1. Surface plasmon resonance (SPR) technology
A significant number of diverse and multidisciplinary research areas ranging from protein chemistry, enzyme kinetics to drug discovery require elucidation of macromolecular interactions such as protein–protein interaction, protein–ligand, and drug–receptor interactions. Currently adopted methodologies have inherent limitations to restrict evaluation at a molecular level. Surface Plasmon Resonance (SPR) technology is a powerful label-free method can overcome these limitations. Concisely, one of the two interacting moieties (ligand) is immobilized on a sensor chip surface, and the other (analyte) is passed through a microfluidic system in contact with the chip surface. The sensor chip is central to the SPR system, which provides the essential physical conditions necessary to emit the signal. The molecules under study interact on the surface of the chip. Biomolecules can be attached to the surface of the sensor chip by covalent immobilization, high affinity capture or hydrophobic adsorption [Citation1]. Principle of SPR operation is detailed in . Real time binding as a change of mass at the surface, is measured and the interaction can be characterized in terms of ‘on’ and ‘off’ rates (kinetics) and binding strength (affinity). Due to these high-throughput features, SPR remains as a technique of choice for rapid and quantitative in vitro evaluations to characterize, screen, and develop small molecules for biomedical applications and to evaluate protein interactions that drive protein signaling. SPR was first used in 1990s in biological system [Citation2]. It is a single solution for interaction analysis of molecular events in real time allowing quantitative measurement of; (a) concentrations of active analytes ranging from pM (picomoles) to mM (millimoles), (b) specificity of the binding between two molecules, (c) kinetics to generate information on, the rate of association and/or dissociation, cooperativity or allosteric effects, as well as scatchard analysis, (d) affinity between to binding partners, and (e) binding targets identification due to ability of SPR to interface with mass spectrometers which provides discovery-based research in proteomic studies. Currently, SPR technique is increasingly becoming a prime choice of researchers to expedite their drug discovery efforts [Citation3]. This in vitro technique is very well accepted to determine the binding between, protein–protein, Protein–DNA, enzyme–substrate or inhibitor, receptor–drug, protein–Lipid membrane (or polysaccharides) and cell or virus–protein [Citation3]. Specific applications of SPR in drug discovery and applications are concisely explained in [Citation4–Citation6].
Figure 1. Schematic illustration of SPR to determine binding interactions of representative small molecule ligand (molecule in the black circle) to target protein (blue): (a) Analytes (small molecule ligands; black circle) are passed through microfluidic system (buffer) and flown over the receptor (target protein; blue ribbon structure) immobilized on the chip surface. Polarized light is directed through a prism and incident upon bottom side of sensor chip (surface of the gold film) where waves of surface plasmons are generated at the critical angle of incident light; (b) After analytes bind to the receptor, there is a change in the critical angle of the incident light and reflected as I and II; (c) SPR sensogram comprising of the association phase (I) and the dissociation phase (II). SPR response values are expressed in resonance units (RU).
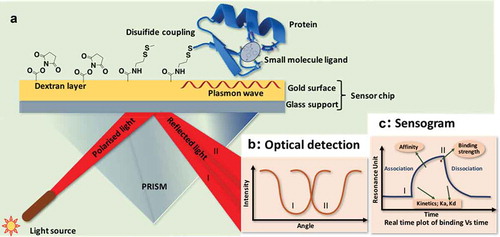
Table 1. Application of surface plasmon resonance (SPR), high-resolution Orbitap mass analyzer and Raman advancement in the area of drug discovery and delivery.
2. Orbitrap mass spec
The emergence of biopharmaceuticals in drug discovery, development, and biomarker discovery in the last decade was substantially supported by new advancements in biological mass spectrometry. The Orbitrap mass analyzer was introduced by Alexander Makarov in 1999 [Citation7], which is the most widely used analyzer in modern Orbitrap mass spectrometers. Orbitrap is a high-resolution, accurate mass (HR/AM) analyzer. One of the main advantages of HR/AM instrument for proteomic research is that they provide accurate charge state and mass of the multiply charged species in the complex mixtures. The level of protein identification, characterization and metabolite profiling confidence that it has been providing is invaluable for OMICS research. The major shortcomings of Fourier transform family mass spectrometers were overcome by Orbitrap, such as complexity and high maintenance of Fourier Transform-Ion Cyclotron Resonance (FT-ICR), low sensitivity and dynamic range of Time-of-Flight (TOF) and limited mass accuracy of ion trap. The typical hybrid Orbitrap mass spectrometer uses quadrupole for precursor ion selection and Orbitrap analyzer for high resolution and accurate mass. The currently available hybrid Orbitrap mass spectrometers have mass resolution ranging from 140,000 FWHM at m/z 400 to 1,000,000 in the full scan mode. Orbitrap mass analyzers have accelerated the study of biochemical metabolites and their relationship in complex mixtures [Citation8]. In the study of proteomics, top-down and bottom-up approaches are routinely used for obtaining high-quality sequence information. Orbitrap has been successfully used for both the approaches and facilitated the identification of challenging protein isoforms and post translational modifications (PTMs) indicating the high mass accuracy, resolving power, and performance [Citation9]. Another major leap in the use of Orbitrap is native mass spectrometry for the discovery of noncovalent protein complexes in their native form [Citation10]. Of the several advancements made in Orbitrap mass spectrometers since its invention, addition of alternative fragmentation techniques such as electron transfer dissociation has improved the possibilities of Orbitrap [Citation11]. Unlike FT-ICR and TOF, the ease of use and sensitivity of Orbitrap makes it viable for quantitative experiments. The combination of quadrupole isolation of precursor ions, transfer of ions via high-energy collision-induced dissociation (HCD) cell and C-trap defines the parallel reaction monitoring (PRM) capability of Orbitrap mass spectrometer. The PRM- based targeted protein quantification is highly selective and specific [Citation12]. Applications of Orbitrap mass spec are listed in .
