Abstract
A simple strategy for the modification of disposable combination graphite-clay electrode, pencil graphite electrode (PGE), by multi-walled carbon nanotube–gold nanoparticle (MWNT–GNP) composite multilayers was proposed as a sensor to determine estradiol. The modification was carried out by the layer-by-layer (LBL) assembling of polyethyleneimine (PEI), decorated GNPs and polyacrylic acid (PAA) functionalised MWNTs via electrostatic interactions on a negatively charged PGE. The resulting MWNT–GNP multilayer modified PGE (MWNT–GNP/PGE) exhibited a sensitive electrochemical response towards the oxidation of estradiol at 0.90 V. Under optimal working conditions, the oxidation current of estradiol at MWNT–GNP/PGE increased linearly with its concentration in the range 7.0 × 10−8–4.2 × 10−5 mol l−1, with a low-detection limit of 1.0 × 10−8 mol l−1 for 200 s accumulation at an open circuit. This sensor was successfully applied to the detection of estradiol in blood serums.
1. Introduction
Self-assembled multilayers are recently attracting increasing attention because of its properties resembling biomembranes in configuration and the promising applications in the field of interfacial chemistry. When incorporated in the sensing element of electrochemical sensors, self-assembled multilayers are usually able to endow electrochemical sensors with special functions, for example the enhancement of selectivity and sensitivity, the decrease of response time, the overpotential reduction of analytes and the improvement of stability. A variety of species are detected by self-assembled multilayer sensing films on different substrate electrodes, for instance, gold Citation1–5, pyrolytic graphite Citation6–8, glassy carbon Citation9–11, platinum (Pt) Citation12,Citation13, indium tin oxide (ITO) Citation14 and mercury electrodes Citation15,Citation16. Most of these electrodes need pretreatments before assembling processes to create functional groups as adsorption sites.
Estradiol (), a crucial female sex hormone, plays a very important role in female fertility. The change in its concentration is closely related to human health. Therefore, the determination of estradiol level is important for clinical diagnosis. Various techniques have been employed for the determination of estradiol, such as competitive enzyme-linked immunosorbent assay Citation17, high-performance liquid chromatography (HPLC) Citation18, liquid chromatography–mass spectrometry (LC–MS) Citation19 and gas chromatography-mass spectrometry (GC–MS) Citation20. Although these methods are sensitive, they are generally expensive and time consuming. As a simple and rapid technique, electrochemical methods are also used for the determination of estradiol, and chemically modified electrodes have been reported Citation21–29.
Thanks to its low cost, strong adsorption, easy maintenance, low-background current and wide potential window, pencil lead graphite has been demonstrated to be an excellent electrode material for the sensitive determination of various analytes, i.e. DNA Citation30–32, trace metals Citation33–35, paracetamol Citation36, caffeine Citation37, titratable acidity Citation38, nitrate Citation39,Citation40, pollutants Citation41 and immunoassay Citation42.
In this work we report a novel sensor based on self-assembled multilayer modified pencil graphite electrode (PGE) for highly sensitive determination of estradiol. The modification was carried out by the layer-by-layer (LBL) assembling of polyacrylic acid (PAA) decorated gold nanoparticles (GNPs) and polyethyleneimine (PEI) functionalised multi-walled carbon nanotubes (MWNTs) on the negatively charged surface of PGE via electrostatic interactions. The resulting MWNT–GNP composite modified electrode showed a sensitive electrochemical response towards the oxidation of estradiol. And the oxidation current was amplified for about 15 times compared to that at a bare PGE. This electrochemical sensor based on MWNT–GNP/PGE was successfully applied to the determination of estradiol in blood serum samples. This work demonstrated for the first time the promising application of PGE as substrate electrodes for constructing high-performance disposable electrochemical sensors by LBL techniques.
2. Experimental procedure
2.1. Chemicals
Standard solutions of estradiol (1.0 × 10−2 mol l−1) were prepared by dissolving estradiol (Sigma, USA) in absolute ethanol. PEI (MW = 10,000, 20%) and PAA (MW = 100,000) were purchased from Acros, USA. MWNTs (95%, Nanotimes Co., Chengdu, China) were of about 8 nm in diameter and were synthesised by a catalytic pyrolysis method and purified with concentrated HNO3. Phosphate buffer solutions (PBSs, 0.1 mol l−1) of desired pH were prepared from NaH2PO4 and Na2HPO4 and used as the supporting electrolyte. All the chemicals were of analytical grade and used without further purification. The water used in this study was doubly distilled.
