Abstract
Cobalt ferrite (CoFe2O4) nanoparticles were prepared through an auto-combustion method. The results of XRD show that the crystallite size of CoFe2O4 nanoparticles increases from 57 to 105 nm with a slight change in lattice constant as the annealing temperature increases from 300 to 1200°C. The magnetic measurements by vibrating sample magnetometer (VSM) show that M s increases monotonously by increasing the annealing temperature from 300 to 1200°C and C hB and M s reached a maximum when annealing temperature is about 400°C due to the change of the grain size.
1. Introduction
Cobalt ferrite (CoFe2O4), a kind of inverse-spinel oxide, which belongs to the square group (Fd3m), has been widely investigated because of its cubic magnetrocrystalline anisotropy, relatively high H
c, moderate saturation magnetisation (M
s), good chemical stability Citation1,Citation2 and broad prospect of applications in several technological fields, including electronic devices, ferrofluids and high-density information storage Citation3–6. Various methods, including co-precipitation Citation7,Citation8, hydrothermal synthesis Citation9,Citation10, sol–gel techniques Citation11, microemulsion method Citation12,Citation13, forced hydrolysis method Citation14 and sonochemical reaction Citation15, have been reported for preparing CoFe2O4. Most of these methods suffer with troublesome post-treatment and cannot be applied to a large-scale and economic production. Some researches Citation16,Citation17 have prepared nanoparticles of CoFe2O4 using auto-combustion method. It is noticed that this method stands out as an alternative and highly promising method for synthesis of CoFe2O4. However, problems still exist. For instance, NH4OH was added into the starting solution to adjust pH, which was thought to be a necessary step, but this step induces the existence of some byproducts, such as NH4
+ and Co2O3
Citation17.
In this article, single-phase CoFe2O4 nanoparticles with rare by-products were prepared by auto-combustion method without the addition of NH4OH. The as-prepared powder of CoFe2O4 is relatively small, which is convenient for us to control the crystallite size by subsequent heat treatment. It is known that most of the physical properties of CoFe2O4 spinel depend strongly on the shape and size of the nanoparticles. Thus, the other purpose of this work is to investigate the dependence of magnetic properties on crystallite size of CoFe2O4 nanoparticles.
2. Experimental procedure
First, the analytical grade reagent of citric acid (CA 99.5%), Fe(NO3)3 · 9H2O (98.5%) and Co(NO3)2 · 6H2O (99%) were dissolved in distilled water. The initial ratio was Co2+ : Fe3+ = 1 : 2 and CA : metal ions = 1 : 1. The pH of the solution was about 2. The mixed solution was then heated on a high-power heating device. With the evaporation of H2O, the solution became viscous gel and combusted finally. This combusting process just lasts for several seconds and at last black fleecy powder is formed. The as-burnt powders were then annealed at 300, 400, 600, 700, 900 and 1200°C, respectively.
X-ray fluorescence spectrometry (XFS S4 PIONEER) and X-ray photoelectron spectroscopy (XPS XSAM 800 Krato. Ltd) were used to determine the atomic percentage of the as-burnt powders and showed that the molar ratio of Co : Fe : O is very close to 1 : 2 : 4. The structure was determined from the X-ray diffraction (XRD D8-Advance Cu-Kα). The average crystallite sizes were calculated by using (311) peaks and Scherrer's formula. Fourier transform infrared (FT-IR) transmission spectra were taken on NICOLET 5700 Infrared Spectrometer from 4000 to 400 cm−1. Raman spectra were carried out using RM 1000 Raman microscope. The microstructure and morphology of the powders annealed at 400°C were specified by transmission electron microscopy (TEM JEM2010). The magnetic hysteresis loops of all samples were collected at room temperature by using vibrating sample magnetometer (VSM).
