Abstract
We report a facile and effective way to prepare cell labelling quantum dots (QDs) using cetyltrimethylammonium bromide (CTAB) and to enhance biocompatibility of the QD labels. There are several reports on the use of this ligand to encapsulate nanoparticles including QDs. However, due to its high cellular toxicity, CTAB has still not been employed to prepare QDs for cellular labelling. After removing the free ligand by dialysis, we could successfully use CdSe/CdS/ZnS (core/shell/shell) QDs for cellular labelling. In addition, we found that the simple introduction of a sonication step to cause the emulsion of the QDs in the aqueous surfactant solution could lead to a five-times higher encapsulation of the QDs as compared to other methods. Fluorescent microscopy images of HeLa cells revealed that the QDs were evenly dispersed inside them. Furthermore, fluorescent morphological images of the QD labelled cells were more distinct than bright field images.
1. Introduction
During recent decades, new biological and medical applications of quantum dots (QDs) have been explored because of their superior photostability, multiplexing feasibility and high emission cross section in the near infrared region Citation1–3. Tracking the development of frog embryo cells and cancer metastasis or guiding the surgical resection of deep lymphatic nodes represent unique applications of the inorganic fluorescent labels Citation4–6. The success of such studies significantly relies on appropriate surface treatment of the QDs to meet criteria such as stable suspension, biocompatibility and sustained optical properties in biological environments. The use of cationic lipids or ligands is one of the most effective ways to prepare cellular labelling QDs because of the persistent quantum yields and no additional steps required for conjugation with peptides or antibodies Citation5,Citation7,Citation8. In spite of the successful fluorescent cellular labelling achieved with those ligands, there are still some limitations, such as indistinct cellular morphology on fluorescent images, the small exclusion size for encapsulating the QDs and the relatively complex composition of the system Citation5,Citation7,Citation8. However, for a practical and favourable use of the nanocrystals in biological or medical applications, an easy and high yield way to produce QD labels is needed. There have been several reports on the size independence of nanoparticles including QDs, gold nanoparticles and nanorods using cetyltrimethylammonium bromide (CTAB) micelles Citation7,Citation9,Citation10. Due to the high solubility and the simple and fast steps involved in preparing water soluble nanoparticles using CTAB, encapsulation of QDs by CTAB is expected to be an adaptable way to produce highly concentrated QDs in an aqueous buffer. However, until now, there has been no report of CTAB-micelle QDs (CTAB QDs) as intracellular labels because of the cytotoxicity of CTAB. Therefore, herein we show a way to prepare CTAB QDs as fluorescent cellular labels by effectively removing the free CTAB. By introducing a short sonication step, we were able to achieve a yield of about 90% from highly concentrated QDs within 20 min. The formation of CTAB QDs was independent of the size of the QDs and did not alter their optical properties.
2. Materials and methods
2.1. Preparation of cellular labelling QDs
The CdSe/CdS/ZnS (core/shell/shell) QDs were synthesised following the procedures reported previously Citation11. Before mixing them with CTAB solution, QDs were first precipitated three times with methanol to remove any free octadecylamine present in the solution and then redispersed in chloroform. The CTAB solution was prepared by dissolving 0.4 g of CTAB (Fluka, USA) in 10 ml of Millipore water. The solution containing CTAB and the QDs in chloroform (10 : 1 v/v) was then mixed vigorously until an emulsion was formed. Sonication of the emulsion was carried out in an ultrasonic cleaner (Branson, Model 3510-DTH, USA). Then, the emulsion was heated at 80°C for 10 min (until complete evaporation of chloroform). The CTAB QD suspension was filtrated through a 0.2 µm pore regenerated cellulose membrane (Millipore, USA). Unincorporated free CTAB was removed by cooling the CTAB QD suspension at 4°C and dialysing it with Amicon ultra centrifugal filter units (molecular weight cut off = 30 kD, Millipore, USA) against water. Dynamic light scattering data was obtained for estimating hydrodynamic size of CTAB QDs by Zetasizer Nano (Malven Instruments Ltd., England). Absorbance and emission spectra of the CTAB QDs were obtained using Agilent 8453 (Agilent, USA) and FL-1039 (Jobin Yvon Inc., USA), respectively.