Pencil leads (type 507, black lead of degree 2B) were purchased from a local store. All the pencil leads had a total length of 11.5 cm and a diameter of 0.5 mm.
2.2. Apparatus
Electrochemical measurements were performed using a CHI 830 electrochemical analyser (Chenhua Co., Shanghai, China). A conventional three-electrode system, including a MWNT/GNP self-assembled multilayer modified PGE working electrode, a saturated calomel reference electrode (SCE) and a Pt wire counter electrode, was employed. All experiments were conducted at room temperature. Scanning electron microscopy (SEM) was performed on Quanta 200, FEI, Holland.
2.3. Synthesis of PEI-decorated GNPs and PAA-functionalised MWNTs
PEI-grafted GNPs were prepared by adding in turn 0.23 ml 20% (w/w) PEI, 0.50 ml 1% (w/w) HAuCl4 and 2.00 ml 2.0 mg ml−1 KBH4 into 45.0 ml double distilled water, and adjusted the solution acidity to pH 7.0 with 1.0 mol l−1 HCl after 30 min. The average size of Au nanoparticles is about 9 nm (). PAA functionalised MWNTs were prepared by adding 50.0 mg MWNT into 200.0 ml 5.0 mg ml−1 PAA aqueous solution and sonicated the mixture for about 4 h. The resulting solution was filtered on a mixed cellulose filtering membrane and the collected MWNTs were thoroughly washed with water until the filtrate became neutral, which were redispersed in water with sonication to form a solution of 0.5 mg ml−1 ().
2.4. Fabrication of MWNT/GNP self-assembled multilayer-modified PGE
PEI is a synthetic polymer that is very soluble in water with a high capacity for positive charge Citation43–45, so PEI grafted GNPs is positive charged. After purifying with concentrated HNO3, there are many carboxyl groups and hydroxyl groups on the surface of MWNT. PAA is a polymeric substance containing carboxylic groups and linear CH2–CH2 chains, so PAA-functionalised MWNT is negatively charged. There are electrostatic interaction between PEI-grafted GNPs and PAA-functionalised MWNT, and electrostatic self-assembled film was formed.
The procedure for preparing MWNT/GNP self-assembled multilayer modified PGE is represented in . Briefly, pencil leads of 2.3 cm in length were adhered to copper wires with silver epoxy glue and dried in air for about 24 h. The pencil lead–copper wire joint was inserted into a plastic pipette tip with a portion of 5 cm copper wire exposed outside the plastic tube, and then sealed with insulated epoxy glues. The exposed pencil leads were cut to a length of 0.4 cm before use. PGEs were pretreated by sweeping in 0.1 mol l−1 NaOH in the potential range of 0.1 ∼ −1.0 V until stable cyclic voltammograms were obtained. This was performed to gain a reproducible surface of PGE instead of producing functional groups for the assembling process because the as-prepared PGE can also be modified by the MWNT–GNP composite via LBL techniques. To modify the surface of PGE with the multilayer of MWNTs and GNPs, a pretreated PGE was alternately soaked into the freshly prepared solution of PEI/GNP and PAA/MWNT for approximately 20 min at room temperature. During the intervals, the modified electrode was thoroughly rinsed with water to remove any physically adsorbed component. This was repeated for certain cycles to construct the desired MWNT–GNP/PGE. The photos and the structural mode of MWNT–GNP/PGE are shown in .
2.5. Electrochemical measurement
A volume of 10 ml phosphate buffer (0.1 mol l−1, pH 2.0) was used as the supporting electrolyte for the electrochemical detection of estradiol. In a typical measurement, MWNT–GNP/PGE underwent successive cyclic sweeps between 0.10 and 1.0 V until stable voltammograms were obtained. Then, a certain volume of estradiol solution in ethanol was added into the supporting electrolyte. After an accumulation at an open circuit for 200 s, linear sweep voltammograms in the same potential range were recorded, and the peak current at 0.876 V was measured. After each measurement, MWNT–GNP/PGE was activated by 15 successive cyclic voltammetric sweeps from +0.10 to +1.0 V at 100 mV s−1 in the supporting electrolyte to remove any adsorbates.
3. Results and discussion
3.1. Surface morphology of MWNT–GNP/PGE
The surface morphology of PGE and MWNT–GNP/PGE is characterised by SEM (). As shown in , although PGE seems to have a rough surface from the low-amplification appearance, its surface is actually smooth under high amplification. In contrast, after the modification by MWNT/GNP self-assembled multilayers, the surface of PGE becomes rather rough, along with the appearance of some particle-like microstructures (). This directly indicates the successful assembling of MWNT–GNP composite on the surface of PGE. The presence of particle-like rather than thread-like modifiers on the surface of PGE may arise from the serious intertwist of PEI-functionalised MWNTs and PAA-decorated GNPs due to strong interactions by molecular assembling.
