Abstract
Superparamagnetic iron oxide (Fe2O3) has emerged as a potential MRI contrast agent in whole animal imaging. We achieved initial success in binding superparamagnetic iron oxide with myoglobin antibody (SPIOM) in order to develop it as a possible MRI contrast agent. In this paper, we highlight the binding properties of iron oxide with myoglobin and their pH dependence shown by Raman spectroscopy and MRI imaging. The use of iron oxide--myoglobin superparamagnetic particles as a contrast agent was developed in order to visualize its myoglobin distribution within a mouse heart using MRI imager at 11.7 Tesla. To validate the MRI visualization, the MRI imaging of iron-oxide bound myoglobin SPIOM phantom was carried out using capillaries filled with contrast agent at pH 7 and pH 4. The MRI imaging can serve as a reference to measure the density distribution of myoglobin at different SPIOM concentrations in the mouse's body, especially its heart and blood vessels. We report the technical details of SPIOM and its possible use in MRI as a tool for visualizing mice heart and myocardium.
Keywords:
1. Introduction
Myoglobin is abundant in cardiac muscles in two forms: oxymyoglobin or deoxymyoglobin. It has very high binding affinity with carbon monoxide. As a result, it serves as an indicator of oxygen reserve and also acts as an oxygen reservoir. In cardiac muscle injury, myoglobin (Mb) immediately reflects the early events of ischemia and the possibility of necrosis. For development of a microsphere, myoglobin can be encapsulated in silica glass and can be bound with MnO2. Its smaller size and oxygen carrying capacity provides the opportunity to develop a myoglobin biosensor which acts as the payload for an anti-myoglobin antibody on superparamagnetic iron oxide particles. However, the properties of the myoglobin-antibody and superparamagnetic iron oxide particles are affected by several physiological properties and their covalent binding characteristics. In this report, we highlight evidence of encapsulated myoglobin feasibility and its physical binding characteristics with iron oxide along with its applications in imaging.
2. Principles of SPIOM contrast agent preparation
Above Curie temperature, magnetic particles lose their long-range order of atomic magnetic moments and become paramagnetic. In the presence of magnetic fields, these particles align in the direction of the applied field. In the micron sample size, these particles align in single domain and become ‘superparamagnetic’. Typically, Fe2O3 coated with albumin showed this property Citation1. Superparamagnetic iron oxide with myoglobin antibody (SPIOM) can be prepared by covalent coupling and microspheres using Bang's Lab Technical Note 205 method Citation2. For coating microspheres, there are a number of strategies that are considered, including covalent coupling, adsorption and affinity binding and evaluation of the coating procedure. However, binding protocol design and optimization further also relies on the specific reactive groups and their coupling chemistry such as chemical groups on ligand and microsphere. In general, biomolecules are coupled to polymeric surfaces or silica microspheres through polymeric chemical reactions and silica surface binding. Crosslinking reagents (linker, spacer, activator) have affinity for reactive groups on the bead or biomolecule surface. Crosslinking reagents ‘activate’ groups that exhibit low reactivity in an aqueous hydrophilic environment. The main considerations in the binding procedure are microsphere composition; reagent quality; reagent concentration; buffers; blockers and bead handling. At our Center of Nanomagnetics and Biotechnology, different carboxyl-modified microspheres, amino-modified microspheres, hydroxyl-modified microspheres, hydrazide-modified microspheres and chloromethyl-modified microspheres were prepared Citation3. Specifically: albumin, polystyrene, polyethylene and hydroxyl-propyl cellulose with a core of Fe2O3 (32% iron) were used in the preparation of nanospheres using 30% avidin to functionalize the surface of the surfactant and the monolayer coating of particles. For details, please see the technical no Citation2, Citation3. In this process, dispersion and uniformity of particles were some of the crucial factors. The capacity of the nanosphere as a superparamagnetic particle contrast agent depends on the magnetic moment generated. The emerging possibilities of biomedical use of these superparamagnetic particles in imaging can be categorized as following:
-
Cell trafficing and monitoring acute myocardial infarction (AMI) based on magnetic nanoparticles
-
High sensitivity biocompatible sensors to detect biomolecules
-
Magnetic Resonance Imaging immunoassays
-
Avidin-biotin antibody imaging contrast agents.