2.2. Measuring cellular toxicity of CTAB QDs
The CTAB QD cytotoxicity effects were evaluated using the standard 3-(4, 5-dimethylthiazol-2-yl)-2,5-diphenyl tetrazolium bromide (MTT) assays. Briefly, HeLa cells (104 cells/ml) were treated with the QDs (1 M–100 nM) for 4 h before adding the MTT solution. After an incubation period of 4 h, the UV-Vis absorption spectrum was measured at 550 nm with 670 nm as the reference wavelength.
2.3. Cell labelling
The HeLa cells (ATCC number: CCL-2) were cultured in Opti-MEM (Gibco, Invitrogen, USA) supplemented with 10% fetal calf serum in a humidified 5% CO2 atmosphere at 37°C. Twenty-four hours after seeding, the cells (104 cells/ml) were rinsed with the medium and the CTAB QD solution (1 nM–50 nM) was added. All fluorescence images were recorded using a Zeiss LSM 510 confocal microscope (Carl Zeiss AG, Germany) with an excitation wavelength of 488 nm and an emission wavelength of >505 nm.
3. Results and discussion
3.1. Effect of sonication on preparation of the CTAB QDs
Stable CTAB QD aqueous suspension is believed to form through the transfer of QDs from the chloroform in the water emulsion to the water phase during evaporation of the organic solvent Citation5. Hydrophobic interdigitation between the primary alkane of the stabilising ligands of the QDs and the secondary alkane of the surfactant is the driving force of this transfer. Therefore, a finer size of the emulsion could facilitate formation of a more stable aqueous suspension of QDs. In general, sonication is used to prepare finer emulsion droplets or micelles in the development of drug-delivering polymeric microspheres Citation12,Citation13. Likewise, we achieved a significant difference in the optical density of the final CTAB QD suspension depending on whether sonication was performed (). No changes in profiles of the absorption and emission spectra were observed (). When a 10-min sonication power was provided to the emulsion, most of CTAB QD suspension was recovered after filtration through a 0.2-μm pore membrane filter (). However, without the sonication, the optical density of the QD suspension was only 25% that of the initial QD in chloroform at the first excitonic absorbance peak. The optical photograph in clearly shows the dramatic effect of the sonication step on the production yield of the CTAB QD suspension. It is worth noting that the CTAB QD suspension can be obtained from a dilute solution of QDs in the organic solvent without sonication (data not shown). However, sonication is necessary to stimulate the emulsion formation from a CTAB solution with higher concentrations of QDs. Further preparation of CTAB QDs from more concentrated QD organic suspensions () and their size analysis () support the above postulation regarding the effect of finer emulsions on the formation of the CTAB QD suspension. The size of the particles in a micelle-QD suspension sonicated for 3 min was about two times that of the original QDs in the organic solvent, whereas there was no significant size increase in the case of the sample sonicated for 10 min. The quantum yield of the CTAB QDs was nearly 90% of the original one. Another remark is that the size of the QDs in the CTAB micelles was about 10 nm, which is approximately twice the size of lipid bilayer QD hybrids. The larger size of our encapsulated CTAB QDs highlights their potential use as multicolour cellular labels Citation8. Compared to other encapsulating cationic ligands (), the sonication assisted encapsulation of QDs inside CTAB micelles is the most practical way to produce cellular labelling QDs (with a processing time at least six-times faster and a five-fold higher quantity of QDs).
Figure 1. (a) Absorption and (b) emission spectra of a QD solution (870 nM) in chloroform (blue) and of a CTAB QD suspension with (green) and without (red) sonication. (c) Optical photograph of filtrated CTAB QDs with (left) and without (right) sonication. (d) Optical photograph of a suspension of CTAB QDs (2.6 µM) without sonication (left) as well as with 3- (middle) and 10-min (right) of sonication.