3.2. Electrochemical behaviours of estradiol sensor
The electrochemical behaviour of estradiol sensor based on MWNT–GNP/PGE in 0.1 mol l−1 phosphate buffer (pH 2.0) was investigated by cyclic voltammetry (CV) (not shown). An oxidation peak is observed on cyclic voltammograms at 0.88 V, and no reduction peak appears in the reversal scan, indicating that the electrochemical oxidation of estradiol is an irreversible process. In addition, the oxidation peak current of estradiol decreases with the increase in number of the sweeping cycles. This may be explained with strong adsorption of the reaction products, which may passivate the electroactive sites on the surface of the electrode and block the diffusion of estradiol from solution to the electrode surfaces.
The electrochemical behaviours of estradiol at different PGEs are studied by linear sweep voltammetry (LSV) (). It is clear that no apparent electrochemical response of estradiol is observed on PGE (curve (a)). Whereas a small oxidation peak at 0.90 V appears after 200 s accumulation in an open circuit (curve (b)), indicating that accumulation operations can effectively improve the electrochemical response of estradiol. It is also observed that the peak current of estradiol at MWNT/PGE (e) and GNP/PGE (f) have evident enhancement compared to curve (b), which have testified the strong adsorption ability of MWNT and the excellent electric ability of GNP. Furthermore, estradiol shows a sensitive oxidation peak by no accumulation at MWNT–GNP/PGE (curve (c)), which is significantly enhanced after an accumulation at an open circuit for 200 s (curve (d)). Moreover, the background current of MWNT–GNP/PGE is enlarged for about 15 times compared with PGE. Thus, the enhanced sensitivity of estradiol at MWNT–GNP/PGE should mainly arise from the high-surface area of the MWNT–GNP composite film on PGE as well as the strong accumulation capacity of this film towards estradiol.
Figure 4. LSVs of 1.0 × 10−5 mol l−1 estradiol in 0.1 mol l−1 phosphate buffer (pH 2.0) on bare PGE (b), MWNT–PGE (e), GNP/PGE (f) and MWNT–GNP/PGE (d). Accumulation time = 200 s, scan rate = 100 mV s−1. Curves (a) and (c) correspond to bare PGE and MWNT–GNP/PGE in the same condition without accumulation, respectively.
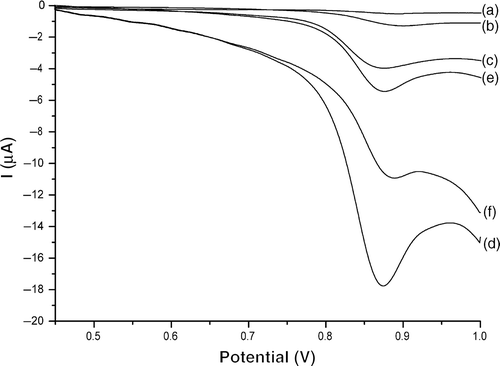
3.3. Electrooxidation mechanism of estradiol at MWNT–GNP/PGE
The influence of scan rate on the peak current of 1.0 × 10−5 mol l−1 estradiol was examined by LSV (). The results showed that the oxidation peak current of estradiol at MWNT–GNP/PGE increases linearly with the square root of scan rate from 50 to 300 mV s−1 (insets (a)), suggesting that the electrochemical oxidation of estradiol under this condition is controlled by a diffusion process. As a totally irreversible electrochemical process, the relationship between the oxidation peak potential of estradiol and scan rate can be described as follows Citation46:
Figure 5. LSVs of 1.0 × 10−5 mol l−1 estradiol at MWNT–GNP/PGE in 0.1 mol l−1 phosphate buffer (pH 2.0) at scan rate from 50 to 300 mV s−1. Inset: (a) Plot of peak current versus the square root of scan rate; (b) plot of peak potential versus the logarithm of scan rate.