However, other emerging applications developed at the Center of Nanomagnetics and Biotechnology are notably red cell separation, magnetic immunoassays, motion detection of cells by Hall effect transducer, avidin-biotin antibody imaging contrast agents, hydroxyl-propyl cellulose magnetic particles, solid-phase Enzyme Linked Immuno-Sorbent Assay and magnetic hyperthermia Citation4.
3. Iron oxide--myoglobin characterization by Raman spectroscopy
Myoglobin and iron oxide binding behavior are highly pH dependent i.e. lactic acidosis in the muscles changes the binding kinetics of myoglobin with iron oxide or oxygen saturation. So, it becomes necessary to characterize these changes. Raman spectroscopy is method of choice for this characterization. The sol--gel encapsulation method preserves the natural free unbound form of myoglobin intact and so, is the most suited technique. It is also a powderless method enabling the preparation of native myoglobin directly from solution. Precursors are mixed at the molecular level i.e. deoxymyoglobin(His F8) and Fe2+ at different pH conditions. In preparation of the myoglobin nanoparticles, the sol--gel powderless method was used Citation5. The behaviour of iron oxide in myoglobin can be predicted by the interaction of Histidine(His F8) with the iron moiety of myoglobin at different pH conditions identified as distinct vibrational Raman spectral frequencies. The oxygen content bound at the sixth co-ordinate valency on the iron atom represents the myoglobin's state in the tissue environment. The same principle applies to the encapsulated myoglobin and iron oxide complex to develop the superparamagnetic imaging contrast agent. However, success also depends on several variables (including preparation conditions such as pH, temperature etc.) to prepare classical silica glasses Citation2. The interaction and behaviour of myoglobin and iron oxide binding or oxygen binding with iron in myoglobin can be predicted by Raman Spectroscopy (personal communication at Raman Spectroscopy facility of Chemistry department, FSU) Citation6. The previous reports on sol--gel encapsulation were confirmed for iron oxide--myoglobin binding behaviour at low pH range of 2.5–3.5 Citation5–7.
Raman spectroscopy showed that sol--gel encapsulation of deoxymyoglobin (deoxyMb) dramatically slows down the low pH induced cleavage of the iron and proximal histidine (Fe--His F8) bond at pH values as low as 2.0.
To explain the biochemical basis of these physical distinct intermediate forms of Fe-His F8 moiety, the ionic interactions can be shown as follows in support of distinct Raman spectroscopy frequencies of distinct forms. The Edge view of myoglobin shows the interaction of His (F8)–FeO–His (E7) and polar behaviour of iron oxide moiety.
The molecular orbital model of iron oxide bonding explains the iron oxide and deoxymyoglobin affinity. When deoxy-myoglobin in a high-spin ferrous state (S = 2) combines with dioxygen in a triplet ground state (S = 1), s-donation primarily arises from the overlap of an antibonding π orbital of dioxygen with the dz 2 orbital of iron. As a result, partial transfer of an electron occurs simultaneously from a t 2 g orbital of iron to another π orbital of dioxygen so as to place oxymyoglobin in a diamagnetic low-spin state (S = 0).
The binding equilibrium behaviour of iron oxide with myoglobin explains the capacity of iron oxide to bind with the myoglobin chain, a crucial factor. In general, the reversible binding of myoglobin (P) to iron oxide (L) can be described by equilibrium expression as follows in equations (Equation1) and (Equation2),
The bound fraction θ of iron oxide with antimyoglobin will be:
In this respect, the FeO2 bonding of Mb seems to be thermodynamically stable if the globin protein is isolated from its aqueous environment. However, things are quite different in vivo. The FeO2 center is always subject to the attack of an entering water molecule or hydroxide anion. These can cause irreversible oxidation of MbO2 to its ferric met-form with generation of the superoxide anion () Citation9. To stabilize the bound dioxygen, therefore, Mb seems to have evolved with a globin moiety that can protect the FeO2 center from easy access of a water molecule, including its conjugate ions OH− and H+. Although the globin moiety has still not attained maximal ability to block entering water molecules from the FeO2 center, it has obtained relative stability as the oxygenated forms, in proton-rich aqueous solution and at physiological temperature.
The FeO2 center of myoglobin is always subjected to the bimolecular, nucleophilic displacement of the superoxide anion from iron oxide center (SN2 attack) by an entering water molecule, accompanied by a very strong acid-catalysis performed by the distal (E7) histidine residue. The FeO2 center is also open to the attack by an entering hydroxide anion in the basic pH range as shown in . However, binding of myoglobin with iron oxide may be altered due to the steric effects of other surrounding amino acids i.e. Phenylalanine (Phe CD1), Valine (Val E 11), or Histidine (His E7) as recently evidenced Citation10.
