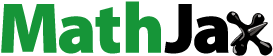
Abstract
Regarding applied, facile, green chemical research, a bio-inspired approach is being reported for the sonochemical synthesis of Ag nanoparticles by Aloe vera gel extract as a natural reducing/stabilising and solid support agent without using any toxic and harmful reagent. The biosynthesized Ag NPs/A. vera gel bionanocomposite was characterised by advanced physicochemical techniques like ultraviolet-visible (UV–Vis), Scanning Electron Microscopy (SEM), Transmission Electron Microscopy (TEM), Energy Dispersive X-ray spectroscopy (EDX), X-ray photoelectron spectroscopy (XPS), X-ray Diffraction (XRD) and elemental mapping study. It has been established that Aloe vera gel-stabilized silver nanoparticles have a spherical shape with a mean diameter of around 35–40 nm. To investigate the antioxidant potentials of Ag NPs/A. vera gel, one of the common antioxidant techniques i.e. DPPH was used. The results of DPPH test proved the excellent antioxidant properties of Ag NPs/A. vera gel in comparison to the positive control used i.e. butylated hydroxytoluene. The IC50 of Ag NPs/A. vera gel was 42 µg/mL in the antioxidant test. Thereafter, on having a significant IC50 value in radical scavenging assay, we extended the bio-application of the desired nanocomposite in the anticancer study. To survey the cytotoxicity and anti-human cervical carcinoma effects of Ag NPs/A. vera gel, MTT assay was used on CC1-PI19, SiHa, HeLa and HNCF-PI52 cell lines. The IC50 of the Ag NPs/A. vera gel was 16, 13, 15 and 11 µg/mL against CC1-PI19, SiHa, HeLa and HNCF-PI52 cell lines, respectively. As mentioned, the Ag NPs/A. vera gel had significant antioxidant and anti-human cervical cancer effects. After approving the above results in the clinical trial studies, the Ag NPs/A. vera gel can be used as a chemotherapeutic drug for the treatment of several types of cervical cancers. According to the above results, the significant anti-cervical cancer effects of Ag NPs/A. vera gel are confirmed.
1. Introduction
In the application of current common methods that are carried out on a large scale in the world, medicines that are introduced into the body with the aim of destroying cancer cells in the desired area may be reduced in their effectiveness on the way to the cancerous tissue, and when they reach the desired location, their dose may be lower than the prescribed amount [Citation1–5]. That is why chemotherapy and radiotherapy courses must be repeated continuously for the patient so that more and more cancer cells are attacked and destroyed in each series [Citation6–9]. In the last decade, following research and obtaining more information about tumours and types of cancer, a new method called nanotechnology has been proposed to treat cancers [Citation10–13]. Nanotechnology is one of the effective and less dangerous solutions with more benefits than chemotherapy and radiotherapy for patients, which is part of the new cancer treatments [Citation14–17]. In this method, anti-cancer drugs are placed on very small particles of engineered compounds (with a size of nanometers) and are directed into the bloodstream. The interesting and key point of how this method works is that when the materials reach the nanoscale, they exhibit different behaviour and characteristics that were not seen before [Citation18–23]. Also, they show unique electrical, optical and structural properties that favour our desired treatment process in this method. The use of nanotechnology for cancer treatment has attracted much attention in recent years. This technology can provide unique methods for the prediction, prevention and early treatment of cancer [Citation23–28]. Since nanoparticles are 102 to 103 times smaller than tumour cells, they can travel easily by blood and link with tumor-specific proteins on the cancer cells inside and surface. Therefore, their use as drug-delivery carriers to cancer cells has attracted much attention [Citation29–33]. Drugs encapsulated in targeted nanoparticles have the potential to ameliorate the safety and efficacy of existing drugs. Also, at present, multipurpose nanocarriers have emerged to help radiation therapy and chemotherapy [Citation33–35].
Nanoparticles are designed in such a way that they can carry a higher dose of medicine with them and deliver it to the target area that is affected by cancer. In fact, these particles are not a threat to healthy body cells and only affect cancer cells and destroy them [Citation31–35]. This method is considered a targeted treatment that attacks only the target cells and acts selectively. By this method on several patients, positive and satisfactory results have been obtained, which can be considered as part of the great developments of medical science in the field of cancer treatment [Citation32–34].
