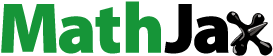
Abstract
In the present study, zinc oxide nanoparticles were green-synthesized using the aqueous extract of the rhizomes of Cyperus rotundus. The chemical methods EDX, FE-SEM, XRD, UV-Vis., and FT-IR analysis were used to characterize ZnONPs@C. rotundus were characterized by analytical techniques including. The FE-SEM image revealed a spherical shape for the nanoparticles in a size range of 38.05 to 75.41 nm and 33.09 nm was calculated for ZnONPs@ C. rotundus crystal size using the XRD results. MTT assay was used on common lung well-differentiated bronchogenic adenocarcinoma cell line i.e. HLC-1 to survey the cytotoxicity and anti-lung well-differentiated bronchogenic adenocarcinoma effects of the synthesized nanoparticles. To determine the antioxidant properties of the synthesized nanoparticles, the DPPH test was used in the presence of butylated hydroxytoluene as the positive control. The synthesized nanoparticles had very low cell viability and high anti-lung well-differentiated bronchogenic adenocarcinoma activities dose-dependently against the HLC-1 cell line without any cytotoxicity on the normal cell line (HUVEC). The synthesized nanoparticles inhibited half of the DPPH molecules at the concentration of 41 µg/mL. Maybe significant anti-human lung well-differentiated bronchogenic adenocarcinoma potentials of the synthesized nanoparticles against common human lung well-differentiated bronchogenic adenocarcinoma cell lines are linked to their antioxidant activities.
1. Introduction
Traditional Chinese Medicine (TMC) is one of the oldest folklore medicines around the world with a history of more than 2000 years. Cyperus rotundus with the Chinese name of “Xiangfu” has been a treatment for different diseases in TMC for around 1700 years [Citation1]. C.rotundus belongs to the Cyperaceae family which is the third-largest family of monocotyledonous plants. Among different parts of C.rotundus, the plant rhizome has shown the most effective to cure various illnesses. The rhizome of C. rotundus is used to treat stomach aches, bowel disorders, and inflammation in different countries of Asia [Citation2]. C. rotundus is also an agent against aging, apoptosis, cell cycle arrest, atherosclerosis, cancer, cystitis, antioxidant, antimicrobial, and prostatitis disorders [Citation2–4]. So far, the presence of a different class of secondary metabolites such as alkaloids, flavonoids, glycosides, phenols, tannins, steroids, and terpenoids in the extract of C. rotundus have been reported by several research teams [Citation3, Citation5–7]. As mentioned above, C. rotundus is known as a sufficient remedy to cure various illnesses, this ability is contributed to the existence of various secondary metabolites especially phenolic compounds [Citation2, Citation8]. Recently, C. rotundus has been used in treating several cancers such as colorectal and gastric cancers in traditional medicine [Citation4–8].
Recently, cancer is one of the diseases with high death rates in the world and it affects a wide range of people of different ages and races. Chemotherapy and radiotherapy can be mentioned among the usual and common methods of cancer treatment, which are mostly used as the chosen method of treatment in most parts of the world [Citation9–11]. Despite the widespread use of these methods, they have many disadvantages and complications that affect patients. One of the disadvantages of the common methods of cancer treatment is that in these methods, both healthy and cancerous cells are affected by the drug and undergo changes, which causes the insufficient drug to reach the cancer cells and increases the excretion of those drugs; In this way, it is necessary to increase the amount of drug injection, which will not be possible from an economic point of view and depending on the patient’s general condition and tolerance [Citation9–13]. Modern medical knowledge aims to minimize these problems and unpleasant side effects for cancer patients so that they can go through the course of their illness and treatment with less pain and damage [Citation11–13]. In the last decade, following research and obtaining more information about tumors and types of cancer, a new method called nanotechnology has been proposed to treat cancers. Nanotechnology is one of the effective and less dangerous solutions with more benefits than chemotherapy and radiotherapy for patients, which is part of the new cancer treatments [Citation14–19]. In this method, anti-cancer drugs are placed on very small particles of engineered compounds (with a size of nanometers) and are directed into the bloodstream. The interesting and key point of how this method works is that when the materials reach the nanoscale, they exhibit different behavior and characteristics that were not seen before [Citation20–24]. Also, they show unique electrical, optical and structural properties that favor our desired treatment process in this method. The use of nanotechnology for cancer treatment has attracted much attention in recent years. This technology can provide unique methods for the prediction, prevention, and early treatment of cancer [Citation25–31]. Since nanoparticles are 102 to 103 times smaller than tumor cells, they can travel easily by blood and link with tumor-specific proteins on the cancer cells inside and surface. Therefore, their use as drug delivery carriers to cancer cells has attracted much attention [Citation32–36]. Drugs encapsulated in targeted nanoparticles have the potential to ameliorate the safety and efficacy of existing drugs. Also, at present, multipurpose nanocarriers have emerged to help radiation therapy and chemotherapy [Citation36–38].