Myoglobin has a heme moiety (protoporphyrin ring with four nitrogen atoms) bound with a single ferrous (Fe 2+) species with high oxygen binding capacity, at its sixth coordinate bond. The existence of intermediates at different pH ranges, supports the distinct intermediates seen by Raman spectroscopy and other spectroscopic methods Citation10. At low pH acidic conditions, unfolding of deoxyMb occurs in solution. In solution, it precedes through two intermediates, both of which exhibit a loss of the Fe--His bond. Between pH 4.5 and 3.5, an intermediate is formed. In this intermediate, the heme moiety remains a five-coordinate valent moiety, but water replaces the proximal histidine as the fifth ligand. Between pH 3.5 and 2.5, a second spectroscopically distinct intermediate, is formed. In this second intermediate, the heme becomes a four-coordinate valent moiety but appears to remain within the heme pocket. At the low end of this pH regime, further unfolding and associated heme loss becomes the dominant processes as shown in and and . However, the sol-gel imposes stability that influences the acid-induced formation of the deoxyMb unfolding intermediates.
Figure 1. Low-frequency resonance Raman spectra of sol--gel encapsulated deoxyMb. The top spectrum (a) is at pH 7.0. After the jump of pH from 7.0 to 2.6, spectra were taken at (b) 5 min, (c) 15 min, and (d) 100 min. Each spectrum shows the data collected at 30 sec time intervals for spectra (a), (b), and (c) and 15 min for spectrum (d). For better clarity, the intensity of spectrum at Raman shifts in the range of wave numbers 200–1700 cm−1 for spectra (a--d) is vertically displaced (on left) and (a--b) (on right). The intensity of spectrum (d) is normalized to the same collection time (30 sec). Modified from the Reference with permission Citation7.
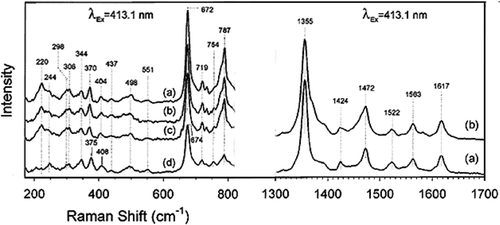
Figure 2. A detailed edge view geometry of the FeO2 bond in the myoglobin molecule in the top row. The bound dioxygen is bent, held end-on and forms a hydrogen bond to the distal (E7) histidine at position 64, with the angles of +Fe–O–O = 115° and angle between O–O–H = 96°. A simplified molecular orbital (MO) representation of the FeO2 molecule is shown at the bottom. Modified from reference with permission Citation7.
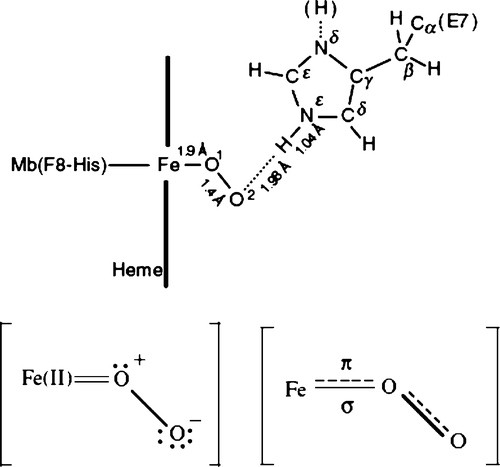
Figure 3. Schematic representation of the basal autoxidation process in water with the rate constant kH2O and the protoncatalyzed process with the rate constant kH H2O in neutral pH range. For sperm whale MbO2, kH2O = 0:18 × 10−4 h−1M−1 and = 0:20 × 104 h−1M−2 in 0.1 M buffer at 25°C. The aqua-met Mb formed by each process can be isomerized into the other. Modified from reference with permission Citation8.