Synthesis of metal nanoparticles by green chemistry due to its environmental begin approach has been widely investigated using extracts of plants and microbes [Citation36,Citation37]. Biologically active plant compounds and polysaccharides have hydroxyl and phyto-compounds that are used as stabilizing and reducing agents for metal NPs synthesis [Citation36–38]. Aloe vera due to its richness in biomolecules has medicinal properties and is widely investigated in current research [Citation37,Citation38]. The gel of Aloe vera contains cellulose, hemicelluloses, pectin, flavonoids, polyphenols, quinones, citric acid, ascorbic acid, acetic acid, etc. [Citation39–41]. In the present work, Ag NPs were synthesised by extract of Aloe vera gel under ultrasonic conditions to treat the cervical cancer.
2. Experimental
2.1. Materials and characterisation
All of the chemical compounds for nanoparticle synthesis were purchased from Sigma. In the instrumental section, the morphology, particle size, and elemental mapping analysis of the nanocomposite were characterised using a FESEM-TESCAN MIRA3 microscope equipped with EDX. X-ray diffraction (XRD) analysis on a Bruker D8 Advance diffractometer with CuKα radiation at 40 kV and 20 mA were evaluated to establish the crystalline structures of the composites. Transmission electron microscopy (TEM) analysis was applied on a Phillips CM10 microscope at an accelerating voltage of 200 kV.
2.2. Preparation of extract from Aloe vera
The extract of Aloe vera by finely cutting the Aloe vera leaf was prepared. 5.0 grams of fresh weight of gel was mixed with 50 mL of DI-H2O (1:10) and stirred at 60 °C for 20 min. Then, the mixture was cooled to room temperature and filtered by vacuum filtration. Finally, the extract was stored at 4 °C for the next step.
2.3. Biosynthesis of Ag NPs/A. vera gel bionanocomposite
Silver nanoparticles were prepared by adding 10 ml of aqueous Aloe vera gel extract to 50 ml of aqueous 1 mMAgNO3 solution by constant sonication (60 Hz) at 60 °C for 20 min. By the change of colour from watery yellow to brown colour, the reduction of Ag+ to Ag° is confirmed. The synthesised Ag NPs/A. vera gel were purified by centrifuge (10,000 rpm) for 10 min.
2.4. DPPH assay protocol
In this method, 1 ml of different concentrations of the Ag NPs/A. vera gel bionanocomposite (1–1000 µg/ml) was mixed with 1 ml of 300 µM DPPH) and then the final volume of the mixture with methanol reached 4000 µl. Then the falcons were vortexed and kept for 30 min. The absorbance was read at a wavelength of 517 nm. DPPH radical inhibition percentage was calculated by the following equation:
Here, A0 is the absorption of the control (containing all reactive components without the sample and AS is the absorption of the sample) [Citation42].
2.5. MTT assay protocol
In this study, the anticancer effects of Ag NPs/A. vera gel bionanocomposite samples against the cervical cancer CC1-PI19, SiHa, HeLa and HNCF-PI52 cell lines were investigated.
The MTT method was used to investigate cytotoxicity. The cells were cultured in a culture medium containing 10% FBS and streptomycin (thousand micrograms/ml) and penicillin (hundred units/ml) antibiotics. The cultures were incubated at 37 °C and in the presence of 5% CO2. To perform the MTT test, 5000 cells were placed in each house from a 96-well plate. After 24 h, concentrations of nanoparticles solvent were prepared and treated on cell lines for 24 h. After the above time, the contents of the wells were carefully removed and 200 µl of MTT dye was added to them. The microplate was placed in a 37 °C incubator for 4 h, after which the supernatant was drained and 200 µl of DMSO solution was added to each well. In the next step, the samples absorbance was read with a microplate reader at a wavelength of 570 nm, and the percentage of living cells was calculated relative to the optical absorbance of the control cells [Citation43].