In recent years, the synthesis of zinc oxide (ZnO) with nanoparticle size has achieved considerable attraction due to its desirable chemical and physical properties. ZnO is a multifunctional, fundamental, and non-toxic inorganic material [Citation39]. ZnONPs have an optical, high exciton binding energy, large band gap, and electrical characteristics [Citation40–42]. ZnONPs are used in photocatalysts, chemical sensors, piezoelectric transducers, lasers, solar cells, and light-emitting devices [Citation43]. Besides, the application of ZnONPs in chemistry and physics, NPs are widely used in health care studies and pharmaceutical industries such as cosmetic products [Citation44]. ZnONPs green-synthesized by medicinal plants have also shown antibacterial, antifungal, and anticancer activity [Citation45–47]
The purpose of the recent research was the biosynthesis of ZnONPs@ C. rotundus by Cyperus rotundus extract, as a new modern chemotherapeutic drug to treat the lung well-differentiated bronchogenic adenocarcinoma at the cancer cell.
2. Experimental
2.1. Preparation of Cyperus rotundus rhizome extract
To obtain the Cyperus rotundus rhizome extract, 400 mL of boiled deionized water were added to 40 g of the dried and chopped plant part. The container was kept for 4 h. After filtrations, the extract was used as the reducing agent for green synthesis of ZnONPs@ C. rotundus
2.2. Green synthesis and chemical characterization of ZnONPs@ C. rotundus
A reported procedure (with some modifications) was used to green-synthesis of ZnONPs@ C. rotundus [Citation43]. First, 40 ml of the plant extract was added to 20 mL of 0.04 M Zn(NO3)2 .6H2O. Then, The pH was adjusted to 8. The reaction flask was placed in an ultrasonic bath for 70 min at 60 °C. After that time, the zinc nanoparticle was formed. The obtained ZnONPs@ C. rotundus was washed with water and ethanol three times and then centrifuged at 10,000 rpm for 15 min. Finally, the NPs were put in an oven at 45 °C for 4 h to dry. ZnONPs@ C. rotundus as a cream powder was kept in a cold place before the chemical characterization and biological activity evaluation.
2.3. MTT assay protocol
In this study, the anticancer effects of ZnONPs@ C. rotundus samples against the lung well-differentiated bronchogenic adenocarcinoma cell (HLC-1) were investigated.
MTT method was used to investigate cytotoxicity. The cells were cultured in a culture medium containing 10% FBS and streptomycin (thousand micrograms/ml) and penicillin (hundred units/ml) antibiotics. The cultures were incubated at 37 °C and in the presence of 5% CO2. To perform the MTT test, 5000 cells were placed in each house from a 96-well plate. After 24 h, concentrations of nanoparticles solvent were prepared and treated on cell lines for 24 h. After the above time, the contents of the wells were carefully removed and 200 µl of MTT dye were added to them. The microplate was placed in a 37 °C incubator for 4 h, after which the supernatant was drained and 200 µl of DMSO solution was added to each well. In the next step, the sample’s absorbance was read with a microplate reader at a wavelength of 570 nm, and the percentage of living cells was calculated relative to the optical absorbance of the control cells [Citation48–50].