3. Results and discussion
The typical FT-IR spectrum of the as-burnt powder is as shown in . The intensive broad band at 3430 cm−1 is associated with the O–H stretching vibration. The band at 1623 cm−1 is due to the H–O–H bending vibration. This indicates the presence of residual water, which may be ascribed to the physical absorption of H2O by the as-burnt powder. The band at 1100 cm−1 comes from the coupling of the O–H bending vibration and stretching vibration of C–O. This indicates the existence of–COOH. The bands at 1384 and 1452 cm−1 are associated with the bending vibration of C–H of–CH2. The band at 2330 cm−1 is ascribed to the stretching vibration of C–C. The bands at 2860 and 2925 cm−1 are ascribed to the stretching vibration and anti-symmetric stretching vibration of C–H and–CH2, respectively. It is clear that the as-prepared powder contains some organic products. The wide absorption band at 570 cm−1 corresponds to the character band of Fe–O stretching vibration mode in CoFe2O4 compounds. It indicates the formation of CoFe2O4 compound in the as-burnt powder. However, the band associated with Co–O stretching vibration in CoFe2O4 compounds, which is generally around 700 cm−1, is undistinguished due to its weak absorption and usually being covered by the wide absorption of Fe–O stretching vibration.
shows the XPS core spectra of Fe and Co for the as-burnt powders. The peak of Fe 2p3/2 has an asymmetric shape with the maximum around 711.2 eV and a broaden shoulder at 713.4 eV. Both peaks have the characteristic of +3 value, and can be ascribed to Fe3+ ions in octahedral and tetrahedral sites, respectively. The XPS spectrum of Co 2p3/2 in CoFe2O4 also exhibits asymmetry due to the two sites of Co2+ ions, in octahedral and tetrahedral sites. It is noted that intense satellite structure is found on the high binding energy side of the Co 2p3/2 and Co 2p1/2, which is believed to be a direct evidence of the band structure associated with octahedral Co2+ in the oxide lattice.
The XRD patterns of the as-burnt sample as well as the samples annealed at various temperatures are depicted in . It is clear that all patterns are consistent very well consistent with the data of CoFe2O4 spinel (JCPDS cards No. 22-1086). The intensity of all peaks increases while the full-width at half maximum (FWHM) decreases with the increase of the annealing temperature. In order to evaluate the influence of annealing temperature, the lattice constants were calculated by least squares, and the average crystallite size were determined from the FWHM of the (311) peak using the Scherrer's formula. The results are shown in . It is noted that the lattice constants show little change while the average crystallite size increases from 57 to 105 nm as the annealing temperature increases from 300 to 1200°C. It indicates that the annealing temperature has little influence on the crystal structure but increases the crystallite size.
Figure 3. (a) The XRD patterns of the as-burnt powder and the sample annealed at 300, 400, 600, 700, 900 and 1200°C, respectively. (b) Particle size and lattice constant vs. annealing temperature.
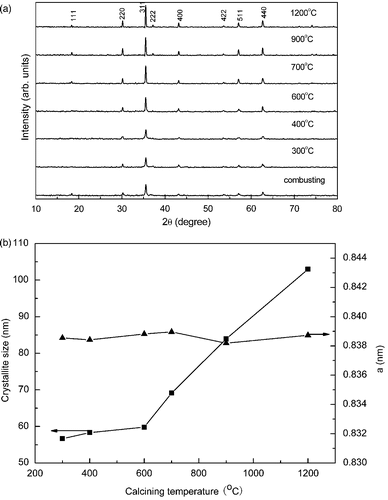
However, it is hard to conclude that all the samples are single-phase CoFe2O4 due to the similar spinel structure of CoFe2O4 and γ-Fe2O3 Citation18. It is known that the Raman spectra of γ-Fe2O3 exhibit much stronger Raman peaks at 1378 and 1576 cm−1 than that of CoFe2O4 Citation19. So the Raman spectra are introduced for further confirmation. The Raman spectra of the samples annealed at temperature from 300 to 1200°C were carried out and no peaks were observed above 1000 cm−1. It suggests that the formation of γ-Fe2O3 is unlikely and the samples are at single CoFe2O4 phases.
CoFe2O4 has a cubic inverse-spinel structure and belongs to the square group (Fd3m) Citation20,Citation21. The factor group analysis predicts the following modes in spinel:
shows the TEM images for the morphology of CoFe2O4 nanoparticles annealed at 400°C. It shows that there is obvious boundary in most regions. Particles of rough, irregular but generally spheroidal shapes are observed. The average particle size observed by TEM is 20–30 nm, which is much smaller than that obtained by XRD observations. It is known that aggregation is common in this system due to the strong interaction between CoFe2O4 magnetic nanoparticles. Obviously, this aggregation combining with the annealing process results in the increasing of the average particle size of CoFe2O4 nanoparticles. shows the selected diffraction patterns of the same sample and the results calculated from the electron diffraction formula is given in , which is well consistent with the data of XRD.
Figure 5. (a) The TEM images of the CoFe2O4 nanoparticles annealed at 400°C. (b) The selected diffraction patterns of the same sample.