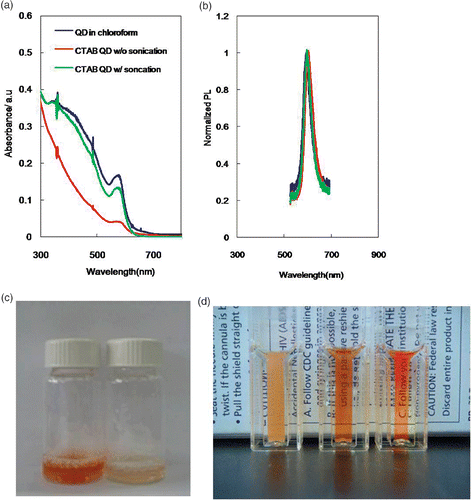
Table 1. Hydrodynamic sizes of CTAB QDs depending on the sonication time.
Table 2. Conditions for producing cellular labelling QDs.
3.2. Cytotoxicity of the CTAB QDs
The toxic effect of the CTAB QD suspension on cells was evaluated using the MTT assay (). As shown in , there was a noticeable difference in the cellular toxicity of the CTAB QDs depending on whether the free CTAB removal step was applied. Without this step, only 1 nM CTAB QD suspension resulted in a 15% decrease in cell viability. After removing the free CTAB from the CTAB QD solution by dialysis, the cell viability increased two-fold. The biocompatibility of the thus obtained CTAB QDs is comparable to, or even better than that of poly(ethylene glycol)-grafted poly(ethylene imine) (PEG-g-PEI) coated QDs Citation14. There was about 10% reduction in the cell viability 1 nM PEG-g-PEI QDs, while 10 nM CTAB QDs showed the same reduction, one order of magnitude less cytotoxicity than that of PEG-g-PEI QDs.
3.3. Cellular labelling efficiency of CTAB QDs
To evaluate the CTAB QD suspension as an effective intracellular fluorescent label, we compared the uptake of the hybrid with Lipofectamine™-treated QDs in cultured HeLa cells. As shown in , uniform accumulation of QDs inside the cells was observed. The fluorescent intensity of the images was proportional to the amount of CTAB QDs. The well dispersed fluorescence inside the cells is a distinctive feature of the CTAB QD suspension, whereas the Lipofectamine™-treated QDs sporadically accumulate in an uneven way (). Also, the fluorescence intensity of cultured cells treated with 10 nM CTAB QDs is almost equal to that of cells treated with 100 nM Lipofectamine™. Another remark is that the morphological fluorescence images of HeLa cells cultured with 10 and 50 nM CTAB QD suspension are sharper than the bright field microscopy images of the same cell cultures. It has been reported that cationic liposomes may enhance the DNA transfection efficiency because disruption of endosome by the cationic liposomes leads to a release of DNA into cytoplasm Citation14,Citation15. The uniform accumulation of CTAB QDs can be compared with this cationic effect to destabilise endosomes. The cell staining properties of the CTAB QDs are basically similar to those described in other reports for systems where cationic ligand coated QDs are used Citation5,Citation7,Citation8,Citation16. Among those reports, the morphological distinction obtained herein is comparable to that of lipid bilayer incorporated QDs even using a five-fold reduced amount of QDs and superior to that of LipofectamineTM-treated QDs Citation5,Citation8.
4. Conclusions
We have showed a way for high-yield production of optically clear, highly concentrated CTAB QD suspensions by simply introducing an additional sonication step to the conventional procedure, and a potential of CTAB QDs as effective cellular fluorescent labels by effectively removing the free CTAB from CTAB QD. This is the first demonstration of the feasibility of using CTAB QDs for cellular staining, in particular by showing the morphological distinction of the cultured cells. No cytotoxicity was observed even at a concentration of the CTAB gold nanoparticles as high as 1 µM after removing the free CTAB from the suspension by centrifugation Citation9,Citation10. Therefore, there is still room for improving the biocompatibility of the CTAB QD suspension at higher concentrations. We expect that this first-time application of CTAB QDs to fluorescent cellular labelling could lead to further applications of these QDs as fluorescent labels, particularly for cellular morphological imaging.
Acknowledgements
This study was supported by a grant of the Korea Health 21 R&D Project, Ministry of Health & Welfare, Republic of Korea (A060660) and the Korea Research Foundation Grant funded by the Korean Government (MOEHRD) (KRF-2005-005-J13102).