2.4. DPPH assay protocol
DPPH (1,1-diphenyl-2-picryl-hydrazyl) is a way of measuring the antioxidant property based on electron transfer, in which a violet-colored solution is produced in ethanol and has a maximum absorption at the wavelength of 517 nm. To perform this test, DPPH powder was obtained from Sigma-Aldrich, USA. First, 0.1 mM DPPH solution was prepared in 96% ethanol, and in the next step, it was mixed with nanoparticles and the standard antioxidant combination under BHT. After 45 min, the absorbance of the sample was read at a wavelength of 517 nm. To calculate the concentration required to inhibit 50% of antioxidant activity (IC50) for nanoparticles and BHT, the experiment was carried out in eleven different concentrations (1–1000 µg/ml) of these two substances. Each experiment was done in three repetitions and the average values obtained were used for calculations. DPPH radical inhibition percentage was obtained by the following formula [Citation49, Citation50]:
3. Results and discussion
3.1. Chemical characterization of ZnONPs@ C. rotundus
The XRD diffraction patterns of ZnONPs@ C. rotundus was used to evaluate the NPs crystallinity. The pattern of the diffractogram is shown in . The formation of nanoparticles was confirmed in the result. Despite the small size of ZnONPs@ C. rotundus, the pattern of XRD indicated well crystallizing. The achieved data were compared with the standard database of ICDD PDF card no. 01-080-3002. The signals with 2θ values of 31.87, 33.60, 36.40, 47.98, 56.54, 63.07, and 68.03 are indexed as (100), (002), (101), (102), (110), (103), and (201) planes. The signals were matched to those of ZnO for the zinc NPs. The crystal size of ZnONPs@ C. rotundus was measured using X-ray diffraction and according to Scherer’s equation. The results revealed a 33.09 nm for ZnONPs@ C. rotundus crystal size. Various crystalline sizes according to XRD analysis for the biosynthesized ZnNPs using plant extracts. Instead of, 46.74, to 779.38 nm for Thymus vulgaris leaf extract [Citation46]; 28.24 nm for Solanum torvum extract [Citation47]; 16.7 and 15.0 nm for Vaccinium arctostaphylos fruits extract [Citation51]; 59 nm for Berberis vulgaris extract [Citation52]; and 27.9 nm and 21 nm have reported for Vaccinium arctostaphylos leaf extract [Citation53].
The morphology of ZnONPs@C. rotundus was assessed by the FE-SEM technique. presents the FE-SEM of ZnONPs@ C. rotundus. The images show the spherical shape of the nanoparticles with particle sizes in the range of 38.05 to 75.41 nm. According to the previous studies, the green synthesized metallic nanoparticles usually tended to aggregate [Citation47, Citation54–58]; The FE-SEM images presented this property for ZnONPs@ C. rotundus. In our review of literature, the size of zinc nanoparticles, which were synthesized using plant extract was in the range of 10 to 80 nm [Citation51, Citation59, Citation60].
The qualitative elemental analysis of ZnONPs@ C. rotundus was run using the EDX diagram (). The formation of ZnO was approved by the signals for zinc and oxygen (the peaks at 1.0 keV for ZnLα, below 9.0 keV for ZnKα, and around 9.5 keV for ZnKβ; the peak around 0.5 keV for OLα). The signals for green synthesized zinc NPs have been reported by other research groups [Citation47].
