Abstract
Nanotechnology involves working at the atomic, molecular, and macromolecular levels including imaging. Recently, four areas have emerged in cardiovascular imaging: 1. Targeted therapeutics to deliver cardioprotective drugs at the target sites they are needed; 2. Myocardial tissue engineering to replace the defective valves, damaged heart muscle, clogged blood vessels and myocardium; 3. Molecular imaging using “smart” imaging agents in targeted therapeutics and imaging; 4. Biosensors and myocardial diagnostics. Several approaches to nanoparticles i.e. dendrimers, liposomes, polymer delivery molecules, cantilevers, nanoscaffolds, nanofibers are potential candidates in cardiac visualization. The extracellular matrix plays a significant role by chemokines, cytokines and growth factors. The limitations of these emerging techniques and a new possibility of MRI visualization of mouse cardiac atheroma by superparamagnetic iron-oxide gadolinium-apoferritin (SPIOA) and myoglobin (SPIOM), for targeted functional and molecular imaging of atherosclerosis are highlighted in this paper. These emerging techniques provide an opportunity for tracking functional and structural changes in myocardium and heart tissue.
1. Introduction
Nanoparticles are composed of clusters of molecules or atoms. Their size is in the range of 10–100 nm. These particles penetrate across the cells as payload delivery systems, biomarkers, and targeted path tracking biosensors. The success of these nanoparticles depends on their superparamagnetic properties and measurements of these labeled molecules either as their metal content, electrical resistance, induced magnetic field or presence of atomic force microscope cantilevers are the diagnostic assay. In cardiovascular imaging, these can have a significant role for imaging and drug delivery to identify thrombi, clots, apoptosis-linked gene expressed and all these localized changes can be visualized by tracking with nanoparticles. These visible localized changes can be utilized in several cardiovascular applications. The major applications are delivering cardioprotective drugs to target sites; regenerating new tissues to replace defective valves, damaged heart muscle, clogged blood vessels, and so forth; molecular imaging by using “smart” receptor, antibody-ligands imaging agents that identify disease more specifically; and diagnostic biosensor devices for the lab or implantable sensors to detect disorders inside the heart and vessels.
1.1. Physics of nanoparticle action as contrast agent
The iron oxide molecule has iron with an unpaired electron and non vanishing magnetic moment with an associated nonzero dipole magnetic field, so called “paramagnetic”. These moments align in external magnetic fields to generate bulk atomic magnetization. Iron oxide mixed with background substances, behave like a set of very large magnetic moments, producing “superparamagnetism”. With an external field, the aligned iron oxide domains produce strong self-magnetic field and get saturated. As a result, these particles cause signal loss due to dephasing in regions where they are deposited up to the extent of volume of million times in the field as shown in . This dephasing effect causes phase shift resulting with magnitude change as shown below.
Figure 1. The figure represents measurable effective voxel of volume 1000R 3 containing superparamagnetic sphere of radius R in the center. Due to dephasing, it creates signal loss as MRI visible contrast in the ten fold boxed region (10R) or 1000R 3MRI visible volume of interest.
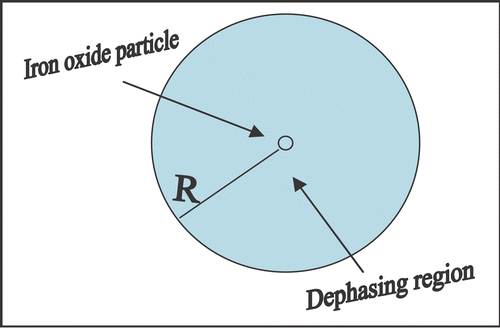
The dipole moment μ of a sphere with radius R can be shown as:
The dipole moment μ will cause change in induced magnetic field B due to particle rotation N as:
From the standpoint of capitalizing the nanoparticles in cardiovascular application, different applications of their use in industry and clinics are reviewed.
1.2. Targeted therapeutics and molecular imaging
The iron oxide nanoparticles with a payload of imaging contrast agent or a drug or a combination of both, can be used for drug delivery and monitoring by images Citation1–12. The iron-oxide nanoparticles bound with specific antibody also serve as a targeting system to locate the leached out antigen from the site of myocardial infarction. Typically, they act as a molecular zip code, for example, a myoglobin antibody that binds to specific cardiomyocyte cells in the body. The targeting system allows for the precise delivery of the drug payload in the body. Other major advancements are made in the development of drug delivery systems. The drug is encapsulated in nanospheres and nanocapsules inside a shell, such as polymeric micelles, with a hydrophobic core and hydrophilic tails.