References
- Alivisatos , AP , Gu , W and Larabell , C . 2005 . Quantum dot as cellular probes . Annu. Rev. Biomed. Eng. , 7 : 55 – 76 .
- Medintz , IL , Uyeda , HT , Goldman , E and Mattoussi , H . 2005 . Quantum dot bioconjugates for imaging labelling and sensing . Nat. Mater. , 4 : 435 – 446 .
- Nie , S , Kim , GJ and Simons , JW . 2007 . Nanotechnology applications in cancer . Annu. Rev. Biomed. Eng. , 9 : 257 – 288 .
- Dubertret , B , Skourides , P , Norris , DJ , Noireaux , V , Brivanlou , AH and Libchaber , A . 2002 . In vivo imaging of quantum dots encapsulated in phospholipid micelles . Science , 298 : 1759 – 1762 .
- Voura , EB , Jaiswal , JK , Mattoussi , H and Simon , SM . 2004 . Tracking metastatic tumor cell extravasation with quantum dot nanocrystals and fluorescence emission-scanning microscopy . Nat. Med. , 10 : 993 – 998 .
- Kim , S , Lim , YT , Soltesz , EG , DeGrand , AM , Lee , J , Nakayama , A , Parker , JA , Mihaljevic , T , Laurence , RG , Dor , DM , Cohn , LH , Bawendi , MG and Frangion , JV . 2004 . Near-infrared fluorescent type II quantum dots for sentinel lymp node mapping . Nat. Biotech. , 22 : 93 – 97 .
- Fan , H , Level , EW , Scullin , C , Gabaldon , J , Tallant , D , Bunge , S , Boyle , T , Wilson , MC and Brinker , CJ . 2005 . Surfactant-assisted synthesis of water-soluble and biocompatible semiconductor Quantum dot micelles . Nano Lett. , 5 : 645 – 648 .
- Gopalakrishnan , G , Danelon , C , Izewska , P , Prummer , M , Bolinger , P , Geissbühler , I , Demurtas , D , Dubochet , J and Vogel , H . 2006 . Multifunctional lipid/quantum dot hybrid nanocontainers for controlled targeting of live cells . Angew. Chem. Int. Ed. , 45 : 5478 – 5483 .
- Connor , EE , Mwamuka , J , Gole , A , Murphy , CJ and Wyatt , MD . 2005 . Gold nanoparticles are taken up by human cells but do not cause acute cytotoxicity . Small , 1 : 325 – 327 .
- Takahashi , H , Niidome , Y , Niidome , T , Kaneko , K , Kawasaki , H and Yamada , S . 2006 . Modification of gold nanorods using phosphatidylcholine to reduce cytotoxicity . Langmuir , 22 : 2 – 5 .
- Qu , LH and Peng , XG . 2002 . Control of photoluminescence properties of CdSe nanocrystals in growth . J. Am. Chem. Soc. , 124 : 2049 – 2055 .
- Maa , Y and Hsu , CC . 1999 . Performance of sonication and microfluidization for liquid–liquid emulsification . Pharm. Dev. Technol. , 4 : 233 – 240 .
- Yokoyamaa , M , Satoha , A , Sakuraia , Y , Okano , T , Matsumurab , Y , Kakizoeb , T and Kataokac , K . 1998 . Incorporation of water-insoluble anticancer drug into polymeric micelles and control of their particle size . J. Control. Release , 55 : 219 – 229 .
- Farhood , H , Serbina , N and Huang , L . 1995 . The role of dioleoyl phosphatidylethanolamine in cationic liposome mediated gene transfer . Biochim. Biophys. Acta , 1235 : 289 – 295 .
- Chen , Q , Zhang , L , Stass , SA and Mixson , AJ . 2000 . Co-polymer of histidine and lysine markedly enhances transfection efficiency of liposomes . Gene. Ther. , 7 : 1698 – 1705 .
- Duan , H and Nie , S . 2007 . Cell-penetrating quantum dots based on multivalent and endosome-disrupting surface coatings . J. Am. Chem. Soc. , 129 : 3333 – 3338 .