The UV–Vis. spectra of the green-synthetic nanoparticles of ZnONPs@ C. rotundus are shown in . The peaks at the wavelength of 276 and 388 nm approved the formation of ZnONPs@ C. rotundus. The bands are very close to those of zinc nanoparticles, which have green synthesized using Tecoma castanifolia extract [Citation58].
shows the FT-IR spectrum of ZnONPs@ C. rotundus. The formation of the zinc oxide is confirmed by the presence of the peaks at 437 and 532 cm−1 that belong to the vibration of Zn-O. Similar peaks with some differences in the wavenumber have been reported for green-synthetic ZnONPs by other research groups [Citation58, Citation59]. The other peaks in the FT-IR spectrum belong to the different functional groups of organic compounds in the extract of C. rotundus which play as the reducing agents in the green synthesis of ZnONPs@ C. rotundus. The presence of secondary metabolites such as phenolic, flavonoid, saponins, Quinones, and Terpenoids in C. rotundus extract has been reported previously [Citation61, Citation62]. The peaks in 3409 and 2929 cm−1 are related to O-H and aliphatic C-H stretching; the peaks from 1402 to 1643 cm−1 are corresponded to C = C and C = O stretching, and the peaks at 1242 and 1054 cm−1 could be ascribed to ‐C‐O and C-O-C stretching.
3.2. Cytotoxicity of ZnONPs@ C. rotundus
In the application of current common methods that are carried out on a large scale in the world, Medicines that are introduced into the body to destroy cancer cells in the desired area may be reduced in their effectiveness on the way to the cancerous tissue, and when they reach the desired location, their dose may be lower than the prescribed amount [Citation43–45]. That is why chemotherapy and radiotherapy courses must be repeated continuously for the patient so that more and more cancer cells are attacked and destroyed in each series. On the other hand, if nanoparticles enter the bloodstream as drug carriers, since they have nothing to do with normal body cells and do not spend their energy and drug dose on them, as a result, they completely reach the place affected by cancer and use all their medicinal capacity to fight and destroy cancer cells [Citation45–48]. These particles protect the transported drugs so that they are not changed during reaching the cancerous area and do not cause any toxicity or side effects in the treated person [Citation43–46]. Nanoparticles, with the ability to pass through biological barriers and barriers in the body, deliver the drug dose completely and perfectly to the desired area. Also, the duration of the presence of these particles in the patient’s blood is longer than in other cancer treatment methods [Citation47–49]. Therefore, there is no need to visit consecutively to receive medicine. In this way, the effect of drugs on cancer treatment can be significantly strengthened and increased. There are two types of approaches to delivering the desired drugs to cancer masses by nanoparticles, which include active and passive strategies [Citation48–50]. This type of targeting can be done according to the characteristics and properties of various tumors, taking into account the environmental conditions of tumor cells, pH of the environment, enzyme activity, temperature changes, or through direct delivery of the drug to the cancer site [Citation45–48]. Blood vessels in the area around tumor formation are more permeable than in normal conditions. This condition, which is known as EPR or increased tumor permeability, makes the nanoparticles that have been sent to this place easily reach the cancer cells by passing through the vessel wall and begin to destroy them [Citation43–47]. The difference between the active method and the passive targeting is that in this method, active cell factors such as antibodies, peptides, and nanorobots are attached to carrier molecules which are nanoparticles and after EPR, they reach the cancer site and settle there [Citation49, Citation50, Citation63]. By connecting the ligands attached to the nanoparticles to the receptors located on the antigen surface of the cancer cells, this compound enters the abnormal cell and begins to destroy it [Citation43–47].
In recent research, the therapeutic and toxicity effects of ZnONPs@ C. rotundus on cancer (HLC-1) and normal (HUVEC) cells were determined by MTT methods ( and ). The IC50 of ZnONPs@ C. rotundus was 234 µg/mL against HLC-1 cell lines, respectively ( and ).
3.3. Antioxidant activity of ZnONPs@ C. rotundus
In a recent study, the effects of BHT and ZnONPs@ C. rotundus on the common free radicals (DPPH) were evaluated (). In the antioxidant test, the IC50 of ZnONPs@ C. rotundus and BHT against DPPH were 41 and 24 µg/mL, respectively.