Other potent encapsulated carriers are liposomes made of hydrophobic lipid micelles. They can contain a drug or imaging agent inside and antibody on the outside with a polyethylene glycol coat to avoid the immune system. These liposomes function for targeting. Recently, dendrimers were particles made of highly branched polymer molecules and they perform delivery. Typically they are known as “quantum dots.” These quantum dots have fluorescent properties useful for fluorescent imaging for cell tracking to assess the efficiency of heart transplantation. Recently, doxorubicin bound NK911 nanoparticles (core-shell nanoparticles of PEG-based block copolymer encapsulating doxorubicin) were reported to inhibit restenosis Citation1. The nanoparticles easily permeate through the blood vessels and vascular injury sustains the hyperpermeability. As a result, nanoparticles help in delivering the drugs to prevent the neointima formation and maintaining luminal area. Another benefit of nanoparticles is to decrease the toxicity of doxorubicin. An example of targeted therapeutics is the killing action against bacterial bioforms in the lung around the myocardium. These can be targeted with inhalation of antimicrobial drugs coated with nanoparticles. Stronger or poorly soluble drugs can be encapsulated to deliver at targets with a controlled release. We used taxotere for cancer treatment and presumed it would work for the prevention of stent restenosis. It was poorly soluble in solvent but its encapsulation in nanoparticles enhanced the solubility up to 40 mg/ml of the drug and allowed for sustained release over several days to monitor its chemosensitivity effect Citation2.
Other advancement is microlevel imaging of cells. The in vivo cell tracking by nanoparticles is restricted by poor sensitivity of imaging methods. However, the superparamagnetic iron oxide highly derivatized and cross-linked iron oxide (CLIO-HD) nanoparticles can enter into lymphocytes through Tat peptide. These labeled T-lymphocytes can be detected with MRI up to the resolution level of 10 microns. The treatment of myocardial infarction can be evaluated and optimized by the tracking of labeled bone marrow cells. Recently the authors proposed the possibility of nanoparticles to bind with inflammatory molecules that recognize alveolar inflammation and detect the bacteria or viruses. These nanoparticles can act as targeted imaging probes that are activated at disease sites. In cardiovascular imaging, these imaging probes can be useful.
These imaging probes get activated by enzymes responsible for remodeling of the heart (heart failure) or arteries (aneurysms). The approval by the FDA for use of iron oxide superparamagnetic particles in molecular imaging is of specific note. Combidex ® as Ferumoxtran-10 to cell labeling is approved for clinical use and commercialized poly-ethylene Glycol coated ferrous oxide particles for animal models Citation12. We used myoglobin bound iron-oxide to visualize mice heart muscles as shown in . Furthermore, iron oxide particles are engulfed by macrophages in atherosclerosis and these macrophages offer an opportunity of imaging them by MRI.
1.3 Role in myocardial tissue engineering
Extracellular signaling includes extracellular matrix, soluble signals, growth factors, cytokines, chemokines, and cell-to-cell contact affecting signal transduction pathways that regulate transcription and gene expression, tissue formation, tissue homeostasis, or tissue regeneration. Injectable self-assembling short peptides into nanofibers can be injected into myocardium to form cell-friendly micro-environments primarily endothelial cells and sarcomeric actins with slower increase in myocytes. This shows the potential for tissue damage recovery in myocardial infarction Citation13. Engineering growth factors and other signals into the matrix show the potential for promoting the differentiation of the myocytes. The matrix also contains smooth muscle cells forming vessel-like structures, so there may be the potential for vascularization of the tissue. Other potent applications of nanoscaffolds impregnated with growth factors and other signaling molecules may guide growth and differentiation of newly visible cardiac tissues by imaging.
1.4. Diagnostics by cantilever sensors
In myocardial infarction (MI), each nanocantilever can be functionalized individually with a different antibody to detect a specific protein. So, binding of an antigen to the nanocantilever causes bending of the cantilever, this in turn deflects a laser beam and thereby provides quantitative measurements. The cantilevers can functionalize plasma antibodies to troponin and myoglobin (indicative of MI). The cantilever array can show very rapid quantitative detection of changes in the DNA sequences, multiplex protein concentration or virus infection in plasma by cantilever array coated with antibodies. The possibility of cantilever sensors in imaging is an unpredictable application today.