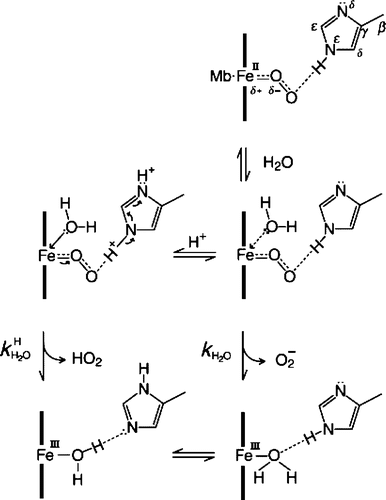
Table 1. Low-frequency resonance Raman spectra intensity (in arbitrary units or a.u.) of sol--gel encapsulated deoxyMb represented as base value (100) and reduced intensity value (in%) from base 100 a.u. at different pHs (column 1) and duration (column 2). The Raman shifts show more sensitivity at excitation frequency λex = 441 nm* (shown here) than λex = 413 nm (not shown). The reduced intensities of spectral peaks at low pH after jump and duration of time are notable due to Fe--His F8 linkage disruption and conformational fluctuations resulting in heme pocket unfolding or opening and more protonated His pocket in myoglobin. The formation and existence of intermediates are shown as Raman shifts or wave number (cm−1) in columns 3–7 represented as spectral peaks in .
Typically, in Raman studies of various myoglobins (Mb), the vibrational stretching frequency of the Fe--His(F8) bond (V Fe--His) was characteristically in the 200–230 cm−1 region in a tetramethyl orthosilicate (TMOS) derived wet sol--gel matrix at pH 7.0 and 2.6 Citation6. The pH plays a significant role in assessment of muscle metabolism i.e. lactic acidosis occurs at low pH. Thus myoglobin protein encapsulated in the gel, retains its structure and spectroscopic properties at pH higher than 3.5 and above. So, myoglobin deoxyMb encapsulated in a wet gel at neutral pH is stabilized in its native form Citation6.
Other recently emerged Surface Enhanced Raman Scattering (SERS) uses an incident wave at frequency ω resulting in a scattered field at a new shifted frequency ω s . This shift ω − ω s is equal to one of the molecule's vibrational frequencies, and the combination of all such vibrational frequencies represents the myoglobin molecule's “fingerprint” Citation11. SERS is a linear, incoherent optical process. Because the plasmon modes cover a very broad spectral range, the fields at frequencies ω and ω s can both experience the enhancement caused by the resonant plasmon modes. As a result, the enhancement factor for SERS is given by the product of the enhancements for the two fields at frequencies ω and ω s Citation11. The enhancement G RS can be represented as following,
The SERS has additional advantage in explaining the unfolding of the globin moiety which increases the ease of attack of the solvent water molecule or hydroxyl ion on the FeO2 center and causes a very rapid formation of the Ferric met-species by the nucleophilic displacement mechanism. In the molecular evolution from simple ferrous complexes to myoglobin and hemoglobin molecules, as illustrated in , the protein matrix can be depicted as a breakwater of the FeO2 bonding against protic, aqueous solvent Citation12.
Figure 4. Role of the globin moiety in stabilizing the FeO2 bond in myoglobin. Notice the globin chain folding in relation to time. Redrawn from the reference with permission Citation8.
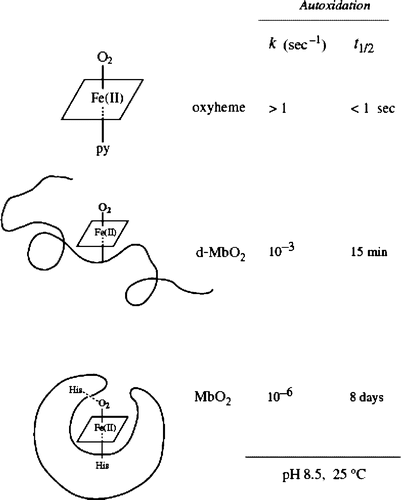
Mb has evolved with a globin moiety that can protect the FeO2 center from easy access of a water molecule and its conjugate ions (OH− and H+). The polypeptide matrix can play a considerable role in stabilizing the oxygenated heme, but the integrity of the native protein architecture is essential to obstruct access of a water molecule to the FeO2 center. In the molecular evolution from simple ferrous complexes to myoglobin and hemoglobin molecules therefore, the protein matrix can be depicted as a breakwater of the FeO2 bonding against protic, aqueous solvents. The free energy change of activated complex is represented as:
4. Synthesis of encapsulated nanoparticles and magnetic immnoassays
At our lab, maghemite particles were coated with albumin to prepare albumin coated maghemite microspheres to attach to red blood cells as shown in Citation13. Avidin was used as a model ligand for immunoassays and it was used for monolayer formation and coating on particles Citation13. The magnetization measurements suggested the suitability of their superparamagnetic characteristics for MRI imaging. These particles were reversibly separated from solution with a small magnet. This property was suitable for magnetic immunoassays. Recently, immunoassay was developed to detect myoglobin and fatty acid binding protein (FABP) using solid phase ELISA Citation13. Human myoglobin and FABP were used as antigens and two different monoclonal anti-myoglobin or anti-FABP antibodies targeting two epitopes on the myoglobin or FABP protein. The antibodies conjugated with myoglobin protein forming microsphere-myoglobin-enzyme complexes. Using external magnets, superparamagnetic properties of the microspheres allowed the separation of the complex from all background media after repeated washing.