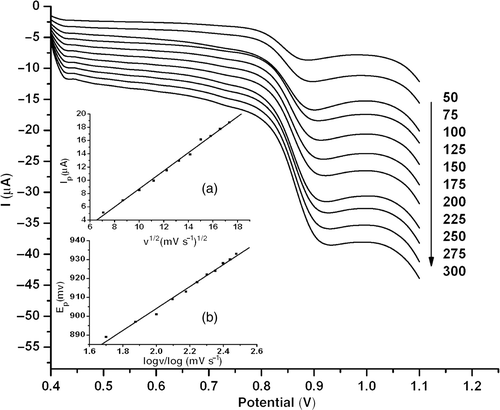
According to Equations (Equation1) and (Equation3), we can calculate αan = 0.509. This value is consistent with the result deduced from Equation (Equation2) (i.e. αan = 0.501) when the average value of Ep − Ep /2 is 95.20 mV in the scan rate range of 50–300 mV s−1. In the case of a totally irreversible process, α can be regarded as 0.5. Therefore, one electron might be involved in the rate-determining step of estradiol oxidation. That is to say, the reaction site for the oxidation of estradiol at MWNT–GNP/PGE may be the hydroxyl group in A-ring.
The variation of the electrochemical response of estradiol with solution pH in 0.1 mol l−1 phosphate buffer was studied using LSV. shows the oxidation peak current of 1.0 × 10−5 mol l−1 estradiol in solution of different pHs. The results showed that the peak potential was pH-dependent and gradually shifted to the positive direction by increasing solution acidity from pH 7.0 to 1.0, which obeys the following equation:
Figure 6. LSVs of 1.0 × 10−5 mol l−1 estradiol at MWNT–GNP/PGE at different solution pHs. Inset: (a) Plot of peak potential versus pH; (b) plot of peak current versus pH.
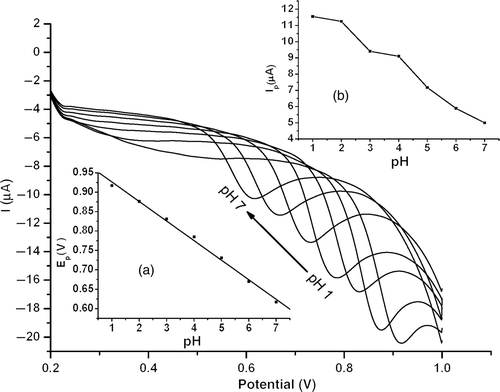
The slope of plot Ep versus pH is about 0.050, suggesting that the number of electrons transferred was equal to that of protons.
3.4. Optimisation of working conditions
3.4.1. Supporting electrolyte
The conditions of the electrolyte, such as types of electrolyte and solution pH, are major factors that affect the response of estradiol sensor. A series of supporting electrolytes were tested for the determination of estradiol, including acetate, citrate and phosphate buffers. Both the peak height and the peak shape were taken into consideration when choosing the supporting electrolyte. Of these, phosphate buffer gave the best response. Furthermore, phosphate buffers of different concentrations ranged from 0.01 to 0.2 mol l−1 were tested at a constant estradiol concentration. The result showed that the highest signal could be obtained in 0.1 mol l−1 phosphate buffer. This indicated that 0.1 mol l−1 phosphate buffer (pH 2.0) was suitable as the supporting electrolyte.
3.4.2. Assembling cycle for preparing MWNT–GNP/PGE by LBL techniques
The effect of assembling cycle on the peak current of estradiol was examined (). It showed that at the beginning, the peak current increased with the increase in assembling cycle, due to the loading increase in MWNTs and GNPs on PGE as well as the enlargement of the effective sensing area by these functional nanomaterials. However, when the assembling process was repeated for more than seven cycles, the peak current decreased slightly by contraries. This could be explained that a too thick MWNT–GNP composite was formed which blocked the mass transfer and electron transfer of estradiol. Therefore, seven cycles were selected as the optimal condition for preparing MWNT–GNP/PGE by LBL techniques.
3.4.3. Accumulation conditions
The accumulation potential is also an additional key factor, which affects the sensitivity of estradiol sensor. The influence of accumulation potential on the peak current of 1.0 × 10−5 mol l−1 estradiol was evaluated. shows that the peak current hardly changed when the adsorption potential varies in the range 0.1–0.6 V, indicating a possible hydrophobic adsorption mechanism of estradiol on MWNT–GNP/PGE. Thus, an optimal accumulation condition at an open circuit was performed to avoid any interference by potential bias.
Figure 8. LSVs of 1.0 × 10−5 mol l−1 estradiol at MWNT–GNP/PGE in 0.1 mol l−1 phosphate buffer (pH 2.0) at an accumulation potential from 0.1 to 0.6 V. Inset: Plot of peak current versus accumulation potential.