In this study, SPSS22 software was used to check the qualitative variables, compare the percentage of variables and analyse the data. SPSS version 22 statistical software was used to analyse all data. Friedman’s non-parametric test was used to check the difference between extracts, nanoparticles, and the combined state, and the paired test was used to check the difference between each one separately. A value of less than 0.05 was considered significant.
3. Results and discussion
In the current study, the green synthesis of AgNPs has been described using an aqueous gel extract of Aloe vera. A visual colour change from watery yellow to brown colour confirmed the successful green formation of Ag NPs. Next, the reaction medium containing AgNPs was analysed by UV–Vis spectrum that showed a broad absorbance at 445 nm which is assigned to AgNPs (). The reducing action of Aloe vera gel extract is indicated in this reaction by the successful synthesis of silver nanoparticles.
The surface morphology of the Ag NPs/A. vera gel was analysed by the FE-SEM image (). It shows silver nanoparticles with quasi-spherical shape. TEM analysis () provided information about the size and morphology of the desired Ag NPs/A. vera gel. The formed nanocomposite was found to have an average size of 35–40 nm and spherical shape that capped by gel extract without aggregation. Moreover, the histogram of particle size distribution indicated that size is approximately 36.5 nm for Ag NPs ().
The chemical component of the Ag NPs/A. vera gel bionanocomposite was investigated from the EDX spectrum and the related output is represented in . It displays two strong and major signals at 3.05 keV, ascribed to Ag NPs. However, the first one is related to the Au vapour deposited over the sample prior to analysis. The minor and small peaks observed in the lower region correspond to the C and O species, indicating the gel extract modification.
The constitutional study was further extended in elemental mapping analysis, in addition to the EDX study. represents the corresponding X-ray scan image of the SEM analysis. This validates the presence of C and Ag species, which are uniformly distributed over the surface.
Again, crystalline nature, phase behaviour and the purity of Ag NPs/A. vera gel was studied by XRD data (). The first phase in the 2θ region (5–15°) was assigned to Aloe vera gel extract as non-crystalline phase. Also, the diffraction peaks at 2θ = 38.2°, 44.3°, 64.5° and 77.3° corresponds to the diffraction on fcc silver (111), (200), (220) and (311) planes (JCPDS No.87-0717) confirmed the Ag crystalline phases.
XPS analysis was applied to calculate the Ag species oxidation state (). The results show the presence of an intense doublet at a binding energy (BE) of 374.05 and 368.01 eV assigned to Ag(0), corresponding, respectively, to the Ag 3d3/2 and Ag 3d5/2 peaks. It shows that all of the Ag exist in the reduced form, confirming the successful conversion of Ag(I) to Ag(0) NPs by Aloe vera gel extract [Citation44].
In the recent study, the effects of BHT and Ag NPs/A. vera on the common free radicals (DPPH) were evaluated (). In the antioxidant test, the IC50 of Ag NPs/A. vera and BHT against DPPH were 31 and 42 µg/mL, respectively.
A living cell is a collection of microscopic components with specific functions and functions. The plasma membrane surrounds the cell contents and is in contact with the external environment. The selective permeability of the membrane maintains the stability of the cell with the help of various transport mechanisms [Citation45–48]. Small-sized nanoparticles easily pass through the membrane and due to their special surface properties, they interact with important cellular components such as mitochondria, lysosomes and nuclei. The continuity of the structure and maintenance of the function of cells is often determined by biological macromolecules such as carbohydrates, lipids and proteins. The structure of biomolecules changes under the influence of interaction with various types of nanoparticles [Citation46–48].