1.5. Transferrin, troponins and myoglobins
Transferrin molecules can be attached to each iron oxide particle with chemical linkers, increasing the particle's affinity for the transferrin receptor. The addition of amine groups during dextran cross-linking makes antibodies, snippets of DNA, and other compounds. This advance has opened up entirely new avenues of imaging research. It can functionalize plasma antibodies to Troponin I or T and myoglobin (indicative of MI). The authors achieved resolution up to 100 microns using myoglobin conjugates as shown in . In future, it may be possible to visualize Troponin T leaching out by imaging modalities. Of specific interest, the authors highlight the use of superparamagnetic iron-oxide gadolinium-apoferritin (SPIOA) in cardiovascular imaging. The T1 relaxivities of Apoferritin (1030 ± 20 ms) were drastically reduced upon mixing with gadolinium GdHPDO3A (75.5 ± 13 ms) showing a new possibility of its use as contrast agent (unpublished data) as shown in . Using this approach, the authors report the contrast achieved at high field strength 21 Tesla MRI imager with resolution (23 µm × 23 µm × 30 µm) image of lipid rich vascular wall 3D visualization of necrotic core shown in .
Figure 2. A mouse was injected with gadolinium bund MION-myoglobin particles (courtesy of Dr. Yousef Haik) through a vein in its tail and imaged by MRI at 500 MHz. The vascular regions showed clear wall and lumen areas as shown by arrows. Multislice-multiecho (Bruker Biospin at TE = 15 ms, TR = 1500 ms) gave proton density T 2* images with spatial resolution of 100 microns.
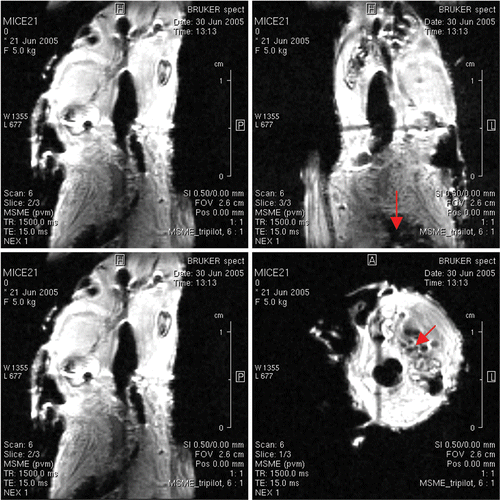
Figure 3. Quantitative T1 maps with pseudocolour obtained from solutions imaged in glass MR tubes. The tubes demonstrate a marked T1 shortening effect by loading GdHPDO3A (Prohance) into apoferritin. The sample on the lower right contains 100 mM Prohance only. After loading, the apoferritin will be extensively dialysed to remove free Prohance from solution, ensuring that the observed increase in relaxivity will be due to GDIII-apoferritin complexes.
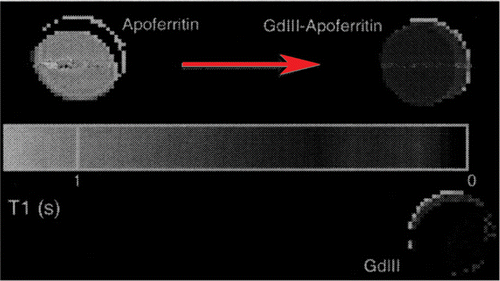
Figure 4. Axial section of the brachiocephalic artery image in control shown in panel (a) and after ‘artery injury’ induced mice ex vivo showing lipid rich necrotic core enhancement by iron oxide particles (arrow in c). Plaque components can be quantified using semi-automated segmentation techniques based on signal intensity histograms shown in panel (b). Segmentation allows quantification of individual vessel components and, in addition, (c) the segmented images can discriminate vessel damage shown with arrow in panel c. Combining slices, it can be reconstructed to show anatomical relationships of different components in 3D such as (demarcated necrotic cores in d). Wall details are visible in three different levels (e).
