1. Introduction
In most therapeutic areas, the reverse chemical biology paradigms that utilize well-known molecular targets to develop specific treatments have become a standard approach in contemporary drug discovery pipelines [Citation1,Citation2]. Unfortunately, recent years have demonstrated significant problems of reverse strategies particularly in development of new, behavior-modifying neuroceuticals [Citation3–6]. The discovery and success rate of such pharmaceuticals, commonly referred to as psycho-active or psycho-modulating drugs, is today noticeably lower than in most other therapeutic areas [Citation3–6]. In fact, a great majority of behavior-modifying neuroceuticals used nowadays in clinical practice were discovered in the middle of the last century during serendipitous phenotypic observations of behavioral changes upon their administration [Citation3–6].
The main reason behind the problems associated with discovery of new behavior-modifying chemicals is the fact that despite decades of very active research in neurobiology, we still have very rudimental understanding of the molecular foundations underlying most psychosomatic conditions [Citation3–6]. In other words, we know much less about the neuro-molecular underpinnings of human brain and behaviors than about mechanisms in all other organ systems. The structural complexity and multifaceted functional integration of the CNS combined with lack of precise knowledge of molecular targets makes most reverse chemical biology paradigms tremendously challenging [Citation3–6].
Complicating the complexity is also the polygenic nature of most neuro-psychiatric conditions. Furthermore, since most psycho-modulating pharmaceuticals usually impact diverse neural and molecular pathways, they tend to exhibit rather poorly characterized pleiotropic activities and often have unpredictable side-effects reaching beyond the CNS [Citation3–6].
2. Revival of phenotypic drug discovery
Since in most cases we do not know molecular targets or do not fully understand the molecular crosstalk underlying psycho-somatic illnesses, the effective solution for drug discovery can be the phenotypic screening, often referred to as classical pharmacology [Citation1–3]. It is a viable and time-tested approach that is often greatly under-appreciated in the modern era of target-specific drug development. In fact, contrary to common opinion, a priori knowledge of the molecular targets is not a necessary pre-requisite for successful discovery or any regulatory approvals of drugs. This is clearly demonstrated by clinical practice and successful use of many existing legacy neuroceuticals [Citation1–3].
Accordingly, there is a renewed and rising interest in performing target-agnostic phenotypic screens to find molecules that serve as better starting points for optimization to develop potential new drugs as well as repurposing existing drugs for use in different therapeutic arenas [Citation1–3]. One of the main challenges associated with discovery of psycho-active drugs is, however, how to effectively perform lead compound prioritization and phenotypic screening at very large scale [Citation3,Citation5,Citation7,Citation8]. Even the most advanced, isolated cell-based systems using in vitro neuronal networks and electrophysiological analysis of their activity cannot hope to recapitulate higher functionalities of the intact CNS that manifest themselves in discrete behavioral phenotypes [Citation3,Citation9]. Despite many attempts made to perfect organotypic cell cultures and more recently even Organ-on-a-Chip technologies, they remain suboptimal solutions for uncovering new behavior-modifying substances [Citation3].
Contrariwise, highly physiologically relevant in vivo rodent models are performed in the context of intact organisms exhibiting desired behavioral phenotypes. Unfortunately, due to their nature, they are extremely low throughput solutions and that require expensive support personnel and infrastructure [Citation3,Citation9–11]. As such they cannot be economically and ethically used to rapidly screen lead compounds for initial characterization of their desired neuroactive potential and thus prioritization for further stages of evaluation. Furthermore, mammals exhibit intricate, complex, and often idiosyncratic behavioral traits that can be a significant impediment to high-throughput data acquisition and computational analysis in the early stages of drug discovery [Citation3].
Accordingly, better proxy models are needed for prioritization of lead compounds. Those need to not only be able to recapitulate functional characteristics of intact CNS but at the same time be amenable for high-throughput screening routines.
