Abstract
Three different colloids of gold nanowire networks in pure water at room temperature were prepared by pulse laser ablation of a gold metal disc using different wavelength of Nd: YAG laser. The products were examined by transmission electron microscopy (TEM) and optical absorption spectroscopy. The TEM images of the products revealed that the attachment of gold nanowire to one another is more when it is prepared using shorter laser wavelength (355 nm) as compared with those prepared with longer laser wavelength (532 or 1064 nm). The results of the laser ablation of gold metal in pure water using three different laser wavelengths of Nd: YAG laser are compared with their corresponding TEM images.
1. Introduction
In the last decade, much work has been done to synthesise two-dimensional (2D) and three-dimensional (3D) well-arrayed structures, in which size-selected metal nanoparticles are bound to each other with ligands covering the metal nanoparticles Citation1–3. Lisiecki et al. Citation2 prepared interconnected networks of copper metallic particles by chemical reduction of a copper salt into metal nanoparticles in a micelle. Recently, organisation of nanoparticles into nanowires with a networked structure and investigation of the growth process has been a subject of active research due to its potential uses in microelectronics, optoelectronics, nanoscale electronic devices, and other fields Citation4–6. Although these studies succeeded in fabricating 2D nanostructure, a full understanding of the formation mechanism of the metallic nanowire still requires more studies related to its formation process.
A large variety of chemical methods of nanoparticles/nanonetworks preparation is known to date Citation1–10 but laser ablation Citation11,Citation12 of solids in liquid environment result in the formation of pure nano colloidal solutions containing no other ions or surface-active substances.
In this article, we report a simple and rapid laser ablation technique for the formation of gold nanowire networks of different structure in water solution in a single step by using different wavelength of Nd: YAG laser. Mafune et al. Citation13 and Mafune Citation14 have reported the formation of gold nanonetworks in two steps. In the first step, they prepared surfactant-free gold nanoparticles having an average diameter of ∼20 nm in pure water by laser ablation of gold metal plate using 1064 nm wavelength of Nd: YAG laser. In the second step, water containing surfactant-free gold nanoparticles prepared previously was mixed with an aqueous solution of sodium dodecyl sulfate, and this solution was irradiated with an intense pulsed laser of wavelength 532 or 355 nm. Here, we demonstrate the formation of gold nanowire networks in a single step by laser ablation at 1064, 532 or 355 nm of a gold metal disc in pure water without using any surfactant. Extensions of similar strategies could offer potential ways to tailor the nanowire aspect ratio and network connectivity and could open up new routes for forming porous mesoarchitectures that could serve as a self-supporting nanocatalyst and interconnected wiring for devices Citation5.
2. Experimental details
shows the experimental setup used in present study. Surfactant-free gold nanowires networks were prepared by laser ablation of gold metal disc (purity 99.99%, thickness 1 mm, and diameter 10 mm, Valcambi, Switzerland), which was placed on the glass holder immersed in the glass vessel filled with 10 mL pure water. The laser ablation was performed using the fundamental (1064 nm), second harmonic (532 nm), and third harmonic (355 nm) of INNOLAS Spit light 600 Nd: YAG pulsed laser (Pulse width ∼7 ns) operating at 10 Hz. The laser beam was focused using a quartz lens having a focal length of 200 mm. The spot size of the focused laser beam on the surface of the gold metal disc was typically 0.2 mm in diameter. During the laser ablation, each colloidal solution was homogenised by the magnetic stirrer so as to obtain a solution in which gold nanowire networks are homogeneously distributed in space. The laser energy used in the present study was 25 mJ per pulse. The ablation time was 60 min.
A Gentec Solo PE power meter was used to monitor the laser power. The colloids formed have been characterised by UV–visible spectroscopy (Perkin–Elmer lambda 35) and transmission electron microscopy (TEM) Tecnai-20 FEI operated at 200 kV.