Molecular oxygen dissolved in biological fluids is transformed into singlet oxygen under the influence of light energy required for biological transfer reactions. Superoxide radical is formed by a reduction reaction of oxygen molecules. This reaction can be done as a result of the redox cycle, or enzymatically with the catalysis of NADPH oxidase, and also as a byproduct of enzymatic reactions with Xanthine oxidase and a byproduct of the electron transport chain in mitochondria [Citation48–51]. By upregulating the xanthine oxidase and NADPH oxidase enzymes, nanomaterials increase the production of superoxide radicals in some cells such as macrophages and neutrophils and trigger inflammatory reactions. The superoxide anion is suddenly converted to hydrogen peroxide (H2O2) by the catalysis of the superoxide dismutase enzyme and with the help of copper, manganese or zinc as a cofactor. The dissolution of nanomaterials based on iron or copper catalyses the formation of active oxygen species and through the Fenton reaction, leads to the production of hydroxyl (OH) and peroxyl (OOH) free radicals [Citation49–52]. For example, TiO2 nanoparticles used in sunscreens produce singlet oxygen and superoxide under the influence of light rays. An increase in the level of ROS leads to inflammatory responses such as an increase in cells with polymorphous nuclei and disturbances in the phagocytosis process of macrophages in some model animals such as rodents [Citation52–54]. Oxidative stress is caused by the predominance of free radicals on antioxidants and is one of the main mechanisms of toxicity of most metal nanoparticles such as gold, zinc oxide, and silver. Oxidative stress by regulating redox-sensitive transcription factors activates some kinase enzymes and intermediate proteins of inflammatory reactions and causes tissue damage such as damage to the cell membrane, genetic material and biological macromolecules [Citation49–53]. At very high levels, disruption of the signalling pathways inside the cell leads to apoptosis and cell necrosis. Reactive oxygen species disrupt the function of the central nervous system by peroxidizing the unsaturated fatty acids of neuronal cells. Clogging of blood vessels, blood pressure and re-narrowing of arteries after angioplasty due to ROS cause disorders of the cardiovascular system. Mitochondria are one of the ROS production main sources through the electron transport respiratory chain [Citation51–54]. Several clinical syndromes such as stroke, Duchenne muscular dystrophy, cardiac conduction defects due to ROS oxidative attack and mitochondrial DNA double-strand break occur. By producing reactive oxygen species in cancer cells, nanoparticles create changes in the chemical structure of histones or other proteins that are effective in shaping the structure of DNA [Citation50–54]. Unfolding of the helical structure of DNA disrupts gene expression and malfunction of regulation of cellular function of cancer cells. In addition to ROS toxicity, some nanoparticles use other effective mechanisms for cell damage by releasing metal ions, accumulating in some cellular components, and interacting with nuclear components. In general, the cell damage mechanisms by nanoparticles include (1) membranes physical damage, (2) structural changes in the cell skeleton components, (3) disturbance in oxidative and transcription damage, (4) mitochondria damage, (5) dysfunction of lysosome, (6) ROS production, (7) membrane proteins dysfunction, and (8) mediators and inflammatory factors synthesis [Citation50–53].
In recent research, the therapeutic and toxicity effects of Ag NPs/A. veraon cancer (HLC-1) and normal (HUVEC) cells were determined by MTT methods ( and ).
Figure 10. The anti-cervical carcinoma properties of Ag NPs/A. vera against CC1-PI19 (I), SiHa (II), HeLa (III) and HNCF-PI52 (IV) cell lines.
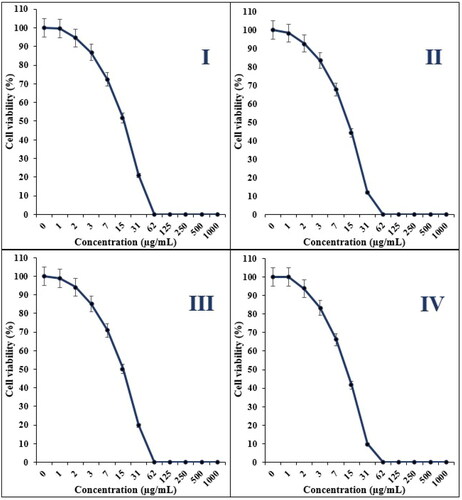
The IC50 of Ag NPs/A. vera was 16, 13, 15 and 11 µg/mL against CC1-PI19, SiHa, HeLa and HNCF-PI52 cell lines, respectively ( and ).
4. Conclusion
In conclusion, we introduce herein the architecture and sustainable synthesis of Aloe vera gel extract fabricated Ag NPs. Aloe vera gel extract has been used in the bio-templated synthesis of Ag NPs as reducing/stabilizing agent. The structural and physicochemical features were assessed through a broad range of analytical methods. The silver nanoparticles were prepared in spherical shape with fcc structure. The viability of cervical cancer cell lines reduced dose-dependently in the presence of Ag NPs/A. vera. The IC50 of the Ag NPs/A. vera gel were 16, 13, 15 and 11 µg/mL against CC1-PI19, SiHa, HeLa and HNCF-PI52 cell lines, respectively. The Ag NPs/A. vera showed the best antioxidant activities against DPPH.