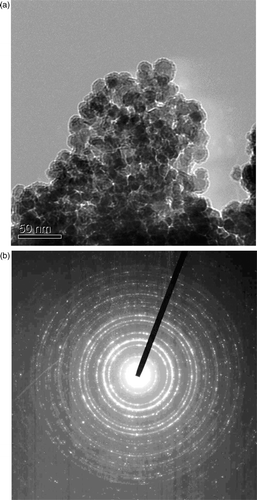
Table 1. Values calculated from the SAD pattern of the CoFe2O4 nanoparticles annealed at 400°C.
The results of VSM measurement at room temperature are shown in . It is obviously that the magnetic properties of CoFe2O4 powders show high dependence upon the annealing temperature. The M s increases with the increase of the annealing temperature while the remanance ratio and the coercivity H c reach its maximum value at 400°C. It indicates that the transition of M s is highly depends upon the growth of CoFe2O4 nanocrystallites. Because both the crystallite size and the M s have the same changing trend with the increasing of the annealing temperature. Also, it indicates that the size of CoFe2O4 nanoparticles is not only the factor in deciding H c and the remanance ratio. It was reported that H c is closely related to the microstructure, grain size, residual stain and many other complex factors Citation24,Citation25. As the particle size is around the single domain size, the H c will reach a maximum value because of the coherent rotation of spins Citation22. This indicates that the crystalline size of the CoFe2O4 powder annealed at 400°C in this work may come close to the size of single domain. The drop of H c at 600°C and the drastic drop when the CoFe2O4 powder annealed at temperatures above 700°C might originate from the transition of magnetic single domains to multi-domain within a particle.
4. Conclusion
In conclusion, single-phase CoFe2O4 nanoparticles with different grain size have been successfully synthesised by a rapid auto-combustion method.
The results of XRD show that the crystallite size of CoFe2O4 increases from 57 to 105 nm with a slight change in lattice constant as the annealing temperature increases from 300 to 1200°C. Magnetic measurements show that M s increases monotonously by increasing the annealing temperature from 300 to 1200°C, while the H c and M s reached a maximum when annealing temperature is at 400°C due to the change of the grain size.
Acknowledgements
The authors would like to thank Z.Y. Kuang and Z. Li of Huazhong University of Science and Technology for their help with the magnetic measurements. This work is supported by the Chinese National Foundation of Natural Science (No. 10674105), National Basic Research Program of China (2007CB607501) and a financed program of Provincial Key Laboratory of Organic Polymer Optoelectronic Materials, Hubei, China.
References
- Dorsey , PC , Lubitz , P , Chrisey , KB and Horwitz , JS . 1996 . CoFe2O4 thin films grown on (100) MgO substrates using pulsed laser deposition . J. Appl. Phys. , 79 : 6338 – 6340 .
- Lee , JG , Park , JY , Oh , YJ and Kim , CS . 1998 . Magnetic properties of CoFe2O4 thin films prepared by a sol-gel method . J. Appl. Phys. , 84 : 2801 – 2804 .
- Kitamoto , Y , Kantake , S , Shirasaki , F , Abe , M and Naoe , M . 1999 . Co ferrite films with excellent perpendicular magnetic anisotropy and high coercivity deposited at low temperature . J. Appl. Phys. , 85 : 4708 – 4710 .
- Sousa , MH and Tourinho , FA . 2001 . New electric double-layered magnetic fluids based on copper, nickel, and zinc ferrite nanostructures . J. Phys. Chem. B , 105 : 1168 – 1175 .
- Speliotis , DE . 1999 . Magnetic recording beyond the first 100 Years . J. Magn. Magn. Mater. , 193 : 29 – 35 .
- Mazaleyrat , F and Varga , LK . 2000 . Ferromagnetic nanocomposites . J. Magn. Magn. Mater. , 215–216 : 253 – 259 .
- Grigorova , M , Blythe , HJ , Blaskov , V and Rusanov , V . 1998 . Magnetic properties and Mössbauer spectra of nanosized CoFe2O4 powders . J. Magn. Magn. Mater. , 183 : 163 – 172 .
- Chinnasamy , CN , Senoue , M , Jeyadevan , B , Perales-Perez , O , Shinoda , K and Tohji , K . 2003 . Synthesis of size-controlled cobalt ferrite particles with high coercivity and squareness ratio . J. Colloid Interface Sci. , 263 : 80 – 83 .