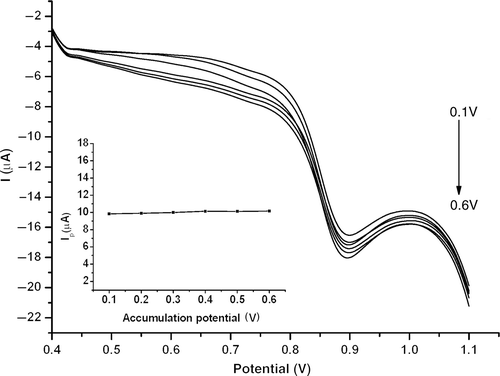
shows the effect of accumulation time on the peak current of 1.0 × 10−5 mol l−1 estradiol. The oxidation peak current of estradiol showed an obvious dependence on accumulation time, which was proportional to the accumulation time within the range 0–200 s. Beyond 200 s, the oxidation current increased slightly and tended to stability. Thus, an accumulation time of 200 s was used throughout.
3.5. Analytical performance
Under the optimised conditions, the relationship between the oxidation peak current and the concentration of estradiol was examined (). The peak current (Ip ) was proportional to estradiol concentration in the range 7.0 × 10−8–4.2 × 10−5 mol l−1, which could be described as I (µA) = 2.88472 + 7.56656c (µmol l−1) (R = 0.994). At concentrations higher than 8.6 × 10−5 mol l−1, the oxidation peak current of estradiol increased slowly. For 200 s accumulation in an open circuit, a low-detection limit of 1.0 × 10−8 mol l−1 was obtained (S/N = 3).
Figure 10. LSVs of 1.0 × 10−5 mol l−1 estradiol at MWNT–GNP/PGE in 0.1 mol l−1 phosphate buffer (pH 2.0) at different bulk concentrations (from a to m: 0.40, 0.90, 1.20, 1.50, 4.20, 7.00, 9.80, 12.5, 18.0, 23.3, 28.6, 33.9 and 41.6 μmol l−1). Inset: Plot of peak current versus concentrations: (a) from 7.0 × 10−8 to 1.5 × 10−6 mol l−1; (b) from 1.5 × 10−6 to 4.2 × 10−5 mol l−1.
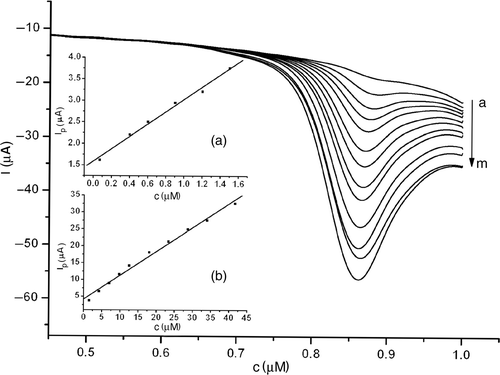
The relative standard deviation (RSD) for eight parallel measurements of 1.0 × 10−5 mol l−1 estradiol at the same sensor was 3.9% and that obtained at eight different modified electrodes was 7.3%. This result suggests that MWNT–GNP/PGE sensor has a good reproducibility and repeatability for the determination of estradiol.
3.6. Interferences
The influences of some potential interferents on the determination of estradiol were investigated (). The results showed that 1.0 × 10−4 mol l−1 ascorbic acid, dopamine, Vitamin B, barbital, caffeine, o-nitrophenol, L-arginine, L-cysteine, cholesterol, xanthine and hypoxanthine hardly interfered with the determination of 1.0 × 10−5 mol l−1 estradiol (i.e. signal change <5%). At the same time, although MWNT–GNP/PGE exhibited sensitive oxidation responses towards uric acid and o-aminophenol, the oxidation peak potentials of these species (1.0 × 10−4 mol l−1) (i.e. 0.62 and 0.52 V for uric acid and o-aminophenol, respectively) were well-separated from that of estradiol, and no interferences were observed for the determination of 1.0 × 10−5 mol l−1 estradiol at 0.88 V. The interference of estrone was serious due to the similar structural and electrochemical properties of estrone to estradiol.
Table 1. Interferences from some organic compounds on the determination of 1.0 × 10−5 mol l−1 estradiol. Accumulation condition: 200 s in an open circuit; scan rate: 100 mV s−1.