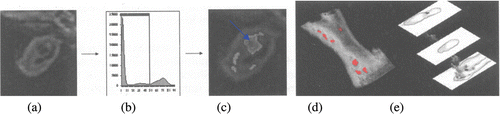
1.6. Lipid coated perfluorocarbons
Perfluorocarbon emulsion coated with a layer of lipid can home antibodies, peptides or short protein fragments and cell surface receptors, more than 200 to 300 of each nanoparticle. Up to 90,000 molecules of gadolinium-DTPA can detect the targeted cell. The perfluorocarbon nanoparticles are useful in cardiovascular imaging. The particles may be able to identify atherosclerotic condition such as fibrin in clots. A protein αVβ3-integrin can detect the immature blood vessels that characterize angiogenesis. A perfluorocarbon nanoparticle can be labeled with a peptidomimetic targeted to αVβ3-integrin. Combined fibrin with αVβ3-integrin targeting can indicate inflammation and rupture Citation14.
1.7. Smart contrast agents
“Smart” agents remain undetected, for example caged gadolinium as a delivery molecule shielded with water protons. These move freely and reach the target site where an enzyme or cellular ion releases the gadolinium. The interaction of water and gadolinium results in changes in T1 relaxation hence useful for MRI. For example, the chelated gadolinium caged by a galactopyranose molecule is opened by β-galactosidase enzyme. Similarly, matrix metalloproteinases, kinases, β-galactosidase and intracellular calcium ions also release gadolinium indicating changes in cell signaling, regulation, and metabolism otherwise imaged by MRI, SPECT or CT. Once the molecule changes shape, water can come in contact with gadolinium, with a detectable reduction in T1 relaxation on MRI.
2. Newer approaches of molecular imaging applications
2.1. Endothelin-1 peptides
Endothelin has received considerable attention as a novel therapeutic target, especially among cardiovascular researchers, because of the potent vasoconstricting and mitogenic effects of the endothelin system.
Endothelin-1 (ET-1) is a 21 amino acid peptide isolated from cultured porcine aortic endothelial cells and is a potent vasoconstrictor. ET-1 acts on two types of G-protein coupled endothelin receptors (ETA and ETB) and causes a variety of functions, including vasoconstriction and mitogenic. ET-1 is produced from the inactive precursor called big ET-1 by the action of ECE. To date, two endothelin-converting enzyme (ECE) family members have been identified: ECE-1 and ECE-2.
Recently, the importance of the endothelin system in development has defined through gene-knockout studies in mice. ETA- and ECE-1-knockout mice die at birth from mechanical obstruction of the airway due to severe malformation of craniofacial structures. These mice commonly display arterial defects and ventricular septum defects Citation15.
Endothelin antagonists such as ETA receptor or ETB receptor selective antagonists showed promising results in animal models of hypertension, congestive heart failure, hypertension. Some have progressed human trials.
Other aspects were focused to prove the efficacy of an Endothelin converting enzyme (ECE-1) inhibitor to prevent hypertensive heart failure, and found the inhibitor had cardioprotective effects beyond blood pressure. With these developments of ET antagonists/inhibitors, several questions still need to be answered before implementing it for nanotechnology. To inhibit the action of ET-1, ECE inhibitor could be an alternative approach. However, still unclear if ETA selective or ETA- ETB-non-selective antagonist is better? For an endothelin converting enzyme inhibitor, which one is better-an ECE-1 selective or ECE-1/ACE dual inhibitor? What are the other optimal target diseases? These issues may certainly supplement the molecular imaging possibility in future.
The authors believe that factors such as VEGF and FGF may be used in gene therapy, and these have been suggested to have a beneficial effect in cardiovascular diseases. It could be useful for the prevention of restenosis, because of HGF-induced re-endothelization, and also be applied in ischemic diseases because of its powerful angiogenic action. Moreover, HGF has anti-apoptotic effects and anti-fibrotic effects on cardiomyocytes. Thus, cardiomyopathy could also be targeted with HGF. The evaluation of VEGF and FGF in imaging application is in progress today.