4. Conclusion
The green synthesis of zinc nanoparticles was carried out using an aqueous extract of C. rotundus. The nanoparticles were characterized using UV-Visible, FT-IR, XRD, FE-SEM, and EDS. The nanoparticles were formed in a spherical morphology with a particle range size from 38.05 to 75.41 nm which is well known as a sufficient size for synthetic nanoparticles.
The viability of lung well-differentiated bronchogenic adenocarcinoma cell line reduced dose-dependently in the presence of ZnONPs@ C. rotundus. The IC50 of ZnONPs@ C. rotundus was 234 µg/mL against the HLC-1 cell line, respectively. The ZnONPs@ C. rotundus showed the best antioxidant activities against DPPH. So, the findings of the recent research show that biologically synthesized ZnONPs@ C. rotundus might be used to cure lung well-differentiated bronchogenic adenocarcinoma.
Data availability statement
Data is available on request from the authors.
Disclosure statement
No potential conflict of interest was reported by the authors.
References
- Wang F, Song X, Ma S, et al. The treatment role of Cyperus rotundus L. to triple-negative breast cancer cells. Biosci Rep. 2019;39(6):1–26.
- Kamala A, Middha SK, Karigar CS. Plants in traditional medicine with special reference to Cyperus rotundus L.: a review. 3 Biotech. 2018;8(7):1–11.
- Pirzada AM, Ali HH, Naeem M, et al. Cyperus rotundus L.: traditional uses, phytochemistry, and pharmacological activities. J Ethnopharmacol. 2015;174:540–560.
- Sung B, Prasad S, Yadav VR, et al. Cancer cell signaling pathways targeted by spice-derived nutraceuticals. Nutr Cancer. 2012;64(2):173–197.
- Sivapalan SR, Jeyadevan P. Physico-chemical and phytochemical study of rhizome of Cyperus rotundus linn. IJPPT. 2017;1(2):42–46.
- Zhou Z, Yin W. Two novel phenolic compounds from the rhizomes of Cyperus rotundus L. Molecules. 2012;17(11):12636–12641.
- Harborne JB, Williams CA, Wilson KL. Flavonoids in leaves and inflorescences of Australian cyperus species. Phytochemistry. 1982;21(10):2491–2507.
- Kamala A, Middha SK, Gopinath C, et al. In vitro antioxidant potentials of Cyperus rotundus L. rhizome extracts and their phytochemical analysis. Pharmacogn Mag. 2018;14(54):261–267.
- Wu S, Powers S, Zhu W, et al. Substantial contribution of extrinsic risk factors to cancer development. Nature. 2016;529(7584):43–47.
- Torre LA, Bray F, Siegel RL, et al. Global cancer statistics, 2012. CA Cancer J Clin. 2015;65(2):87–108.
- Fock MM. The epidemiology and prevention of gastric cancer. Aliment Pharmacol Ther. 2014;40(3):250–260.
- Szymanski MS, Porter RA. Preparation and quality control of silver nanoparticle-antibody conjugate for use in electrochemical immunoassays. J Immunol Methods. 2013;387(1–2):262–269.
- Prabhu V, Uzzaman S, Grace V, et al. Nanoparticles in drug delivery and cancer therapy: the giant rats tail. JCT. 2011;02(03):325–334.
- Abbai R, Mathiyalagan R, Markus J, et al. Green synthesis of multifunctional silver and gold nanoparticles from the Oriental herbal adaptogen: Siberian ginseng. Int J Nanomed. 2016;11:3131–3143.
- Wan G, Ruan I, Yin Y, et al. Effects of silver nanoparticles in combination with antibiotics on the resistant bacteria Acinetobacter baumannii. Int J Nanomed. 2016;11:3789–3800.
- Farah MA, Ali MA, Chen S-M, et al. Silver nanoparticles synthesized from Adenium obesum leaf extract induced DNA damage, apoptosis and autophagy via generation of reactive oxygen species. Colloids Surf B Biointerfaces. 2016;141:158–169.