3.7. Real sample analysis
This estradiol sensor exhibited high sensitivity and good selectivity for the determination of estradiol under an optimal laboratory condition. To illustrate its practical applicability, the sensor has been utilised to detect estradiol in several blood serums of pregnant women. It is known that the concentration levels of free estradiol, estrone and estriol in serum of human patients are about 200–600, 200–600 and 100–300 pg ml−1, respectively Citation47. We use the standard addition method to determine the estradiol content. The experimental procedure involved the direct addition of 1.0 ml blood serum to electrochemical cells containing 4.0 ml PBS (pH 2.0) and the consequent voltammetric measurement. The good recoveries demonstrate the promising application of this sensor for the determination of estradiol in real samples. shows the results for the determination of estradiol in blood serums.
Table 2. Determination of estradiol in blood serums. Accumulation condition: 200 s in an open circuit; scan rate: 100 mV s−1.
4. Conclusions
As a kind of combination of materials consisting of graphite and clay, pencil leads are negatively charged under general conditions due to the presence of clay, which provide a large number of derivation sites for the surface modification by electrostatic assembling. This, along with the successful employments of pencil leads as electrode materials, foresees the wide application of surface-functionalised PGE. In this work, a simple route for the fabrication and the surface modification of PGE was proposed. The modification was based on the alternative assembling of PEI-decorated GNP and PAA-functionalised MWNTs on PGE by LBL techniques. The resulting MWNT–GNP multilayer composite modified PGE exhibited a sensitive electrochemical response towards the oxidation of estradiol. After the optimisation of some working conditions, e.g. scan rate, supporting electrolyte, solution pH, assembling cycle and accumulation condition, a wide calibration range of 7.0 × 10−8– 4.2 × 10−5 mol l−1 and a low-detection limit of 1.0 × 10−8 mol l−1 for the determination of estradiol were obtained. This sensor was successfully applied to the determination of estradiol in serum samples. The result suggested that this method had good reproducibility and reliability for the determination of estradiol in real samples.
Acknowledgements
This research is supported by the National Nature Science Foundation of China (No. 20805035).
References
- Shervedani , R , Hatefi-Mehrjardi , A and Asadi-Farsani , A . 2007 . Sensitive determination of iron (III) by gold electrode modified with 2-mercaptosuccinic acid self-assembled monolayer . Anal. Chim. Acta , 601 : 164 – 171 .
- Sun , Y and Wang , S . 2006 . Simultaneous determination of dopamine and ascorbic acid at a triazole self-assembled monolayer-modified gold electrode . Microchim. Acta , 154 : 115 – 121 .
- Sehlotho , N and Nyokong , T . 2006 . Electrocatalytic oxidation of thiocyanate, l-cysteine and 2-mercaptoethanol by self-assembled monolayer of cobalt tetraethoxy thiophene phthalocyanine . Electrochim. Acta , 51 : 4463 – 4470 .
- Bozic , R , West , A and Levicky , R . 2008 . Square wave voltammetric detection of 2,4,6-trinitrotoluene and 2,4-dinitrotoluene on a gold electrode modified with self-assembled monolayers . Sensors Actuators B , 133 : 509 – 515 .
- Loyprasert , S , Thavarungkul , P , Punnee , A , Booncharoen , W , Chusak , L and Proespichaya , K . 2008 . Label-free capacitive immunosensor for microcystin-LR using self-assembled thiourea monolayer incorporated with Ag nanoparticles on gold electrode . Biosensors Bioelectron. , 24 : 78 – 86 .
- Brett , A and Chiorcea , A . 2003 . Atomic force microscopy of DNA immobilized onto a highly oriented pyrolytic graphite electrode surface . Langmuir , 19 : 3830 – 3839 .
- He , P , Hu , N and Zhou , G . 2002 . Assembly of electroactive layer-by-layer films of hemoglobin and polycationic poly (diallyldimethylammonium) pyrolytic graphite electrode . Biomacromolecules , 3 : 139 – 146 .
- Chen , X , Ruan , C , Kong , J and Deng , J . 2000 . Characterization of the direct electron transfer and bioelectrocatalysis of horseradish peroxidase in DNA film at pyrolytic graphite electrode . Anal. Chim. Acta , 412 : 89 – 98 .
- Yu , Z , Tian , M , Jiao , Q and Xia , D . 2006 . Self-assembling nanobimetallic particles of Au-Pt on glassy carbon electrode . Acta Phys. – Chim. Sin. , 22 : 1015 – 1020 .
- Wu , F , Hu , Z , Xu , J , Tian , Y , Wang , L , Xian , Y and Jin , L . 2008 . Immobilization of horseradish peroxidase on self-assembled (3-mercaptopropyl)trimethoxysilane film: Characterization, direct electrochemistry, redox thermodynamics and biosensing . Electrochim. Acta , 53 : 8238 – 8244 .
