(2):317–324.
- Abdolmaleki A, Asadi A, Ardabili M, et al. Importance of nano medicine and new drug therapies for cancer. Adv Pharm Bull. 2020;12(3):112–118.
- Trombino S, Cassano R, Ferrarelli T, et al. Trans-ferulic acid based solid lipid nanoparticles and their antioxidant effect in rat brain microsomes. Colloids Surf B Biointerfaces. 2013;109(9):273–279.
- Huang M, Hu M, Song Q, et al. GM1-modified lipoprotein-like nanoparticle: multifunctional nanoplatform for the combination therapy of Alzheimer’s disease. ACS Nano. 2015;9(11):10801–10816.
- De Boer A, Gaillard P. Drug targeting to the brain. Annu Rev Pharmacol Toxicol. 2007;47(1):323–355.
- Jeon M-T, Kim K-S, Kim ES, et al. Emerging pathogenic role of peripheral blood factors following BBB disruption in neurodegenerative disease. Ageing Res Rev. 2021;41(10):101–113.
- Das M, Patil S, Bhargava N, et al. Auto-catalytic ceria nanoparticles offer neuroprotection to adult rat spinal cord neurons. Biomaterials. 2007;28(10):1918–1925.
- Germain M, Caputo F, Metcalfe S, et al. Delivering the power of nanomedicine to patients today. J Control Release. 2020;326(6):164–171.
- Gallud A, Klöditz K, Ytterberg J, et al. Cationic gold nanoparticles elicit mitochondrial dysfunction: a multi-omics study. Sci Rep. 2019;9(1):1–19.
- Lee CS, Leong KW. Advances in microphysiological blood-brain barrier (BBB) models towards drug delivery. Curr Opin Biotechnol. 2020;6(6):78–87.
- Sarkar S, Levi-Polyachenko N. Conjugated polymer nano-systems for hyperthermia, imaging and drug delivery. Adv Drug Deliv Rev. 2020;163–164(3):40–64.
- Wu Q, Yang L, Wang X, et al. Mesostructured carbon-based nanocages: an advanced platform for energy chemistry. Sci China Chem. 2020;63(5):665–681.
- Middleton EJR, Kandaswami C, Theoharides TC. The effects of plant flavonoids on mammalian cells: implications for inflammation, heart disease, and cancer. Pharmacol Rev. 2000;52(4):673–751.
- Hosseinpour M, Mobini-Dehkordi M, Saffar S, et al. Antiproliferative effects of matricaria chamomilla on Saccharomyces cerevisiae. J HerbMed Pharmacol. 2013;2:49–51.
- Rafieian-Kopaei M, Baradaran A. Oxidative stress and the paradoxical effects of antioxidants. J Res Med Sci. 2013;7:628.
- Rafieian-Kopaei M. Medicinal plants and the human needs. J HerbMed Plarmacol. 2012;1:1–2.
- Alyamani AA, Albukhaty S, Aloufi S, et al. Green fabrication of zinc oxide nanoparticles using phlomis leaf extract: characterization and in vitro evaluation of cytotoxicity and antibacterial properties. Molecules. 2021;26(20):6140.
- Mahdavi B, Saneei S, Qorbani M, et al. Ziziphora clinopodioides lam leaves aqueous extract mediated synthesis of zinc nanoparticles and their antibacterial, antifungal, cytotoxicity, antioxidant, and cutaneous wound healing properties under in vitro and in vivo conditions. Appl Organometal Chem. 2019;33(11):e5164.
- Noël PH, Pugh JA, Larme AC, et al. The use of traditional plant medicines for non-insulin dependent diabetes mellitus in South Texas. Phytother Res. 1997;11(7):512–517.
- Clawson ML, Caru M, Benson DR. Diversity of Frankia strains in root nodules of plants from the families Elaeagnaceae and Rhamnaceae. Appl Environ Microbiol. 1998;64(9):3539–3543.