- Huang L, Dai T, Xuan Y, et al. Synergistic combination of chitosan acetate with nanoparticle silver as a topical antimicrobial: efficacy against bacterial burn infections. Antimicrob Agents Chemother. 2011;55(7):3432–3438.
- Huang Y, Zhu C, Xie R, et al. Green synthesis of nickel nanoparticles using Fumaria officinalis as a novel chemotherapeutic drug for the treatment of ovarian cancer. J Exp Nanosci. 2021;16(1):368–381.
- Zhai C, Shi C, Hu Y, et al. Anti-breast carcinoma effects of green synthesized tin nanoparticles from calendula officinalis leaf aqueous extract inhibits MCF7, Hs 319. T, and MCF10 cells proliferation. J Exp Nanosci. 2022;17(1):351–361.
- Jia Z, Sun H, Gu Q. Preparation of Ag nanoparticles with triethanolamine as reducing agent and their antibacterial property. Colloid Surf A Physicochem Eng Asp. 2013;419:174–179.
- Philip D. Green synthesis of gold and silver nanoparticles using Hibiscus rosa. Physica E Low-Dimens Syst Nanostruct. 2010;42(5):1417–1424.
- Ashrafi SJ, Rastegar MF, jafarpour B, et al. Use of plant pathogenic fungi Fusarium moniliforme for biosynthesis of silver nano particles with emphasis to time. Eur Cell Mater. 2010;20(3):1–8.
- Khatami M, Pourseyedi S. Phoenix dactylifera (date palm) pit aqueous extract mediated novel route for synthesis high stable AgNPs with high antifungal and antibacterial activity. IET Nanobiotechnol. 2015;9(4):184–190.
- Mishra A, Sardar M. Alpha-amylase mediated synthesis of silver nanoparticles. Sci Adv Mater. 2012;4(1):143–146.
- Jing Y, Wang H, Wang Z, et al. Interaction between antitumor drug and silvernanoparticles:combined fluorescence and surface enhanced Raman scattering study. Chin Opt Lett. 2009;7(10):894–897.
- Gu J, Aidy A, Goorani S. Anti-human lung adenocarcinoma, cytotoxicity, and antioxidant potentials of copper nanoparticles green-synthesized by Calendula officinalis. J Exp Nanosci. 2022;17(1):285–296.
- Sankar R, Karthik A, Prabu A, et al. Origanum vulgare mediated biosynthesis of silver nanoparticles for its antibacterial and anticancer activity. Colloids Surf B Biointerfaces. 2013;108:80–84.
- Daoud S, Alqahtani MAM, Alkhalifah DHM, et al. Biosynthesis of silver nanoparticles using Salvia officinalis extract and assessment of their antibacterial activity. Int J Curr Res. 2015;7:21548–21552.
- Prabhu D, Arulvasu C, Babu G, et al. Biologically synthesized green silver nanoparticles from leaf extract of Vitex negundo L. induce growth-inhibitory effect on human Colon cancer cell line HCT15. Process Biochem. 2013;48(2):317–324.
- Abad MJ, Bedoya LM, Apaza L, et al. The artemisia L. Genus: a review of bioactive essential oils. Molecules. 2012;17(3):2542–2566.
- Vijay Kumar PPN, Pammi SVN, Kollu P, et al. Green synthesis and characterization of silver nanoparticles using Boerhaavia diffusa plant extract and their antibacterial activity. Ind Crops Prod. 2014;52:562–566.
- Singh H, Du J, Yi TH. Green and rapid synthesis of silver nanoparticles using Borago officinalis leaf extract: anticancer and antibacterial activities. Artif Cells Nanomed Biotechnol. 2017;45(7):1310–1316.
- Devi JS, Bhimba BV. Anticancer activity of silver nanoparticles synthesized by the seaweed Ulva lactuca in vitro. Sci Rep. 2012;1(4):242–250.