- Alexeyeva , N and Tammeveski , K . 2008 . Electroreduction of oxygen on gold nanoparticle/PDDA-MWCNT nanocomposites in acid solution . Anal. Chim. Acta , 618 : 140 – 146 .
- Marx , K and Zhou , T . 2002 . Comparative study of electropolymerization versus adsorption of tyrosine and the decyl ester of tyrosine on platinum electrodes . J. Electroanal. Chem. , 521 : 53 – 60 .
- Ma , Y , Wang , J , Hui , F and Zang , S . 2006 . The reassembled behavior of bilayer lipid membranes supported by Pt electrode . J. Memb. Sci. , 286 : 174 – 179 .
- Jing , A , Tan , Y , Wang , Y , Ding , S and Qian , W . 2007 . Interfacial construction of gold nanoshells on 3-aminopropyl triethoxysilane modified ITO elecrtrodesurface for studying cytochrome b562 electrochemistry . J. Nanosci. Nanotechnol. , 7 : 440 – 446 .
- Buoninsegni , F , Dolfi , A and Guidelli , R . 2004 . Two photobioelectrochemical applications of self-assembled films on mercury . Collect. Czech. Chem. Commun. , 69 : 292 – 308 .
- Madueno , R , Pineda , T , Sevilla , J and Blazquez , M . 2004 . An electrochemical study of 6-thioguanine monolayers on a mercury electrode in acid and neutral solutions . J. Electroanal. Chem. , 565 : 301 – 310 .
- Volpe , G , Fares , G , delli Quadri , F , Draisci , R , Ferretti , G , Marchiafava , C , Moscone , D and Palleschi , G . 2006 . A disposable immunosensor for detection of 17β-estradiol in non-extracted bovine serum . Anal. Chim. Acta , 572 : 11 – 16 .
- Yamada , H , Yoshizawa , K and Hayase , T . 2002 . Sensitive determination method of estradiol in plasma using high-performance liquid chromatography with electrochemical detection . J. Chromatogr. B , 775 : 209 – 213 .
- Watabe , Y , Kubo , T , Nishikawa , T , Fujita , T , Kaya , K and Hosoya , K . 2006 . Fully automated liquid chromatography–mass spectrometry determination of 17ß-estradiol in river water . J. Chromatogr. A , 1120 : 252 – 259 .
- Edman , K , Eriksson , B , Gunnarsson , P and Svensson , L . 2000 . Determination of estramustine phosphate and its metabolites estromustine, estramustine, estrone and estradiol in human plasma by liquid chromatography with fluorescence detection and gas chromatography with nitrogen-phosphorus and mass spectrometric detection . J. Chromatogr. B , 738 : 267 – 279 .
- Lin , X and Li , Y . 2006 . A sensitive determination of estrogens with a Pt nano-clusters/multi-walled carbon nanotubes modified glassy carbon electrode . Biosensors Bioelectron. , 22 : 253 – 259 .
- Song , J , Yang , J and Hu , X . 2008 . Electrochemical determination of estradiol using a poly(L-serine) film-modified electrode . J. Appl. Electrochem. , 38 : 833 – 836 .
- He , Q , Yuan , S , Chen , C and Hu , S . 2003 . Electrochemical properties of estradiol at glassy carbon electrode modified with nano-Al2O3 film . Mater. Sci. Eng. C , 23 : 621 – 625 .
- Hu , S , Wu , K , Yi , H and Cui , D . 2002 . Voltammetric behavior and determination of estrogens at Nafion-modified glassy carbon electrode in the presence of cetyltrimethylammonium bromide . Anal. Chim. Acta , 464 : 209 – 216 .
- Murugananthan , M , Yoshihara , S , Rakuma , T , Uehara , N and Shirakashi , T . 2007 . Electrochemical degradation of 17β-estradiol (E2) at boron-doped diamond (Si/BDD) thin film electrode . Electrochim. Acta , 52 : 3242 – 3249 .
- Jin , G and Lin , X . 2005 . Voltammetric behavior and determination of estrogens at carbamylcholine modified paraffin-impregnated graphite electrode . Electrochim. Acta , 50 : 3556 – 3562 .
- Sun , Y , Wu , K and Hu , S . 2003 . Fabrication of a multi-wall carbon nanotubes modified glassy carbon electrode and its catalytic effect on the oxidation of estradiol, estrone and estriol . Microchim. Acta , 142 : 49 – 53 .
- Liu , X and Wong , D . 2007 . Electrocatalytic detection of estradiol at a carbon nanotube|Ni(Cyclam) composite electrode fabricated based on a two-factorial design . Anal. Chim. Acta , 594 : 184 – 191 .