2.2. Fibronectins and adhesion molecules
Selective targeting of atheromas in mice can be done using the human antibody to the ED-B domain of fibronectin. It may set the stage for antibody-based molecular imaging of . A mouse was injected with gadolinium bound MION-myoglobin particles (courtesy of Dr Yousef Haik) through tail in vien and imaged by MRI at 500 MHz. The vascular regions showed clear wall and lumen areas as shown by arrows. Multislice-multiecho (Bruker Biospin at TE = 15, TR = 1500 ms) gave proton density images with spatial resolution of 100 microns for atherosclerotic plaques in the intact organism. Adhesion molecules ICAM and VCAM immunoactive molecules may also show their specific role in the prediction of endothelial injury.
2.3. Metabolic imaging
It provides new possibility by fluorescent labeled molecular tracking and cell imaging. Of specific mention, by positron emission tomography and C-13 Magnetic Resonance Spectroscopy it can predict the reduced fatty acid oxidation enzyme activity leading to contractile dysfunction and heart failure Citation16.
2.4. Possible imaging applications
Several current approaches can be useful to researchers or utilizable in imaging of cardiovascular tissue. They were introduced earlier and can be grouped as following:
-
The specific and sensitive site-targeted contrast agents act as epitopes. Membrane phosphatidyl serine epitopes are exposed during apoptosis Citation4.
-
Transfection events by imaging proteins that are expressed after reporter gene transcription i.e. thymidine kinase genes as reporter construct in association with a therapeutic gene by phosphorylating certain exogenously supplied radiolabeled probes (or substrates) that are then trapped inside of cells where then can be imaged Citation5.
-
Biocompatible nanotechnologies provide targeted contrast agents with long circulating half-life (hours), selective binding to epitopes of interest, low background signal and prominent contrast-to-noise enhancement, acceptable toxicity profile, ease of production and clinical use, applicability with standard commercially available imaging modalities, and promise for adjunctive therapeutic delivery in drug and/or gene delivery nanosystems.
-
The contrast mechanism of nanoparticles (liposomes or emulsions) Citation6–8, dendrimers Citation9, Citation10, viral constructs Citation11, buckeyballs Citation17, or various polymers Citation18, Citation19 can hold paramagnetic or superparamagnetic metals, optically active compounds (e.g., fluorescent molecules), or radionuclides. These can be detected with standard imaging equipment i.e. liquid perfluorocarbon nanoparticlesCitation6, Citation20, Citation21.
-
The contrast agent can be monoclonal antibodies or their fragments, peptides, small molecule peptidomimetics, or haptamers. Dissociation constants in the nanomolar range, multivalent binding enhance avidity and reduce “off-rates” to permit imaging at convenient times after delivery of the agent. Polyvalent binding is possible with the use of more than one ligand type per carrier, or with mixtures of ligand-carrier constructs directed at different targets. MRI and ultrasound use paramagnetic polymerized liposomes bearing antibody ligands to neovascular integrins αVβ3 Citation7. Similarly, liquid perfluorocarbon nanoparticle emulsions targeted to αVβ3 integrins permit fibrin visualization in thrombi Citation21 and carotid plaques ex vivo Citation22. The maximum T1 per molecule binding site for a complex of paramagnetic perflurocarbon agents can give sensitivity in the picomolar range of concentrations Citation23. Even single cells may be imaged with such agents Citation24. However, epitopes such as fibrin in thrombi can be used in targeting. Recently, detection of vascular adhesion molecule-1 expression using a novel multimodal nanoparticle was reported Citation25.
-
The ultrasmall iron oxide imaging agents have the potential for delineation of early atherosclerosis in experimental models by uptake of ultra-small particles of iron oxide in plaque macrophages Citation26.
-
The “smart” agents carry gadolinium at pathologic states, or by the protein products (enzymes) of reporter genes after therapeutic transfection Citation27. Cleavage of active sites on these agents exposes sequestered gadolinium atoms to free water and facilitates rapid water exchange to produce an effect on local proton relaxation, in turn enhancing image contrast.
-
Targeted perfluorocarbon nanoparticles are useful for ultrasound applications and fibrin thrombi in vivo by two orders of magnitude or moCitation6, Citation28, Citation29. Additionally, targeting to vascular epitopes such as tissue factor, whose expression is induced in smooth muscle cells in vivo after angioplasty, is possible because these particles can penetrate through microfissures into the vascular medi Citation20, Citation30. Reflective liposomes have also been used to specifically target endothelial αVβ3 integrins Citation8. Other microscale systems (stabilized perfluorocarbon gas microbubbles) have been used for molecular ultrasound imaging of thrombi Citation31 but generally these are restricted to the vasculature in view of their size (5 µm) and their susceptibility to destruction with clinical ultrasound imaging intensities.