- Jiang F, Xie J, Dan J, et al. Selection of optimal ultrasonic extraction process of Elaeagnus angustifolia L. by uniform design. Zhong Yao Cai. 2001;24(12):891–892.
- Gupta MB, Nath R, Srivastava N, et al. Anti-inflammatory and anti-pyretic effect of sitosterol. Planta Med. 1979;3:157–163.
- Kousova RD, Kazakov A. Phenolic compounds in fruit of Elaeagnus angustifolia. Khim Prir Soedin. 1998;8:455–456.
- Koren G, Dugoua JJ, Magee L, et al. MotherNature: establishing a Canadian research network for natural health products (NHPs) during pregnancy and lactation. J Altern Complement Med. 2008;14(4):369–372.
- Dembińska-Migas W, Gill S. Flavonoids in leaves of elaeagnus angustifolia L. Pol J Pharmacol Pharm. 1973;25(6):599–606.
- Martínez-Vázquez M, Ramirez Apan TO, Aguilar H, et al. Analgesic and antipyretic activities of an aqueous extract and of the flavone learn of buddleia cordata. Planta Med. 1996;62(2):137–140.
- Buchanan RL, Shepherd AJ. Inhibition of Aspergillus parasiticus by thymol. J Food Sci. 1981;46(3):976–977.
- (a) Zangeneh MM, Zangeneh A, Pirabbasi E, Moradi R, Almasi M. Falcaria vulgaris leaf aqueous extract mediated synthesis of iron nanoparticles and their therapeutic potentials under in vitro and in vivo condition. Appl Organometal Chem. 2019;33:e5246. (b) Mahdavi B, Paydarfard S, Zangeneh MM, Goorani S, Seydi N, Zangeneh A. Assessment of antioxidant, cytotoxicity, antibacterial, antifungal, and cutaneous wound healing activities of green synthesized manganese nanoparticles using Ziziphora clinopodioides Lam leaves under in vitro and in vivo condition. Appl Organometal Chem. 2019;33:e5248. (c) Jalalvand AR, Zhaleh M, Goorani S, Zangeneh MM, Seydi N, Zangeneh A, Moradi R. Chemical characterization and antioxidant, cytotoxic, antibacterial, and antifungal properties of ethanolic extract of Allium Saralicum R.M. Fritsch leaves rich in linolenic acid, methyl ester. Photochem J Photobiol B. 2019;192:103–112. (d) Zangeneh A, Zangeneh MM. Green synthesis and chemical characterization of gold nanoparticle synthesized using Camellia sinensis leaf aqueous extract for the treatment of acute myeloid leukemia in comparison to daunorubicin in a leukemic mouse model. Appl Organometal Chem. 2019;33:e5290. doi:10.1002/aoc.5290. (e) Hemmati S, Joshani Z, Zangeneh A, Zangeneh MM. Green synthesis and chemical characterization of Thymus vulgaris leaf aqueous extract conjugated gold nanoparticles for the treatment of acute myeloid leukemia in comparison to doxorubicin in a leukemic mouse model. Appl Organometal Chem. 2019;33:e5267. doi:10.1002/aoc.5267. (f) Zhaleh M, Zangeneh A, Goorani S, Seydi N, Zangeneh MM, Tahvilian R, Pirabbasi E. In vitro and in vivo evaluation of cytotoxicity, antioxidant, antibacterial, antifungal, and cutaneous wound healing properties of gold nanoparticles produced via a green chemistry synthesis using Gundelia tournefortii L. as a capping and reducing agent. Appl Organometal Chem. 2019;33:e5015. (g) Shahriari M, Hemmati S, Zangeneh A, Zangeneh MM. Biosynthesis of gold nanoparticles using Allium noeanum Reut. ex Regel leaves aqueous extract; characterization and analysis of their cytotoxicity, antioxidant, and antibacterial properties. Appl Organometal Chem. 2019;33:e5189. doi:10.1002/aoc.5189. (h) Zangeneh MM, Saneei S, Zangeneh A, Toushmalani R, Haddadi A, Almasi M, Amiri-Paryan A. Preparation, characterization, and evaluation of cytotoxicity, antioxidant, cutaneous wound healing, antibacterial, and antifungal effects of gold nanoparticles using the aqueous extract of Falcaria vulgaris leaves. Appl Organometal Chem. 2019;33:e5216. doi:10.1002/aoc.5216.