- Hu , C , Yang , C and Hu , S . 2007 . Hydrophobic adsorption of surfactants on water-soluble carbon nanotubes: A simple approach to improve sensitivity and antifouling capacity of carbon nanotubes-based electrochemical sensors . Electrochem. Commun. , 9 : 128 – 134 .
- Kara , P , Erdem , A , Girousi , S and Ozsoz , M . 2005 . Electrochemical detection of enzyme labeled DNA based on disposable pencil graphite electrode . J. Pharma. Biomed. Anal. , 38 : 191 – 195 .
- Ozcan , A , Sahin , Y , Ozsoz , M and Turan , S . 2007 . Electrochemical oxidation of ds-DNA on polypyrrole nanofiber modified pencil graphite electrode . Electroanalysis , 19 : 2208 – 2216 .
- Hejazi , M , Alipour , E and Pournaghi-Azar , M . 2007 . Immobilization and voltammetric detection of human interleukine-2 gene on the pencil graphite electrode . Talanta , 71 : 1734 – 1740 .
- Rehacek , V , Hotovy , I , Vojs , M and Mika , F . 2008 . Bismuth film electrodes for heavy metals determination . Microsyst. Technol. , 14 : 491 – 498 .
- Bond , A , Mahon , P , Schiewe , J and Vicente-Beckett , V . 1997 . An inexpensive and renewable pencil electrode for use in field-based stripping voltammetry . Anal. Chim. Acta , 345 : 67 – 74 .
- Blum , D , Leyffer , W and Holze , R . 1996 . Pencil-leads as new electrodes for abrasive stripping voltammetry . Electroanalysis , 8 : 296 – 297 .
- Ozcan , L and Sahin , Y . 2007 . Determination of paracetamol based on electropolymerized molecularly . Sensors Actuators, B , 127 : 362 – 369 .
- Ly , S , Jung , Y , Kim , M , Han , I , Jung , W and Kim , H . 2004 . Determination of caffeine using a simple graphite pencil electrode with square-wave anodic stripping voltammetry . Microchim. Acta , 146 : 207 – 213 .
- Kotani , A , Miyaguchi , Y , Harada , D and Kusu , F . 2003 . A disposable voltammetric cell for determining the titratable acidity in vinegar . Anal. Sci. , 19 : 1473 – 1476 .
- Bendikov , T and Harmon , T . 2005 . A sensitive nitrate ion-selective electrode from a pencil lead. An analytical laboratory experiment . J. Chem. Edu. , 8 : 439 – 411 .
- Kitajima , A , Teranishi , T and Miyake , M . 2001 . Detection of nitric oxide on carbon electrode modified with ionic polymers and α-cyclodextrin . Electrochemistry , 69 : 16 – 20 .
- Ozsoz , M , Erdem , A , Ozkan , D , Kerman , K and Pinnavaia , T . 2003 . Clay/sol−gel-modified electrodes for the selective electrochemical monitoring of 2,4-dichlorophenol . Langmuir , 19 : 4728 – 4732 .
- Engel , L , Baumann , W and Fresenius , J . 1993 . Direct potentiometric immunoelectrodes *III. A graphite based atrazine immunoelectrode . Anal. Chem. , 346 : 745 – 751 .
- Chirila , T , Rakoczy , P , Garrett , K , Lou , X and Constable , I . 2002 . The use of synthetic polymers for delivery of therapeutic antisense oligodeoxynucleotides . Biomaterials , 23 : 321 – 342 .
- Panyam , J and Labhasetwar , V . 2003 . Biodegradable nanoparticles for drug and genedelivery to cells and tissue . Adv. Drug Deliv. Rev. , 55 : 329 – 347 .
- Kircheis , R , Wightman , L and Wagner , E . 2001 . Design and gene delivery activity of modified polyethylenimines . Adv. Drug Deliv. Rev. , 53 : 341 – 358 .
- Bard , A and Faulkner , L . 1980 . Electrochemical Methods Fundamentals and Applications , New York : Wiley .
- Katayama , M , Matsuda , Y , Nakane , R , Hara , I , Sato , H and Kaneko , S . 1998 . Determination of estrogens by high performance liquid chromatography with pre-label derivatization with 2-(4-carboxy)-5,6-dimethylbenzimidazole (II): Shortening of analysis time and application to monitoring estrogen in serum from in-vitro fertilization embryo transfer (IVF-ET) patients . Anal. Lett. , 31 : 2145 – 2158 .