3. Fishing for neuroceuticals using zebrafish models
Zebrafish (Danio rerio) is a small, tropical, freshwater fish that in the last two decades has found tremendous applications in fundamental biomedical research and preclinical stages of drug discovery [Citation9–11]. Its advantages are small size, inexpensive, and straightforward husbandry, very high fecundity, as well as optical transparency of embryonal and larval stages. Moreover, very well understood physiology and ease of genetic manipulations enable development of effective transgenic models that recapitulate human pathologies [Citation9–11]. While still allowing for analysis in the context of intact physiological milieu with holistic organ–systems interactions, zebrafish effectively bridges the existing gap between the traditional high-throughput cell-based in vitro assays and low-throughput rodent in vivo tests [Citation9–11].
From the perspective of neuroceuticals discovery, CNS of zebrafish shares significant structural homology with mammals as well as highly evolutionarily conserved molecular and physiological foundations of neuronal signaling [Citation3]. Adult stages of zebrafish exhibit high-level behavioral functions including memory and learning [Citation3,Citation6,Citation10,Citation12]. Complex psycho-somatic deficits such as anxiety, depression, addiction, autism, and obsessive-compulsive state models have been demonstrated using adult zebrafish, opening new vistas for accelerated drug discovery strategies [Citation3,Citation12]. In many respects, zebrafish emerges as a promising alternative and reduced-complexity model organism model for large-scale in vivo phenotypic screening in neuroceutical drug discovery [Citation3,Citation6,Citation12].
4. High-throughput chemobehavioral phenotyping using zebrafish
Apart from advantages of adult models, particular strengths of zebrafish lie in the ability to utilize embryonal and larval stages for rapid screening of chemically induced behavioral phenotypes [Citation3,Citation5–7,Citation12,Citation13]. Those early developmental stages can be kept in multi-titer plates and low volumes of media, thus providing high-throughput screening capabilities on par with in vitro cell culture () [Citation3,Citation5–7,Citation12,Citation13]. Importantly, they exhibit stereotypical behavioral phenotypes that can be used to assess neuro-active and neuro-modulating properties before elevating the prioritized lead compounds to more advanced but also lower throughput adult fish or rodent systems [Citation3,Citation5–7,Citation12,Citation13].
Figure 1. A simplified workflow of the high-throughput chemobehavioral phenotyping strategies using zebrafish at early stages of development and digital video-based animal tracking. Lack of large-scale and automated video-based animal tracking, as well as dedicated bioinformatics approaches that can aid in multi-dimensional behavioral phenoclustering are some of the most significant bottlenecks for effective utilization of high-throughput chemobehavioral screening routines in neuroceuticals drug discovery.
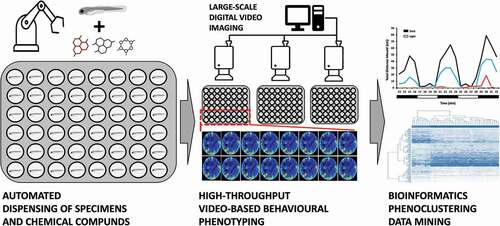
The HT zebrafish strategies implement in situ analysis of behavioral responses upon chemical treatment using recording of short sequences of digital video () [Citation3,Citation14]. The digital recordings can be routinely acquired using dedicated behavioral analysis platforms such as Zebrabox (ViewPoint Inc), Daniovision (Noldus Inc), and Zantiks (Zantiks Inc) [Citation3,Citation14]. Those systems can image an entire 96-well plate using a wide-field infrared camera without any need for cumbersome and complex motorized stages. Furthermore, they are outfitted with programmable light stimulus functionality to elicit photo–motor responses and dedicated animal tracking software necessary for analysis of animal behavioral phenotypes [Citation3,Citation14].