Disclosure statement
No potential conflict of interest was reported by the authors.
Data availability statement
Data available on request from the authors.
Additional information
Funding
References
- Sivapalan SR, Jeyadevan P. Physico-chemical and phytochemical study of rhizome of Cyperus rotundus Linn. IJPPT. 2017;1(2):42–46.
- Sung B, Prasad S, Yadav VR, et al. Cancer cell signaling pathways targeted by spice-derived nutraceuticals. Nutr Cancer. 2012;64(2):173–197.
- Pirzada AM, Ali HH, Naeem M, et al. Cyperus rotundus L.: traditional uses, phytochemistry, and pharmacological activities. J Ethnopharmacol. 2015;174:540–560.
- Kamala A, Middha SK, Karigar CS. Plants in traditional medicine with special reference to Cyperus rotundus L.: a review. 3 Biotech. 2018;8(7):1–11.
- Wang F, Song X, Ma S, et al. The treatment role of Cyperus rotundus L. to triple-negative breast cancer cells. Biosci Rep. 2019;39(6):1–26.
- Wu S, Powers S, Zhu W, et al. Substantial contribution of extrinsic risk factors to cancer development. Nature. 2016;529(7584):43–47.
- Kamala A, Middha SK, Gopinath C, et al. In vitro antioxidant potentials of Cyperus rotundus L. rhizome extracts and their phytochemical analysis. Pharmacogn Mag. 2018;14(54):261–267.
- Harborne JB, Williams CA, Wilson KL. Flavonoids in leaves and inflorescences of Australian Cyperus species. Phytochemistry. 1982;21(10):2491–2507.
- Zhou Z, Yin W. Two novel phenolic compounds from the rhizomes of Cyperus rotundus L. Molecules. 2012;17(11):12636–12641.
- Prabhu V, Uzzaman S, Grace V, et al. Nanoparticles in drug delivery and cancer therapy: the giant rats tail. JCT. 2011;02(03):325–334.
- Szymanski MS, Porter RA. Preparation and quality control of silver nanoparticle-antibody conjugate for use in electrochemical immunoassays. J Immunol Methods. 2013;387(1-2):262–269.
- Fock MM. The epidemiology and prevention of gastric cancer. Aliment Pharmacol Ther. 2014;40(3):250–260.
- Torre LA, Bray F, Siegel RL, et al. Global cancer statistics, 2012. CA Cancer J Clin. 2015;65(2):87–108.
- Huang L, Dai T, Xuan Y, et al. Synergistic combination of chitosan acetate with nanoparticle silver as a topical antimicrobial: efficacy against bacterial burn infections. Antimicrob Agents Chemother. 2011;55(7):3432–3438.
- Farah MA, Ali MA, Chen S-M, et al. Silver nanoparticles synthesized from Adenium obesum leaf extract induced DNA damage, apoptosis and autophagy via generation of reactive oxygen species. Colloids Surf B Biointerfaces. 2016;141:158–169.
- Wan G, Ruan I, Yin Y, et al. Effects of silver nanoparticles in combination with antibiotics on the resistant bacteria Acinetobacter baumannii. Int J Nanomedicine. 2016;11:3789–3800.
- Abbai R, Mathiyalagan R, Markus J, et al. Green synthesis of multifunctional silver and gold nanoparticles from the Oriental herbal adaptogen: Siberian ginseng. Int J Nanomedicine. 2016;11:3131–433143.
- Khatami M, Pourseyedi S. Phoenix dactylifera (date palm) pit aqueous extract mediated novel route for synthesis high stable AgNPs with high antifungal and antibacterial activity. IET Nanobiotechnol. 2015;9(4):184–190.
- Ashrafi SJ, Rastegar MF, jafarpour B, et al. Use of plant pathogenic fungi Fusarium moniliforme for biosynthesis of silver nano particles with emphasis to time. Eur Cell Mater. 2010;20(3):1–8.