- Morais , PC , Garg , VK , Oliveira , AC and Silva , LP . 2001 . Synthesis and characterization of size-controlled cobalt-ferrite-based ionic ferrofluids . J. Magn. Magn. Mater. , 255 : 37 – 40 .
- Cabanãs , A and Poliakoff , M . 2001 . The continuous hydrothermal synthesis of nano-particulate ferrites in near critical and supercritical water . J. Mater. Chem. , 11 : 1408 – 1416 .
- Lee , JG , Park , JY and Kim , CS . 1998 . Growth of ultra-fine cobalt ferrite particles by a sol-gel method and their magnetic properties . J. Mater. Sci. , 33 : 3965 – 3968 .
- Moumen , N and Pileni , MP . 1996 . New syntheses of cobalt ferrite particles in the range 2–5 nm: Comparison of the magnetic properties of the nanosized particles in dispersed fluid or in powder form . Chem. Mater. , 8 : 1128 – 1134 .
- Ahn , Y , Choi , EJ , Kim , S and Ok , HN . 2001 . Magnetization and Mössbauer study of cobalt ferrite particles from nanophase cobalt iron carbonate . Mater. Lett. , 50 : 47 – 52 .
- Hanh , N , Quy , OK , Thuy , NP , Tung , LD and Spinu , L . 2003 . Synthesis of cobalt ferrite nanocrystallites by the forced hydrolysis method and investigation of their magnetic properties . Physica B , 327 : 382 – 384 .
- Shafi , KVPM , Gedanken , A , Prozorov , R and Balogh , J . 1998 . Sonochemical preparation and size-dependent properties of nanostructured CoFe2O4 particles . Chem. Mater. , 10 : 3445 – 3450 .
- Xiao , SH , Jiang , WF , Li , LY and Li , XJ . 2007 . Low-temperature auto-combustion synthesis and magnetic properties of cobalt ferrite nanopowder . Mater. Chem. Phys. , 106 : 82 – 87 .
- Cannas , C , Falqui , A , Musinu , A , Peddis , D and Piccaluga , G . 2006 . CoFe2O4 nanocrystalline powders prepared by citrate-gel methods: Synthesis, structure and magnetic properties . J. Nanoparticle Res. , 8 : 255 – 267 .
- Tang , DP , Yuan , R , Chai , YQ and An , HZ . 2000 . Magnetic-core/porous-shell CoFe2O4/SiO2 composite nanoparticles as immobilized affinity supports for clinical immunoassays . Adv. Funct. Mater. , 17 : 976 – 982 .
- Sousa , MH , Tourinho , FA and Rubim , JC . 2000 . Use of Raman micro-spectroscopy in the characterization of M(II)Fe2O4 (M = Fe, Zn) electric double layer ferrofluids . J. Raman Spectrosc. , 31 : 185 – 191 .
- Wang , ZW , Lazor , P , Saxena , SK and O’Neill , HSC . 2002 . High pressure Raman spectroscopy of ferrite MgFe2O4 . Mater. Res. Bull. , 37 : 1589 – 1602 .
- Yu , T , Shen , ZX , Shi , Y and Ding , J . 2002 . Cation migration and magnetic ordering in spinel CoFe2O4 powder: micro-Raman scattering study . J. Phys. Condens. Mater. , 14 : L613 – L618 .
- Qu , YQ , Ying , HB , Yang , N , Fan , YZ , Zhu , HY and Zou , GT . 2006 . The effect of reaction temperature on the particle size, structure and magnetic properties of coprecipitated CoFe2O4 nanoparticles . Mater. Lett. , 60 : 3548 – 3552 .
- Wang , ZW , Downs , RT , Pischedda , V , Shetty , R , Saxena , SK , Zha , CS and Zhao , YS . 2003 . High-pressure x-ray diffraction and Raman spectroscopic studies of the tetragonal spinel CoFe2O4 . Phys. Rev. B , 68 : 094101
- Liu , BH and Ding , J . 2006 . Strain-induced high coercivity in CoFe2O4 powders . Appl. Phys. Lett. , 88 : 042506
- Wang , YC , Ding , J , Yi , JB , Liu , BH , Yu , T and Shen , ZX . 2004 . High-coercivity Co-ferrite thin films on (100)-SiO2 substrate . Appl. Phys. Lett. , 84 : 2596 – 2598 .