-
Nanoscale imaging mass spectrometry provides exciting opportunities for mapping proteomes in the myocardial tissue. However, its present use is very limited but decisive and unpredictable.
-
The lower abundance of vascular surface markers of VCAM-1 and αVβ3 integrins by using superparamagnetic iron-oxide particles bound with antibody complexes, provide exciting opportunity of imaging of vascular injury and sites of inflammation.
-
High density intravascular epitopes such as fibrin, CD41 in platelets are of tremendous importance in endothelial injury and thrombosis imaging by superparamagnetic particles/specific antibody complexes in animal models of diseases.
3. New imaging applications in cardiovascular therapy
The emerging use is the ability to incorporate drugs or genes into detectable site-targeted nanosystems as drug delivery and dosing. Payloads of therapeutic agents, such as genes or radionuclides, can be complexed to the carriers themselves.
Drugs in the lipid coatings get trapped within the carriers themselves. In the case of ultrasound, methods for gene delivery with synthetic vectors such as microbubbles rely on mechanical stimulation of microbubbles that operate like miniature gene guns Citation32. Stabilized gaseous microbubble contrast agents (5 nm in diameter), otherwise only visible by imaging modalities, have demonstrated potential for use as transfection agents by incorporating DNA directly into the bubble shell or interio Citation33, Citation34.
Focused ultrasound destroys the bubbles and releases genes at selected sites. The opportunity to confirm drug delivery and dose by imaging represents a novel feature for nanosystems that provide controlled drug release. Fortunately, the imaging signals produced by the carriers themselves, along with knowledge of the amount of the specific drug contained per particle, will allow estimation of tissue drug levels. In the case of paramagnetic nanoparticles, a range of perfluorocarbon components can be detected and quantified by NMR spectral analysis to permit quantitative differentiation among a variety of simultaneously targeted molecules Citation23. Recently it has been discovered that targeted “quantum dot” nanosystems might be useful in imaging Citation35. Finally the major focus is to utilize: 1. The molecular imaging and the development of biocompatible nanotechnologies. 2. Complete characterization of the human genome and the subsequent emergence of proteomics, 3. Useful molecular targets.
3.1. New technical developments in nanoparticle based imaging
The notable possible uses of nanoparticles in cardiovascular imaging are for cell tracking by dextron coated iron oxide particles, single cell imaging, contrast enhancement, blood volumetry, relaxivity measurements, off-resonance susceptibility, apoptosis imaging with polyethylene glycol-iron oxide-annexin V, epidermal Growth factor (EGF) receptor targeting and positive contrast by diagonal SPRITE as reported recently elsewhere Citation36. The main focus of nanotechnology still remains unresolved in cell tracking by MRI. In this direction, other unconfirmed applications of nanoparticles in enhanced visualization are notable by hybrid imaging systems now available. Hybrid Positron Emission Tomography/Computed Tomography is in the way to predict angiogenesis, myocardial perfusion, coronary angiography and plaque composition.
The use of magnetic labeling of stem cells using iron oxide compounds followed by transplantation and transfusion showed remarkable tracking of cell distribution Citation37. There is the possibility that stem cell therapy of ischemic heart disease may depend on the accurate assessment of improved perfusion by PET/SPECT and optical imaging. Still the application of gene therapy and stem cell approaches are in their infancy Citation38. Imaging of fibrous cap rupture by iron oxide nanoparticles like SPIO or MION MRI and CT provides unique ways to assess vulnerable plaques.
4. Conclusion
Nanotechnology has enormous potential for diagnosing and treating heart conditions. The major cardiovascular applications are monocrystalline iron oxide nanoparticles, or MIONs wrapped in dextran, cross-linked iron oxide nanoparticles, or CLIOs, transferrin receptors. CLIOs have been attached to multiple sets of DNA building blocks, or oligonucleotides, messenger RNA-targeted CLIOs. Smart imaging agents, delivery systems, ferritin, integrins in targeted imaging of arteries and heart using hybrid imagers are in the development stage.
Acknowledgements
The present work was supported by Florida State University Cornerstone Grant programme activity at Center of Nanomagnetics and Biotechnology with Dr Ching J. Chen as Director of the center.