- Philip D. Green synthesis of gold and silver nanoparticles using Hibiscus rosa sinensis. Phys E Low-Dimens Syst Nanostruct. 2010;42(5):1417–1424.
- Jia Z, Sun H, Gu Q. Preparation of Ag nanoparticles with triethanolamine as reducing agent and their antibacterial property. Colloid Surf A Physicochem Eng Asp. 2013;419:174–179.
- Mishra A, Sardar M. Alpha-amylase mediated synthesis of silver nanoparticles. Sci Adv Mater. 2012;4(1):143–146.
- Jing Y, Wang H, Wang Z, et al. Interaction between antitumor drug and silvernanoparticles:combined fluorescence and surface enhanced Raman scattering study. Chin Opt Lett. 2009;7(10):894–897.
- Vijay Kumar PPN, Pammi SVN, Kollu P, et al. Green synthesis and characterization of silver nanoparticles using Boerhaavia diffusa plant extract and their antibacterial activity. Ind Crops Prod. 2014;52:562–566.
- Abad MJ, Bedoya LM, Apaza L, et al. The Artemisia L. Genus: a review of bioactive essential oils. Molecules. 2012;17(3):2542–2566.
- Prabhu D, Arulvasu C, Babu G, et al. Biologically synthesized green silver nanoparticles from leaf extract of Vitex negundo L. induce growth-inhibitory effect on human colon cancer cell line HCT15. Process Biochem. 2013;48(2):317–324.
- Daoud S, Alqahtani MAM, Alkhalifah DHM, et al. Biosynthesis of silver nanoparticles using salvia officinalis extract and assessment of their antibacterial activity. Int J Curr Res. 2015;7:21548–21552.
- Sankar R, Karthik A, Prabu A, et al. Origanum vulgare mediated biosynthesis of silver nanoparticles for its antibacterial and anticancer activity. Colloids Surf B Biointerfaces. 2013;108:80–84.
- Singh H, Du J, Yi TH. Green and rapid synthesis of silver nanoparticles using Borago officinalis leaf extract: anticancer and antibacterial activities. Artif Cells Nanomed Biotechnol. 2017;45(7):1310–1316.
- Devi JS, Bhimba BV. Anticancer activity of silver nanoparticles synthesized by the seaweed Ulva lactuca in vitro. Sci Rep. 2012;1(4):242–250.
- Seydi N, Mahdavi B, Paydarfard S, et al. Preparation, characterization, and assessment of cytotoxicity, antioxidant, antibacterial, antifungal, and cutaneous wound healing properties of titanium nanoparticles using aqueous extract of Ziziphora clinopodioides Lam leaves characterization and biological effects of TiNPs@Ziziphora. Appl Organometal Chem. 2019;33:e5009.
- Jalalvand AR, Zhaleh M, Goorani S, et al. Chemical characterization and antioxidant, cytotoxic, antibacterial, and antifungal properties of ethanolic extract of Allium Saralicum R.M. Fritsch leaves rich in linolenic acid, methyl ester. J Photochem Photobiol B. 2019;192:103–112.
- Zangeneh MM, Zangeneh A. Biosynthesis of iron nanoparticles using Allium eriophyllum Boiss extract: chemical characterization, antioxidant, cytotoxicity, antibacterial, antifungal, and cutaneous wound healing effects. Appl Organometal Chem.2020;34:e5304.
- (a) Zangeneh MM, Pooyanmehr M, Zangeneh A. Therapeutic effects of Glycyrrhiza glabra aqueous extract ointment on cutaneous wound healing in Sprague Dawley male rats. Comp Clin Pathol. 2019;28:1507–1514.
- Salehi S, Shandiz SAS, Ghanbar F, et al. Phytosynthesis of silver nanoparticles using Artemisia marschalliana Sprengel aerial parts extract and assessment of their antioxidant, anticancer, and antibacterial properties. Int J Nanomedicine. 2016;11:1835–1846. ():
- Park Y, Hong YN, Weyers A, et al. Polysaccharides and phytochemicals: a natural reservoir for the green synthesis of gold and silver nanoparticles. IET Nanobiotechnol. 2011;5(3):69–78.