Figure 5. (On leftmost): A electron microscopic TEM image of Fe2O3; (on second left) SEM of albumin microspheres; (on third panel) TEM image of polyethylene coated microspheres; (on rightmost panel): A SEM image of polystyrene coated microsphere (shown with arrow) attached to red blood cell. Redrawn from references Citation1, Citation13.
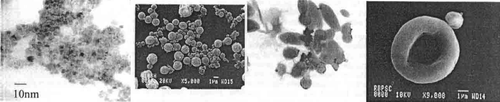
The myoglobin concentration was measured by linked enzyme labelling in the complex Citation13. In another biotin-avidin conjugate method used at our lab, biotin served as bridge between the spheres-antibody moeity and enzyme-antibody moeity. It was used for myoglobin measurement by use of cardiac antihuman myoglobin(mouse epitope IgG1:908 and 4E2 from Research Diagnostics Inc.). The epitopes were placed widely apart on the myoglobin protein Citation14. Coupling of alkaline phosphatase enzyme with streptavidin and avidin-bound superparamagnetic microspheres was achieved by antibody biotinylation using a Sulfo-NHS-LC-biotinylation kit (Pierce Chemicals) as reported elsewh Citation14, Citation15. Using these microspheres and biotinylated antibodies specific to myoglobin, tagged with biomarker molecule, a two-site immunoassay was developed for myocardial infarction detection as shown in .
Figure 6. The figure represents the scheme of two-site immunoassay to develop magnetic immunoassay using anti-myoglobin biotinylated antibodies and tagged biomarker to measure myoglobin. Redrawn from reference Citation15.
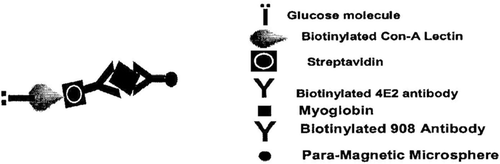
5. Myoglobin encapsulation and uses
Novel sol--gel synthetic techniques were used for myoglobin (Mb) by encapsulation in a stable, optically transparent, porous silica glass matrix under mild conditions such that the biomolecules retained their characteristic reactivities and spectroscopic properties Citation5, Citation14. The resulting silica glass allowed the transport of small molecules into and out of the glass at reasonable rates but nevertheless retained the protein molecules within their pores. Chemical reactions of the immobilized proteins could be monitored by means of changes in their visible absorption spectra. Silica glasses containing the immobilized proteins were observed to have similar reactivities and spectroscopic properties to those of the proteins in solutio Citation14, Citation15. For example, encapsulated superoxide dismutase (CuZnSOD) was demetallated and remetallated, and encapsulated ferricytochrome C was reduced and then reoxidized, and again encapsulated met Mb was reduced to deoxy Mb and then reacted either with dioxygen to make oxy Mb or with carbon monoxide to make carbonyl Mb Citation16.
Myoglobin monoclonal antibodies act as immunosensors by means of self-assembling gold nanoparticles into polyvinyl butyral adducts. It can measure the myoglobin interaction with its monoclonal antibody and has tremendous potential for biosensor development Citation17. The biosensors may be constructed from silicate sol--gels with encapsulated myoglobin proteins. These systems can detect biologically relevant analytes like O2. The recognition of dissolved oxygen is possible with use of myoglobin. Myoglobin can also bind carbon monoxide (CO), and sol--gels with entrapped myoglobin can be used as the sensor for CO by taking advantages of the changes in the absorption spectrum due to protein--CO interaction. The biosensor based on manganese myoglobin is able to detect nitric oxide.