3. Raman advancements
Raman spectroscopy measures vibrational modes produced by the interaction of the incidence photon and the drug molecules (stokes and anti-stokes scattering). It results in the unique fingerprinting and can be utilized in the early stages of compound identification, to their full spectrum characterization. Advantages of Raman includes quick and easy sample preparation, noninvasive nature, continuous in-line monitoring, wider spectral range 4000 to 50 cm−1 and analysis of aqueous drug samples. Downside such as high fluorescence underlying Raman bands and decomposition of thermal sensitive drugs due to high intensity of radiation have limited its utilization in drug discovery and development process. However, its increasing usage can be attributed to recent advancements i.e. 1) Advancement in Raman techniques such as compact, handheld, dispersive, confocal, Fourier etc. due to advancement in optics, electronics, lasers, detectors, temperature variable, and filters; 2) Advent of newer Raman spectroscopic techniques such as stimulating Raman scattering (SRS), transmission Raman spectroscopy (TRS), surface enhanced Raman scattering (SERS), tip enhanced Raman scattering (TERS), coherent anti-stokes Raman scattering (CARS) and 3) Conjunction of Raman with other analytical techniques such as Infrared and Near-infrared (NIR) spectroscopy, differential scanning calorimetry (DSC), dynamic vapor sorption (DVS), scanning electron microscopy (SEM), atomic force microscopy (AFM) and x-ray and neutron scattering, absorption and diffraction [Citation13–Citation15]. These advancements make Raman a unique and powerful technique for both qualitative and quantitative analysis of newly synthesized compounds and their characterization in both drug discovery and development stages with high accuracy and sensitivity. Along with several generalized applications of Raman listed in , recently it is successfully used in 3D spheroid culture for drug discovery studies, assessing the risk of drug crystallization in vivo, antibiotic discovery and understanding the molecular basis of resistance, understanding chemical processes and kinetics of drug actions within living cells, drug detection in microfluidic device, toxicological analysis of synthetic cannabinoids and herbal-based medicines.
Overall, all the three techniques, i.e., SPR, orbitrap mass spec and Raman; individually or in combination, have great potential in speeding the process of drug discovery by characterizing newly synthesized drug molecules with great sensitivity, specificity and accuracy. These techniques work with on different principles and the structural/physico-chemical-biological information generated from the techniques have significant potential to improve the early stage drug development process ().
4. Expert opinion
SPR: Rapid acceptance of SPR in the field of research and drug development makes it an excellent analytical tool for point-of-care diagnostics. The stability of the target protein and its extreme immobilization are pivotal factors for high precision screening that can be achieved optimizing immobilized proteins and re-generable sensors. Furthermore, roles of SPR in determining enzyme kinetics and detecting protein interactions with drug molecules, peptides, hormones, toxins, explosive residues, and bacterial pathogens are now well established. Interestingly, the use of SPR for fragment-based/high throughput screening drug–design has been evaluated with a rational dynamic flow of fragment molecules over target protein static on biosensor chip. However, the challenge for simultaneous detection of protein-interacting compounds whilst HTS lies in the development of sophisticated SPR integrated to mass spectroscopy (SPR-MS). Albeit huge numbers of SPR prototypes biosensors are already existing and many more are in development stage, the capability of biosensor chips with an integrated array of real time analyte binding kinetics and molecular characterization will have a predominant role for the next wave of drug discovery. This along with improving the already established (and commercially available) sensing device would greatly enhance new improvements in SPRs. Thus, trends in SPR technology regarding instrumentation, fluidics, sensor surfaces and kinetic analysis are speculative to degree of impact in the next few years.