3. Results and discussion
shows the optical absorption spectra of gold nanowire networks prepared by pulse laser ablation of gold metal disc in pure water using laser wavelength of 1064, 532, and 355 nm, respectively. The peak at 525 nm is assigned to the surface plasmon of the spherical part of the nanowire networks Citation13,Citation14. The broad tail emerged in the visible to the near-IR region is assigned to the surface plasmon of wires/rods. In addition to the plasmon peak at 525 nm an additional peak is also observed in the ultraviolet wavelength (∼265 nm), which can be attributed to the absorption due to the interband transition in Au nanostructures. In nanoparticles, the electronic energy levels are not continuous as in bulk materials, but discrete due to the quantum size effects of the careers and this can result in an absorption peak corresponding to the interband transitions in nanoparticles Citation15. An interband absorption can be possible at shorter wavelengths due to the transition of an electron from the occupied d-level state to an empty state in the conduction band above the Fermi level in noble metals (Au, Ag, Pt, etc.) Citation16. A weak and broad absorption peak corresponding to interband transition has been observed at a wavelength around 240–300 nm along with a strong plasmon peak around 525 nm in Au nanoparticles Citation17. In addition, theoretical investigations also show a considerable contribution of interband transition to the absorption spectra of Au and Ag nanoparticles at shorter wavelengths Citation18. Similar to previous work Citation17, in our case also, the intensity ratio ((intensity of the interband)/(intensity of plasmon peak)) increases with decrease in the particle size (). It is clear from that overall intensity of the absorbance is large in in comparison to ; therefore, similar to silver nanoparticles Citation19,Citation20, in the present study also, the concentration of the formation of the nanoparticles using three different wavelengths (1064, 532, and 355 nm) is not same because the ablation efficiency of the gold nanoparticles in the water increases with the increase in the laser wavelength. Further, the absorbance at 800 nm is almost zero when no nanowire networks are formed, while it is nonzero when the gold nanowire networks are formed Citation13. It is clear from , the absorbance at 800 nm is not zero which may be the indication of the formation of nanowire network. The intensity of the surface plasmon band at 525 nm gradually decreases, but the decrease in the absorbance near-IR region is less rapid with decreasing laser wavelength (). To clarify this statement, we measured the intensity ratio of absorbance at 800 nm with surface plasmon resonance at 525 nm and the values are tabulated in . It is clear from that the ratio is larger for 355 nm and smaller for 1064 nm laser irradiation, which clearly demonstrates that the formation of nanowire is prominent for 355 nm while it is less for 1064 nm laser irradiation.
Figure 2. Optical absorption spectra of gold nanowire networks prepared in pure water by laser ablation at (a) 1064 nm, (b) 532 nm, and (c) 355 nm.
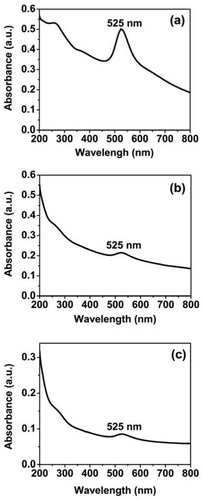
Table 1. Variation of intensity ratio ((Intensity of the interband)/(Intensity of plasmon peak)) with laser wavelengths.
Table 2. Ratio of the absorbance at two wavelengths 800 and 525 nm.
To prove the above statement we analysed these three solutions by TEM, and their electron micrographs are shown in the . In all the gold nanoparticles are interconnected to the gold nanowires and formed a network structure, while in , the gold nanowires seem to be more attached to each other, typically 25–500 nm long and 2–6 nm in diameter. Thus, our results reveal that in laser ablation technique, the geometrical structures (networks formation) of the gold nanoparticles were strongly dependent on the wavelength of the laser.
Figure 3. Electron micrographs of gold nanowire networks produced by laser ablation at (a) and (b) 1064 nm, (c) and (d) 532 nm, and (e) and (f) 355 nm.