- (a) Hemmati S, Joshani Z, Zangeneh A, Zangeneh MM. Biosynthesis and chemical characterization of polydopamine-capped silver nanoparticles for the treatment of acute myeloid leukemia in comparison to doxorubicin in a leukemic mouse model. Appl Organometal Chem. 2019;33:e5277. (b) Hemmati S, Zamenian T, Delsooz N, Zangeneh A, Zangeneh MM. Preparation and synthesis a new chemotherapeutic drug of silver nanoparticle-chitosan composite; Chemical characterization and analysis of their antioxidant, cytotoxicity, and anti-acute myeloid leukemia effects in comparison to Daunorubicin in a leukemic mouse model. Appl Organometal Chem. 2019;33:e5274. (c) Zangeneh MM Green synthesis and formulation a modern chemotherapeutic drug of Spinacia oleracea L. leaf aqueous extract conjugated silver nanoparticles; Chemical characterization and analysis of their cytotoxicity, antioxidant, and anti-acute myeloid leukemia properties in comparison to doxorubicin in a leukemic mouse model. Appl Organometal Chem. 2019;33:e5295. doi:10.1002/aoc.5295. (d) Mohammadi G, Zangeneh MM, Zangeneh A, Siavosh Haghighi ZM. Chemical characterization and anti-breast cancer effects of silver nanoparticles using Phoenix dactylifera seed ethanolic extract on 7,12-Dimethylbenz[a] anthracene-induced mammary gland carcinogenesis in Sprague Dawley male rats. Appl Organometal Chem. 2019;33:e5136. doi:10.1002/aoc.5136. (e) Zangeneh MM, Bovandi S, Gharehyakheh S, Zangeneh A, Irani P. Green synthesis and chemical characterization of silver nanoparticles obtained using Allium saralicum aqueous extract and survey of in vitro antioxidant, cytotoxic, antibacterial and antifungal properties. Appl Organometal Chem. 2019;33:e4961. (f) Hamelian M, Zangeneh MM, Shahmohammadi A, Varmira K, Veisi H. Pistacia atlantica leaf extract mediated synthesis of silver nanoparticles and their antioxidant, cytotoxicity, and antibacterial effects under in vitro condition. Appl Organometal Chem. 2019;33:e5278. doi:10.1002/aoc.5278. (g) Hemmati S, Rashtiani A, Zangeneh MM, Mohammadi P, Zangeneh A, Veisi H. Green synthesis and characterization of silver nanoparticles using Fritillaria flower extract and their antibacterial activity against some human pathogens. Polyhedron. 2019;158:8–14. (h) Zangeneh MM, Joshani Z, Zangeneh A, Miri E. Green synthesis of silver nanoparticles using aqueous extract of Stachys lavandulifolia flower, and their cytotoxicity, antioxidant, antibacterial and cutaneous wound-healing properties. Appl Organometal Chem. 2019;33:e5016. (i) Zangeneh A, Zangeneh MM, Moradi R. Ethnomedicinal plant-extract-assisted green synthesis of iron nanoparticles using Allium saralicum extract, and their antioxidant, cytotoxicity, antibacterial, antifungal and cutaneous wound-healing activities. Appl Organometal Chem. 2019;33:e5247. doi:10.1002/aoc.5247.