- Salehi S, Shandiz SAS, Ghanbar F, et al. Phytosynthesis of silver nanoparticles using Artemisia marschalliana sprengel aerial parts extract and assessment of their antioxidant, anticancer, and antibacterial properties. Int J Nanomed. 2016;11:1835–1846. ():
- (a) Zangeneh MM, Pooyanmehr M, Zangeneh A. Biochemical, histopathological, and pharmacological evaluations of cutaneous wound healing properties of Quercus brantii ethanolic extract ointment in male rats. Comp Clin Pathol. 2019;28(5):1483–1493.
- Zangeneh MM, Zangeneh A. Novel green synthesis of Hibiscus sabdariffa flower extract conjugated gold nanoparticles with excellent anti‐acute myeloid leukemia effect in comparison to daunorubicin in a leukemic rodent model. Appl Organometal Chem. 2020;34:5271.
- Jalalvand AR, Zhaleh M, Goorani S, et al. Chemical characterization and antioxidant, cytotoxic, antibacterial, and antifungal properties of ethanolic extract of Allium Saralicum R.M. Fritsch leaves rich in linolenic acid, methyl ester. J Photochem Photobiol B. 2019;192:103–112.
- Seydi N, Mahdavi B, Paydarfard S, et al. Preparation, characterization, and assessment of cytotoxicity, antioxidant, antibacterial, antifungal, and cutaneous wound healing properties of titanium nanoparticles using aqueous extract of Ziziphora clinopodioides lam leaves characterization and biological effects of TiNPs@ziziphora. Appl Organometal Chem. 2019;33:e5009.
- Darvishi E, Kahrizi D, Arkan E. Comparison of different properties of zinc oxide nanoparticles synthesized by the green (using Juglans regia L. leaf extract) and chemical methods. J Mol Liq. 2019;286:110831.
- Sukri SNAM, Shameli K, Wong MMT, et al. Cytotoxicity and antibacterial activities of plant-mediated synthesized zinc oxide (ZnO) nanoparticles using Punica granatum (pomegranate) fruit peels extract. J Mol Struct. 2019;1189:57–65.
- Chen L, Batjikh I, Hurh J, et al. Green synthesis of zinc oxide nanoparticles from root extract of Scutellaria baicalensis and its photocatalytic degradation activity using methylene blue. Optik. 2019;184:324–329.
- Djurišić AB, Ng AMC, Chen XY. ZnO nanostructures for optoelectronics: material properties and device applications. Prog Quantum Electron. 2010;34(4):191–259.
- Kumar N, Mittal H, Reddy L, et al. Morphogenesis of ZnO nanostructures: role of acetate (COOH−) and nitrate (NO 3−) ligand donors from zinc salt precursors in synthesis and morphology dependent photocatalytic properties. RSC Adv. 2015;5(48):38801–38809.
- Azizi S, Ahmad MB, Namvar F, et al. Green biosynthesis and characterization of zinc oxide nanoparticles using brown marine macroalga Sargassum muticum aqueous extract. Mater Lett. 2014;116:275–277.
- Chaudhary A, Rahul SN. Antibacterial activity of Punica granatum (pomegranate) fruit peel extract against pathogenic and drug resistance bacterial strains. Int J Curr Microbiol App Sci. 2017;6(12):3802–3807.
- Khan R, Khan M, Ansari A, et al. Flower-shaped ZnO nanoparticles synthesized by a novel approach at near-room temperatures with antibacterial and antifungal properties. IJN. 2014;9:853.
- Mahdavi B, Saneei S, Qorbani M, et al. Ziziphora clinopodioides lam leaves aqueous extract mediated synthesis of zinc nanoparticles and their antibacterial, antifungal, cytotoxicity, antioxidant, and cutaneous wound healing properties under in vitro and in vivo conditions. Appl Organometal Chem. 2019;33(11):e5164.
- Yan W, Liu Y, Mansooridara S, et al. Chemical characterization and neuroprotective properties of copper nanoparticles green-synthesized by Nigella sativa L. seed aqueous extract against methadone-induced cell death in adrenal phaeochromocytoma (PC12) cell line. J Exp Nanosci. 2020;15(1):280–296.