References
- Uwatoku , T , Shimokawa , H and Abe , K . 2003 . Application of nanoparticle technology for the prevention of restenosis after balloon injury in rats . Circ. Res. , 92 : 62
- Sharma , R , Kline , RP and Wu , EX . 2005 . Rapid in vivo Taxotere quantitative chemosensitivity response by 4.23 Tesla sodium MRI and histo-immunostaining features in N-Methyl-N-Nitrosourea induced breast tumors in rats . Cancer Cell Int. , 3 : 26
- Matter , CM , Schuler , PK and Alessi , P . 2004 . Molecular imaging of atherosclerotic plaques using a human antibody against the extra-domain B of fibronectin . Circ. Res. , 95 : 1225
- Blankenberg , FG and Strauss , HW . 2001 . Noninvasive strategies to image cardiovascular apoptosis . Cardiol. Clin. , 19 : 165
- Bengel , FM , Anton , M and Avril , N . 2000 . Uptake of radiolabeled 2′-fluoro-2′-deoxy-5-iodo-1-beta-D arabinofuranosyluracil in cardiac cells after adenoviral transfer of the herpesvirus thymidine kinase gene: the cellular basis for cardiac gene imaging . Circulation , 102 : 948
- Lanza , GM , Wallace , KD and Scott , MJ . 1996 . A novel site-targeted ultrasonic contrast agent with broad biomedical application . Circulation , 95 : 3334
- Sipkins , DA , Cheresh , DA and Kazemi , MR . 1998 . Detection of tumor angiogenesis in vivo by alphaVbeta3-targeted magnetic resonance imaging . Nat. Med. , 4 : 623
- Demos , S , Alkan-Onyuksel , H and Kane , B . 1999 . In vivo targeting of acoustically reflective liposomes for intravascular and transvascular ultrasonic enhancement . J. Am. Coll. Cardiol. , 33 : 867
- Wiener , C , Brechbiel , MW and Brothers , H . 1994 . Dendrimer-based metal chelates: a new class of magnetic resonance imaging contrast agents . Magn. Reson. Med. , 31 : 1
- Bryant , LHJ , Brechbiel , MW and Wu , C . 1999 . Synthesis and relaxometry of high-generation (G = 5, 7, 9, and 10) PAMAM dendrimer-DOTA-gadolinium chelates . J. Magn. Reson. Med. , 9 : 348
- Lu , PY , Xie , F and Woodle , MC . 2005 . In vivo application of RNA interference: from functional genomics to therapeutics . Adv. Genet. , 54 : 117
- Wu , EX , Tang , H and Jensen , JH . 2004 . Applications of ultrasmall superparamagnetic iron oxide contrast agents in the MR study of animal models . NMR in Biomed. , 17 : 478
- Hsieh , PC , Davis , ME and Gannon , J . 2006 . Controlled delivery of PDGF-BB for myocardial protection using injectable self-assembling peptide nanofibers . J. Clin. Invest. , 116 : 2378
- Cyrus , T , Abendschein , DR and Caruthers , SD . 2006 . MR three-dimensional molecular imaging of intramural biomarkers with targeted nanoparticles . J. Cardiovasc. Magn. Reson. , 8 : 535
- Loghin , C , Sdringola , S and Gould , KL . 2006 . Does Coronary Vasodilation After Adenosine Override Endothelin-1 Induced Coronary Vasoconstriction? . Am. J. Physiol. Heart. Circ. Physiol. , 9 : 1
- Flogel , U , Laussmann , T and Godecke , A . 2005 . Lack of myoglobin causes a switch in cardiac substrate selection . Circ. Res. , 96 : 68
- Noon , WH , Kong , Y and Ma , J . 2002 . Molecular dynamics analysis of a buckyball-antibody complex . Proc. Nat. Acad. Sci. USA , 99 : 6466
- Roessler , BJ , Bielinska , AU and Janczak , K . 2001 . Substituted beta-cyclodextrins interact with PAMAM dendrimer-DNA complexes and modify transfection efficiency . Biochem. Bioph. Res. Comm. , 283 : 124
- Ma , Q , Remsen , EE and Kowalewski , T . 2001 . Two-dimensional, shell-cross-linked nanoparticle arrays . J. Am. Chem. Soc. , 123 : 4627