- Hosseinimehr SJ, Mahmoudzadeh A, Ahmadi A, et al. The radioprotective effect of Zataria multiflora against genotoxicity induced by γ irradiation in human blood lymphocytes. Cancer Biother Radiopharm. 2011;26(3):325–329.
- Baracca A, Sgarbi G, Solaini G, et al. Rhodamine 123 as a probe of mitochondrial membrane potential: evaluation of proton flux through F(0) during ATP synthesis. Biochim Biophys Acta. 2003;1606(1–3):137–146.
- Byrne A, Southgate J, Brison D, et al. Analysis of apoptosis in the preimplantation bovine embryo using TUNEL. J Reprod Infertil. 1999;117(1):97–105.
- Strober W. Trypan blue exclusion test of cell viability. Curr Protoc Immunol. 2015;2:111.
- Reuter S, Gupta SC, Chaturvedi MM, et al. Oxidative stress, inflammation, and cancer: how are they linked? Free Radic Biol Med. 2010;49(11):1603–1616.
- Arumai Selvan D, Mahendiran D, Senthil Kumar R, et al. Garlic, green tea and turmeric extracts-mediated green synthesis of silver nanoparticles: phytochemical, antioxidant and in vitro cytotoxicity studies. J Photochem Photobiol B. 2018;180:243–252.
- Rehana D, Mahendiran D, Kumar RS, et al. Evaluation of antioxidant and anticancer activity of copper oxide nanoparticles synthesized using medicinally important plant extracts. Biomed Pharmacother. 2017;89:1067–1077.
- Antony JJ, Sithika MA, Joseph TA, et al. In vivo antitumor activity of biosynthesized silver nanoparticles using Ficus religiosa as a nanofactory in DAL induced mice model. Colloids Surf B Biointerfaces. 2013;108:185–190.
- Jeong SC, Koyyalamudi SR, Jeong YT, et al. The immunostimulatory and anti-tumor activities of polysaccharide from Agaricus bisporus (brown). J Med Food. 2012;1:58–65.
- Sankar R, Maheswari R, Karthik S, et al. Anticancer activity of ficus religiosa engineered copper oxide nanoparticles. Mater Sci Eng C Mater Biol Appl. 2014;44:234–239.
- Eitan E, Hutchison ER, Greig NH, et al. Combination therapy with lenalidomide and nanoceria ameliorates CNS autoimmunity. Exp Neurol. 2015;27(3):151–160.
- Ghayour MB, Abdolmaleki A, Behnam-Rassouli M. The effect of Riluzole on functional recovery of locomotion in the rat sciatic nerve crush model. Eur J Trauma Emerg Surg. 2017;4(3):691–699.
- Santos-Sánchez NF, Salas-Coronado R, Villanueva-Cañongo C, et al. Antioxidant compounds and their antioxidant mechanism. London: IntechOpen; 2019; p. 22–31.
- Lewin SL, Utley DS, Cheng ET, et al. Simultaneous treatment with BDNF and CNTF after peripheral nerve transection and repair enhances rate of functional recovery compared with BDNF treatment alone. Laryngoscope. 1997;107(7):992–999.
- Ghayour MB, Abdolmaleki A, Rassouli MB. Neuroprotective effect of lovastatin on motor deficit induced by sciatic nerve crush in the rat. Eur J Pharmacol. 2017;18(12):121–127.
- Radosinska J, Jasenovec T, Radosinska D, et al. Ultrasmall superparamagnetic iron-oxide nanoparticles exert different effects on erythrocytes in normotensive and hypertensive rats. Biomed. 2021;11(9):377–384.
- Kroemer G. The proto-oncogene Bcl-2 and its role in regulating apoptosis. Nat Med. 1997;3(6):614–620.
- Kaufmann SH, Earnshaw WC. Induction of apoptosis by cancer chemotherapy. Exp Cell Res. 2000;256(1):42–49.
- Hengartner MO. The biochemistry of apoptosis. Nature. 2000;407(6805):770–776.