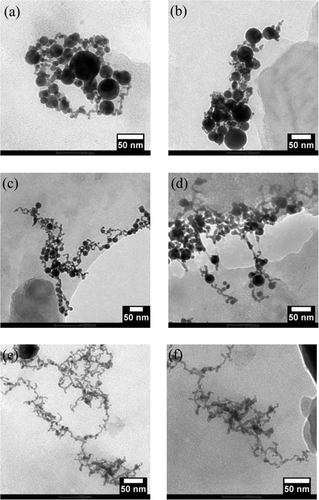
shows the selected-area electron diffraction (SAED) pattern of gold nanowire networks, which clearly demonstrates that the characteristic ring in the polycrystalline diffraction pattern can be indexed to (111), (200), (220), and (311) crystalline facets, creating concentric rings with sequences corresponding to a face-centered cubic lattice gold phase Citation10.
The gold nanowires connecting the gold nanoparticles are likely to form via aggregation growth rather than crystal growth Citation21. The spherical gold nanoparticles formed initially aggregate like a pearl chain, which are clearly observed in the TEM micrographs of . With 355 nm laser ablation, gold nanoparticles agglomerates were produced that had small diameter of nanowires with better network connectivity (). The fragmentation of initially formed gold nanoparticles at each laser fluence is higher for 355 nm, less for 1064 nm and intermediate for 532 nm, and this can be explained with the help of the absorbance efficiency of the solution at different wavelength. It is clear from the absorption spectra of solutions containing gold nanoparticles () that the absorbance in UV region is large in comparison to visible and near-IR region. Therefore, the probability of absorbance by the gold nanoparticles at 355 nm is expected to exceed to that of the 532 or 1064 nm and due to this, nanoparticle tends to agglomerate more for 355 nm and less for 1064 nm, while intermediate for 532 nm. Thus, the structure of nanowire networks of gold in the water depends on laser wavelength, and length of the gold nanowire networks increase with the lower laser wavelength.
4. Conclusions
A simple strategy for synthesising gold nanowire networks with desire sizes and structures using laser ablation of gold metal disc in pure water at different wavelengths of Nd: YAG laser in single step has been demonstrated. Our results show that the laser wavelengths play an important role in the formation of nanowire networks. The structure of gold nanowire networks, such as the size of the particles and the size of the nanowire networks, can be controlled by the laser wavelength in laser ablation method. Extending our methodology to other material systems would open up the possibility of synthesis of new mesoscale structures from nanounits, for applications such as self-supporting catalyst networks and nanowires for devices.
Acknowledgements
The authors are thankful to Dr S.B. Rai (Department of Physics, B.H.U.), Dr Ramesh Chandra (IIT Roorkee) and Dr A.C. Pandey (Nanophosphor Application Center, University of Allahabad) for providing Laser, TEM and UV–Vis facilities, respectively. The financial assisstance from DRPO, New Delhi (Project No.–ERIP/ER/04303481/M/01/787) is duly acknowledged.
References
- Pileni , MP . 1993 . Reverse micelles as microreactors . J. Phys. Chem. , 97 : 6961 – 6973 .
- Lisiecki , I , Biooludet , F and Pileni , MP . 1996 . Control of the shape and the size of copper metallic particles . J. Phys. Chem. , 100 : 4160 – 4166 .
- Taleb , A , Petit , C and Pileni , MP . 1998 . Optical properties of self-assembled 2D and 3D superlattices of silver nanoparticles . J. Phys. Chem. B , 102 : 2214 – 2220 .
- Xia , Y , Yang , P , Sun , Y , Wu , Y , Mayers , B , Gates , B , Yin , Y , Kim , F and Yan , H . 2003 . One-dimensional nanostructures: Synthesis, characterization, and applications . Adv. Mater. , 5 : 353 – 389 .
- Ramanath , G , D’Arcy-Gall , J , Maddanimath , T , Ellis , AV , Ganesan , PG , Goswami , R , Kumar , A and Vijayamohanan , K . 2004 . Templateless room-temperature assembly of nanowire networks from nanoparticles . Langmuir , 20 : 5583 – 5587 .