- Rathor N, Mehta AK, Sharma AK, et al. Acute effect of Aloe vera gel extract on experimental models of pain. Inflammation. 2012;35(6):1900–1903.
- Li G, He D, Qian Y, et al. Fungus-mediated green synthesis of silver nanoparticles using Aspergillus terreus. Int J Mol Sci. 2012;13(1):466–476.
- Subramani K, Shanmugam BK, Rangaraj S, et al. Screening the UV-blocking and antimicrobial properties of herbal nanoparticles prepared from Aloe vera leaves for textile applications. IET Nanobiotechnol. 2018;12(4):459–465.
- Viswanathan K, Bharathi BD, Karuppannan C, et al. Studies on antimicrobial and wound healing applications of gauze coated with CHX-Ag bybrid NPs. IET Nanobiotechnol. 2020;14(1):14–18.
- Medda S, Hajra A, Dey U, et al. Biosynthesis of silver nanoparticles from Aloe vera leaf extract and antifungal activity against Rhizopus sp. and Aspergillus sp. Appl Nanosci. 2015;5(7):875–880.
- Mahdavi B, Saneei S, Qorbani M, et al. Ziziphora clinopodioides Lam leaves aqueous extract mediated synthesis of zinc nanoparticles and their antibacterial, antifungal, cytotoxicity, antioxidant, and cutaneous wound healing properties under in vitro and in vivo conditions. Appl Organometal Chem. 2019;33(11):e5164.
- Hamelian M, Zangeneh MM, Amisama A, et al. Green synthesis of silver nanoparticles using Thymus kotschyanus extract and evaluation of their antioxidant, antibacterial and cytotoxic effects. Appl Organometal Chem. 2018;32(9):e4458.
- Kumar VA, Uchida T, Mizuki T, et al. Synthesis of nanoparticles composed of silver and silver chloride for a plasmonic photocatalyst using an extract from a weed Solidago altissima (goldenrod). Adv Nat Sci Nanosci Nanotechnol. 2016;7(1):015002.
- Martinez-fernandez M, Rubio C, Segovia C, et al. EZH2 in bladder cancer, a promising therapeutic target. Int J Mol Sci. 2015;16(11):27107–27132.
- Lu HC, Ma J, Zhuang Z, et al. Retinoic acid-incorporated glycol chitosan nanoparticles inhibit the expression of Ezh2 in U118 and U138 human glioma cells. Mol Med Rep. 2015;12(5):6642–6648.
- Qin YG, Zhu LY, Wang CY, et al. Glycol chitosan incorporated retinoic acid chlorochalcone (RACC) nanoparticles in the treatment of Osteosarcoma. Lipids Health Dis. 2015;14(1):70.
- Simon JA, Lange CA. Roles of the EZH2 histone methyltransferase in cancer epigenetics. Mutat Res. 2008;647(1-2):21–29.
- Gao F, Ma N, Zhou H, et al. Zinc oxide nanoparticles-induced epigenetic change and G2/M arrest are associated with apoptosis in human epidermal keratinocytes. Int J Nanomedicine. 2016;11:3859–3874.
- Fujii S, Ito K, Ito Y, et al. Enhancer of zeste homologue 2 (EZH2) down-regulates RUNX3 by increasing histone H3 methylation. J Biol Chem. 2008;283(25):17324–17332.
- Ito Y. Oncogenic potential of the RUNX gene family: ‘overview’. Oncogene. 2004;23(24):4198–4208.
- Wang C, Liu Z, Woo CW, et al. EZH2 mediates epigenetic silencing of neuroblastoma suppressor genes CASZ1, CLU, RUNX3, and NGFR. Cancer Res. 2012;72(1):315–324.
- Yoo KH, Hennighausen L. EZH2 methyltransferase and H3K27 methylation in breast cancer. Int J Biol Sci. 2012;8(1):59–65.
- Hanley C, Layne J, Punnoose A, et al. Preferential killing of cancer cells and activated human T cells using ZnO nanoparticles. Nanotechnology. 2008;19(29):295103.