6. Applications of myoglobin in imaging
The myoglobin content in normal, ischemic and necrotic myocardium is considered distinct by triphenyltetrazolium chloride staining, light and electron microscopy or the periodic acid-Schiff stain immunoperoxidase technique. Specifically, our laboratory has established the myoglobin measurement and its significant role in the assessment of myocardial infarction. The reasons may be coronary occlusion resulting in myoglobin loss in the fibers and turning necrotic. This can result in ischemia without necrosis. Thus necrosis can result in myoglobin loss from the myocardium. This observation suggests the efficacy of myoglobin based contrast agents as capable of distinguishing necrosis due to coronary occlusion Citation18.
The generation of myoglobin knockout (myo−/−) mice revealed the surprising result that these mice were viable and fertile and displayed no obvious signs of cardiac or skeletal dysfunction in absence of myoglobin Citation18. However, the value of myoglobin in non-invasive imaging and measurement is still not proven. To this end, there is need of myoglobin sensitive injectable imaging contrast agent that is independent of pH changes in the body target tissues. In our imaging experiments, a home made antimyoglobin iron oxide complex based superparamagnetic imaging contrast agent was prepared with phosphate buffer saline pH 7.4 and acetate buffer at pH 4.0. The imaging contrast agent in phantom tube showed MRI signal intensities distinct at both different pH ranges as shown in and .
Figure 7. (On left panel) The figure represents MRI images of a capillary filled with iron oxide--myoglobin at pH 7.4 in presence of phosphate buffered saline pH7.4. In the background, mice body cross-sectional single slice image is shown with heart image (at the botton on right) without contrast agent. (On right panel) Same capillary with myoglobin at pH 4.0 in presence of acetate buffer pH 4.0 shows loss of signal of iron--oxide--myoglobin shown with asterisk. The images were generated using multislice multiecho spin echo proton density weighted technique at scan parameters TE = 15 ms, TR = 1500 ms, matrix 256 × 256, NEX = 2, FOV = 2.5 cm at 11.7 T or 500 MHz MRI imager. In the background, a same cross-sectional slice image of mice image after anti-myoglobin-iron oxide superparamagnetic contrast injection with cardiac territories are shown by arrows and details of different heart chambers (marked as RA, LA, LV, RV) can be seen in insert. Notice the enhancement of vascular wall regions rich with anti-myoglobin bound superparamagnetic particles after injection at pH 7.4 while the same imaging contrast agent in the capillary turned darker at pH 4.0.
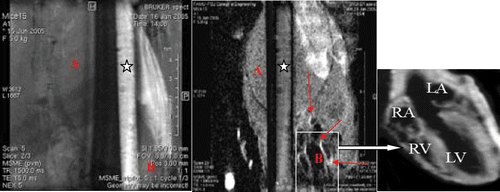
Table 2. The relationship of Raman spectra peaks and MRI signal intensity of phantom is represented to show reduced intensities by both Raman spectrum and MRI imaging. The reason for the reduced Raman spectra peaks of iron oxide--myoglobin in phantom was the low. pH while the reduced MRI signal was due to both low pH and dephasing with phase shifting characteristics of superparamagnetic iron oxide molecule. Notice the increased MRI signal of mouse body* (marked A) after injection of iron-oxide myoglobin contrast agent due to relative loss of signal at solid tissue mass while vascular or fluid mass (marked B) appeared brighter as shown in this table and by arrows.
In cardiac hypertrophy, marker proteins Arpp, Troponin C/I/T proteins are future candidates. Recently, Arpp protein containing an ankyrin repeat domain with proline-rich regions, received attention as a putative genetic marker for cardiac hypertrophy. These potential proteins are possible candidates in gene therapy of cardiac myopathy while they may be useful as in vivo cardiac biomarkers and contrast agents for monitoring gene therapy. Other Carp proteins in cardiac muscles have type I fibers. In adult cardiac muscle, interestingly, Arpp is mainly expressed in the ventricles, whereas Carp is expressed throughout the atrium and ventricle. The Arpp-expression may be associated with the differentiation stage during myogenesis Citation19. Another possible use of ferromagnetic immunoassay is to measure the cardiac troponin I Citation20. It remains to see which is the best choice for rapid imaging and biomarker detection of myocardiac infraction, myopathy and ischemia without the interference of pH and other physiological conditions.
7. Conclusion
The myoglobin encapsulation and iron-oxide bound antibody method may serve the purpose of developing a myoglobin sensitive MRI imaging contrast agent. Iron oxide binds with myoglobin and its oxidation behaviour is pH dependent and detectable by Raman spectroscopy. To develop pH independent superparamagnetic contrast agents, sol--gel encapsulation is the method of choice. The iron-oxide-biotinylated antimyoglobin complex can also be used in magnetic immunoassays and MRI contrast enhancement.