Orbitrap: The study of membrane proteins was a challenging target due to its hydrophobic nature and interaction with lipid bilayer. However, the recent improvements in mass spectrometry instrumentation especially Orbitrap mass analyzer has led to effective analysis of intact proteins in their native form using top down proteomics approach. The more efficient fragmentation techniques viz. electron transfer/higher-energy collisional dissociation (EThcD), electron transfer dissociation (ETD) has greatly improved the fragmentation capabilities of mass spectrometer to identify post translational modifications (PTMs). The key strategy for improving the performance of mass spectrometers was to create hybrid instruments, meaning hyphenation of different mass analyzers to improve the capabilities of the mass spectrometer. An example of such hybrid instrument is Orbitrap Fusion Lumos Tribrid, which incorporated quadrupole, orbitrap, and linear ion trap. In addition to bringing together these different mass analyzers, incorporating multipole and C-trap made the instrument versatile. However, the mass spectrometer techniques used for some of the reported works on native mass spectrometry are prototypes, which are not yet available to widely to researchers. The prospects for the developments in mass spectrometry instrumentation and advanced hyphenation like combining imaging at molecular level with mass spectrometer would provide more detailed structure and regulation of proteins and their complexes. The wide scope of native mass spectrometry is yet to solve many complex biological questions inherent in structural and molecular biology. Overall, with all-in-one characteristics like mass accuracy, resolution and speed, Orbitrap could be explored as one stop solution for both quantitative and qualitative experiments.
Raman: Exciting new advancement in Raman, its utilization in conjunction with other analytical tools and varied applications makes Raman as one of the most powerful, yet underutilized tool in early drug discovery and delivery processes. Significant advancement in the commercial lasers, CCD-detectors and interference filters have helped overcome traditional low intensity disadvantage of weak scattering peaks of Raman. Also, the advancement of Raman to its handheld version (with built in compound libraries) allows quick, nondestructive, no sample preparation and on the spot identification/analysis of compounds. In future, the technique holds substantial potential in the areas of drug discovery, chemical sensing, biological imaging, forensics, mineral identification, material characterization etc.
Declaration of interest
The authors have no other relevant affiliations or financial involvement with any organization or entity with a financial interest in or financial conflict with the subject matter or materials discussed in the manuscript apart from those disclosed.
Reviewer disclosures
Peer reviewers on this manuscript have no relevant financial or other relationships to disclose.
Correction Statement
This article has been republished with minor changes. These changes do not impact the academic content of the article.
Additional information
Funding
References
- Mariani S, Minunni M. Surface plasmon resonance applications in clinical analysis. Anal Bioanal Chem. 2014 Apr;406(9–10):2303–2323.
- Karlsson R, Michaelsson A, Mattsson L. Kinetic analysis of monoclonal antibody-antigen interactions with a new biosensor based analytical system. J Immunol Methods. 1991 Dec 15;145(1–2):229–240.
- Meza-Sanchez DE, Maravillas-Montero JL. Clinical and biomedical applications of surface plasmon resonance systems. Rev Invest Clin. 2019;71(2):85–90.
- Application note: Hepatitis C drug target, NS5B 1b, in the search for novel allosteric drug leads, GE Healthcare Life Sciences, Application note 29245694 edition AA; 2017.
- Application note: Characterizing lead compounds using label-free interaction analysis: Blocking T-cell activation in rheumatoid arthritis therapy, Application Note 28-9383-78, edition AA; 2008.
- Antiochia R, Bollella P, Favero G, et al. Nanotechnology-based surface plasmon resonance affinity biosensors for in vitro diagnostics. Int J Anal Chem. 2016;2016:15.
- Eliuk S, Makarov A. Evolution of orbitrap mass spectrometry instrumentation. Annu Rev Anal Chem (Palo Alto Calif). 2015;8(1):61–80.
- Breitling R, Pitt AR, Barrett MP. Precision mapping of the metabolome. Trends Biotechnol. 2006 Dec;24(12):543–548.
- Macek B, Waanders LF, Olsen JV, et al. Top-down protein sequencing and MS3 on a hybrid linear quadrupole ion trap-orbitrap mass spectrometer. Mol Cell Proteomics. 2006 5; May(5): 949–958.
- Robinson CV. Mass spectrometry: from plasma proteins to mitochondrial membranes. Proc Natl Acad Sci U S A. 2019;116(8):2814–2820.
- Frese CK, Altelaar AF, Hennrich ML, et al. Improved peptide identification by targeted fragmentation using CID, HCD and ETD on an LTQ-orbitrap velos. J Proteome Res. 2011 May 6;10(5):2377–2388.
- Peterson AC, Russell JD, Bailey DJ, et al. Parallel reaction monitoring for high resolution and high mass accuracy quantitative, targeted proteomics. Mol Cell Proteomics. 2012 Nov;11(11):1475–1488.
- Paudel A, Raijada D, Rantanen J. Raman spectroscopy in pharmaceutical product design. Adv Drug Deliv Rev. 2015 Jul;15(89):3–20.
- Gala U, Chauhan H. Principles and applications of Raman spectroscopy in pharmaceutical drug discovery and development. Expert Opin Drug Discov. 2015 Feb;10(2):187–206.
- Wang WT, Zhang H, Yuan Y, et al. Research progress of Raman spectroscopy in drug analysis. AAPS PharmSciTech. 2018 Oct;19(7):2921–2928.