- Zangeneh MM, Zangeneh A, Tahvilian R, et al. Antidiabetic, hematoprotective and nephroprotective effects of the aqueous extract of Falcaria vulgaris in diabetic male mice. Arch Biol Sci (Beogr). 2018;70(4):655–664.
- Hamelian M, Zangeneh MM, Amisama A, et al. Green synthesis of silver nanoparticles using thymus kotschyanus extract and evaluation of their antioxidant, antibacterial and cytotoxic effects. Appl Organometal Chem. 2018;32(9):e4458.
- Thema F, Manikandan E, Dhlamini M, et al. Green synthesis of ZnO nanoparticles via agathosma betulina natural extract. Mater Lett. 2015;161:124–127.
- Zare M, Namratha K, Thakur M, et al. Biocompatibility assessment and photocatalytic activity of bio-hydrothermal synthesis of ZnO nanoparticles by thymus vulgaris leaf extract. Mater Res Bull. 2019;109:49–59.
- Ezealisiji KM, Siwe-Noundou X, Maduelosi B, et al. Green synthesis of zinc oxide nanoparticles using Solanum torvum (L) leaf extract and evaluation of the toxicological profile of the ZnO nanoparticles–hydrogel composite in Wistar albino rats. Int Nano Lett. 2019;9(2):99–107.
- Bayrami A, Parvinroo S, Habibi-Yangjeh A, et al. Bio-extract-mediated ZnO nanoparticles: microwave-assisted synthesis, characterization and antidiabetic activity evaluation. Artif Cells Nanomed Biotechnol. 2018;46(4):730–739.
- Anzabi Y. Biosynthesis of ZnO nanoparticles using barberry (Berberis vulgaris) extract and assessment of their physico-chemical properties and antibacterial activities. Green Process Synth. 2018;7(2):114–121.
- Bayrami A, Alioghli S, Pouran SR, et al. A facile ultrasonic-aided biosynthesis of ZnO nanoparticles using Vaccinium arctostaphylos L. leaf extract and its antidiabetic, antibacterial, and oxidative activity evaluation. Ultrason Sonochem. 2019;55:57–66.
- Baghayeri M, Mahdavi B, Hosseinpor‐Mohsen Abadi Z, et al. Green synthesis of silver nanoparticles using water extract of Salvia leriifolia: antibacterial studies and applications as catalysts in the electrochemical detection of nitrite. Appl Organometal Chem. 2018;32(2):e4057.
- Sharmila G, Thirumarimurugan M, Muthukumaran C. Green synthesis of ZnO nanoparticles using Tecoma castanifolia leaf extract: characterization and evaluation of its antioxidant, bactericidal and anticancer activities. Microchem J. 2019;145:578–587.
- Dobrucka R, Dlugaszewska J, Kaczmarek M. Cytotoxic and antimicrobial effects of biosynthesized ZnO nanoparticles using of Chelidonium majus extract. Biomed Microdevices. 2017;20(1):5.
- Saha R, Karthik S, Balu KS, et al. Influence of the various synthesis methods on the ZnO nanoparticles property made using the bark extract of Terminalia arjuna. Mater Chem Phys. 2018;209:208–216.
- Babiaka SB, Moumbock AF, Günther S, et al. Natural products in Cyperus rotundus L. (Cyperaceae): an update of the chemistry and pharmacological activities. RSC Adv. 2021;11(25):15060–15077.
- Parvez MK, Al-Dosari MS, Arbab AH, et al. The in vitro and in vivo anti-hepatotoxic, anti-hepatitis B virus and hepatic CYP450 modulating potential of Cyperus rotundus. Saudi Pharm J. 2019;27(4):558–564.
- Bai J, Gongsun X, Xue L, et al. Introducing a modern chemotherapeutic drug formulated by iron nanoparticles for the treatment of human lung cancer. J Exp Nanosci. 2021;16(1):397–409.