References
- Chatterjee , J , Haik , Y and Chen , CJ . 2001 . Synthesis and characterization of heat-stabilized albumin magnetic microspheres . Colloid Polym. Sci. , 279 ( 11 ) : 1073
- Bang's Laboratories . Technical Note 205 , Covalent Coupling. http://www.bangslabs.com/support/index.php
- Chatterjee , J , Yousef , H and Chen , CJ . 2002 . Polyethylene magnetic nanoparticle: a new magnetic material for biomedical applications . J. Magn. Magn. Mater. , 246 ( 3 ) : 382
- Haik , Y , Pai , V and Chen , CJ . 1999 . Development of Magnetic device for cell separation . J. Magn. Magn. Mater. , 194 : 254
- Dave , BC , Dunn , B , Selverstone Valentine , J and Zink , JI . 1994 . Sol--gel encapsulation methods for biosensors . Anal. Chem. , 66 : 1120A
- Harold , E , Wart , Van and Zimmer , J . 1985 . Resonance Raman evidence for the activation of dioxygen in horseradish oxyperoxidase . J. Biol. Chem. , 260 ( 14 ) : 8372
- Tang , Q , Kalsbeck , WA , Olson , JS and Bocian , DF . 1998 . Disruption of the heme iron-proximal histidine bond requires unfolding of deoxymyoglobin . Biochemistry , 37 : 7047
- Shikama , K . 1988 . Stability properties of dioxygen--iron(II) porphyrins: an overview from simple complexes to myoglobin . Coordinate Chem. Rev. , 83 : 73
- Shikama , K . 1985 . Nature of the FeO2 bonding in myoglobin: an overview from physical to clinical biochemistry . Experientia , 41 : 701
- Shikama , K . 2006 . Nature of the FeO2 bonding in myoglobin and hemoglobin: a new molecular paradigm . Prog. Biophys. Mol. Bio. , 91 : 83
- McCall , SL , Platzman , PM and Wolff , PA . 1980 . Surface enhanced Raman scattering . Phys. Lett. , 77A : 381
- Shalaev , VM . 2000 . Nonlinear Optics of Random Media: Fractal Composites and Metal-Dielectric Films , 75 – 96 . Heidelberg : Springer .
- Chatterjee , J , haik , Y and Chen , CJ . 2001 . Modification and characterization of polystyrene based magnetic micrpspheres and its composition with albumin based magnetic microspheres . J. Magn. Mag. Mat. , 225 : 21
- Haik , Y , Cordovaz , M and Chen , CJ . 2002 . Magnetic immunoassay for rapid assessment of acute myocardial infarction . Eur. J. Cells Mater. , 1 : 44
- Cardovaz , M . 2001 . Point of care diagnosis of acute myocardial infarction using magnetic immunoassays , Master's thesis, Florida State University .
- Ellerby , LM , Nishida , CR , Nishida , F , Yamanaka , SA , Dunn , B , Valentine , JS and Zink , JI . 1992 . Encapsulation of proteins in transparent porous silicate glasses prepared by the sol--gel method . Science , 255 : 1113
- Béatrice , L , Bouvier , AL and Loïc , J . 2005 . Biosensors for protein detection . Anal. Lett. , 38
- Schlieper , G , Kim , JH , Molojavyi , A , Jacoby , C , Laussmann , T , Flögel , U , Gödecke , A and Schrader , J . 2004 . Adaptation of the myoglobin knockout mouse to hypoxic stress . Am. J. Physiol. Regul. Integr. Comp. Physiol. , 286 : R786
- Ishiguro , N , Baba , T , Ishida , T , Takeuchi , K , Osaki , M , Araki , N and Okada , E . 2002 . Carp, a cardiac ankyrin-repeated protein, and its new homologue, Arpp, are differentially expressed in heart, skeletal muscle, and rhabdomyosarcomas . Am. J. Pathology , 160 ( 5 ) : 1767
- Davies , E , Gawad , Y , Takashashi , M , Shi , Q , Lam , P , Styba , G , Lau , A , Heesehen , C , Usategui , M and Jackowski , G . 1997 . Analytical performance and clinical utility of a sensitive immunoassay for determination of human cardiac troponin I . Clinical Biochem. , 30 ( 6 ) : 497