- Gu , Z , Ye , H , Bernfeld , A , Livi , KJT and Gracias , DH . 2007 . Three-dimensional electrically interconnected nanowire networks formed by diffusion bonding . Langmuir , 23 : 979 – 982 .
- Hansen , CR , Westerlund , F , Poulsen , KM , Ravindranath , R , Valiyaveettil , S and Bjornholm , T . 2008 . Polymer-templated self-assembly of a 2-dimensional gold nanoparticle network . Langmuir , 24 : 3905 – 3910 .
- Pei , L , Mori , K and Adachi , M . 2004 . Formation process of two-dimensional networked gold nanowires by citrate reduction of AuCl4 − and the shape stabilization . Langmuir , 20 : 7837 – 7843 .
- Sakai , T and Alexandridis , P . 2006 . Facile preparation of Ag–Au bimetallic nanonetworks . Mater. Lett. , 60 : 1983 – 1986 .
- Huang , CJ , Chiu , PH , Wang , YH , Yang , CF and Feng , SW . 2007 . Electrochemical formation of crooked gold nanorods and gold networked structures by the additive organic solvent . J. Colloid Interf. Sci. , 306 : 56 – 65 .
- Neddersen , J , Chumanov , G and Cotton , TM . 1993 . Laser ablation of metals: A new method for preparing SERS active colloids . Appl. Spectrosc. , 47 : 1959 – 1964 .
- Henglein , A . 1993 . Physicochemical properties of small metal particles in solution: “Microelectrode” reactions, chemisorption, composite metal particles, and the atom-to-metal transition . J. Phys. Chem. , 97 : 5457 – 5471 .
- Mafune , F , Kohno , JY , Takeda , Y and Kondow , T . 2003 . Formation of gold nanonetworks and small gold nanoparticles by irradiation of intense pulsed laser onto gold nanoparticles . J. Phys. Chem. B , 107 : 12589 – 12596 .
- Mafune , F . 2004 . Structure diagram of gold nanoparticles in solution under irradiation of UV pulse laser . Chem. Phys. Lett. , 397 : 133 – 137 .
- Schaaff , TG , Shafigullin , MN , Kohoury , JT , Vegmar , I , Whetten , RL , Cullen , WG , First , PN , Wing , CG , Ascensio , J and Jose-Yacaman , MJ . 1997 . Isolation of smaller nanocrystal Au molecules: Robust quantum effects in optical spectra . J. Phys. Chem. B , 101 : 7885 – 7891 .
- Alvarez , MM , Khoury , JT , Schaaff , TG , Shafigullin , MN , Vezmar , I and Whetten , RL . 1997 . Optical absorption spectra of nanocrystal gold molecules . J. Phys. Chem. B , 101 : 3706 – 3712 .
- Balamurugana , B and Maruyama , T . 2005 . Evidence of an enhanced interband absorption in Au nanoparticles: Size-dependent electronic structure and optical properties . Appl. Phys. Lett. , 87 : 143105
- Pinchuk , A , Plessen , GV and Kreibig , U . 2004 . Influence of interband electronic transitions on the optical absorption in metallic nanoparticles . J. Phys. D , 37 : 3133 – 3139 .
- Tsuji , T , Iryo , K , Nishimura , Y and Tsuji , M . 2001 . Preparation of metal colloids by a laser ablation technique in solution: Influence of laser wavelength on the ablation efficiency (II) . J. Photoch. Photobio A , 145 : 201 – 207 .
- Tsuji , T , Iryo , K , Watanabe , N and Tsuji , M . 2002 . Preparation of silver nanoparticles by laser ablation in solution: Influence of laser wavelength on particle size . Appl. Surf. Sci. , 202 : 80 – 85 .
- Zhang , DB , Qi , LM , Ma , JM and Cheng , HM . 2001 . Formation of silver nanowires in aqueous solutions of a double-hydrophilic block copolymer . Chem. Mater. , 13 : 2753 – 2755 .