- Lanza , GM , Abendschein , DR and Hall , CH . 2000 . In vivo molecular imaging of stretch-induced tissue factor in carotid arteries with ligand-targeted nanoparticles . J. Am. Soc. Echocardiog. , 13 : 608
- Yu , X , Song , SK and Chen , J . 2000 . High-resolution MRI characterization of human thrombus using a novel fibrin-targeted paramagnetic nanoparticle contrast agent . Magnet. Reson. Med. , 44 : 867
- Anderson , SA , Rader , RK and Westlin , WF . 2000 . Magnetic resonance contrast enhancement of neovasculature with alpha(v)beta(3)-targeted nanoparticles . Magnet. Reson. Med. , 44 : 433
- Flacke , S , Fischer , S and Scott , MJ . 2001 . Novel MRI contrast agent for molecular imaging of fibrin: implications for detecting vulnerable plaques . Circulation , 104 : 1280
- Lanza , GM , Yu , X and Winter , PM . 2002 . Targeted antiproliferative drug delivery to vascular smooth muscle cells with a magnetic resonance imaging nanoparticle contrast agent: implications for rational therapy of restenosis . Circulation , 106 : 2842
- Kelly , KA , Allport , JR and Tsourkas , A . 2005 . Detection of vascular adhesion molecule-1 expression using a novel multimodal nanoparticle . Circ. Res. , 96 : 327
- Ruehm , SG , Corot , C and Vogt , P . 2001 . Magnetic resonance imaging of atherosclerotic plaque with ultrasmall superparamagnetic particles of iron oxide in hyperlipidemic rabbits . Circulation , 103 : 415
- Louie , AY , Huber , MM and Ahrens , ET . 2000 . In vivo visualization of gene expression using magnetic resonance imaging . Nat. Biotechnol. , 18 : 321
- Bulte , JW , Douglas , T and Witwer , B . 2001 . Magnetodendrimers allow endosomal magnetic labeling and in vivo tracking of stem cells . Nat. Biotechnol. , 19 : 1141
- Lanza , GM , Trousil , RL and Wallace , KD . 1998 . In vitro characterization of a novel, tissue-targeted ultrasonic contrast system with acoustic microscopy . J. Acoust. Soc. Am. , 104 : 3665
- Lanza , GM , Abendschein , DR and Hall , CH . 2000 . Molecular imaging of stretch-induced tissue factor expression in carotid arteries with intravascular ultrasound . Invest. Radiol. , 35 : 227
- Takeuchi , M , Ogunyankin , K and Pandian , N . 1999 . Enhanced visualization of intravascular and left atrial appendage thrombus with the use of a thrombus-targeting ultrasonographic contrast agent (MRX-408A1): in vivo experimental echocardiographic studies . J. Am. Soc. Echocardiog. , 12 : 1015
- Song , J , Chappell , JC and Qi , M . 2002 . Influence of injection site, microvascular pressure and ultrasound variables on microbubble-mediated delivery of microspheres to muscle . J. Am. Coll. Cardiol. , 39 : 726
- Unger , EC , McCreery , TP and Sweitzer , RH . 1997 . Ultrasound enhances gene expression of liposomal transfection . Invest. Rad. , 32 : 723
- Shohet , R , Chen , S and Zhou , YT . 2000 . Echocardiographic destruction of albumin microbubbles directs gene delivery to the myocardium . Circulation , 101 : 2554
- Åkerman , ME , Chan , WCW and Laakkonen , P . 2002 . Nanocrystal targeting in vivo . Proc. Nat. Acad. Sci. USA , 99 : 12617
- Protti , A , So , P-W , Herlihy , A and Bell , J . 2006 . “ SPIO positive contrast in-vivo by the use of Diagonal-SPRITE ” . In Proc. Int. Soc. Magn. Reson. Med. USA at Seattle 361 WA
- Bulte , JW , Duncan , ID and Frank , JA . 2002 . In vivo magnetic resonance tracking of magnetically labeled cells after transplantation . J. Cerebrovas. Blood Flow Met. , 22 : 899
- Neuberger , T , Schopf , B and Hofmann , H . 2005 . Superparamagnetic nanoparticles for biomedical applications: Possibilities and limitations of a new drug delivery system . J. Magn. Magn. Mater. , 293 : 483