The larval photo–motor response assay (LPR) is one of the most employed HT bioassays to assess if chemicals possess potential neuro-active properties [Citation3,Citation14]. In LPR, freely swimming larval stages of zebrafish at the age of 5–7 days post fertilization (dpf) are used. They exhibit reproducible startle responses to repeated light–/dark phases with a distinct surge in locomotion at the commencement of the dark phase [Citation12].
The light/dark preference bioassay is also commonly employed and measures phototactic behaviors of larvae that demonstrate a natural preference for the illuminated regions. This bioassay was validated for measuring pharmacological modulation of anxiety and depression [Citation3,Citation14].
Recently, an innovative photo–motor response (PMR) bioassay has been developed and is based on stimulation of embryos between 24 and 36 hours post fertilization (hpf) with a light stimulus [Citation7,Citation15]. The latter induces transient increase in the frequency of photo-induced embryo body flexions. PMR can be translated into intricate behavioral fingerprint barcodes used as signatures for categorization of diverse neuroactive properties [Citation7,Citation15].
The rationale of all above assays is that any neuro-modulating activity will be visible in altered behavioral phenotype [Citation12]. The latter can be biometrically classified using database of behavioral fingerprints from a database of experiments performed with known control drugs such as anti-depressants, stimulants, anxiolytics, etc. In this regard, PMR-based phenotyping strategy was recently validated in the screening of a chemical library of 14,000 natural and industrial small molecules [Citation7,Citation15]. Moreover, HT behavioral phenotyping utilizing both PMR and LPR has been demonstrated for analysis of chemical library that included 4,300 known and 20,000 uncharacterized bioactive compounds. This seminal work demonstrated how robust patterns of behavioral phenotypes can be directly translated to applications in the discovery of new neuroactive drugs [Citation15,Citation16]. Importantly, the results were compiled in a PhenoBlast, first behavioral phenotypic database conceptually related to metrics used in existing fields of molecular bioinformatics [Citation15,Citation16].
5. Expert opinion
High-throughput chemobehavioral phenotyping using transgenic and wild-type zebrafish at early stages of development provides unique opportunities for rapid prioritization of neuroactive lead compounds in a context of functional and physiologically intact environment. This unlocks screening of complex poly-pharmacological interactions and organ-specific toxicities otherwise difficult to study holistically using any of the existing in vitro models [Citation6,Citation15,Citation16].
Despite tangible benefits, there are, however, still significant bottlenecks for high-throughput behavioral screening of neuroceuticals. The main reluctance against behavioral phenotyping has traditionally been significant heterogeneity of behavioral endpoints [Citation3,Citation14]. As demonstrated by recent seminal examples, this can be overcome by employing high-throughput routines with large number of replicates and internal positive controls.
At present, these nascent strategies are still hampered by low adoption of specialized high-throughput robotic liquid handling technologies for automatic sorting and dispensing of small aquatic animals into multi-well plates [Citation3,Citation14]. Furthermore, automated video-based animal tracking, generation of behavioral fingerprints, and streamlined biometric data mining are still largely undeveloped for large-scale applications [Citation3,Citation6,Citation14–16]. In particular, the lack of dedicated bioinformatics approaches that can aid in the rapid analysis and multi-dimensional behavioral phenoclustering creates one of the most significant bottlenecks for effective utilization of high-throughput chemobehavioral screening [Citation3,Citation6,Citation14–16]. This is one of the reasons why most studies utilizing behavioral screening have been so far relatively small in scale.
The implementation of artificial intelligence (AI) and machine deep learning algorithms can prospectively open new vistas for rapid and large-scale phenotyping using zebrafish. Recent examples demonstrated the ability to perform automated morphometric analysis and developmental staging of zebrafish embryos on databases of digital images using definiens Cognition Network Technology (DCTN) as well as supervised machine learning [Citation17–19]. Such proof-of-concept technologies have been demonstrated in drug discovery and automated quantification intersegment vessels (ISV) growth in transgenic embryos kept in 96-well plates, regardless of their spatial orientation [Citation17]. An innovative machine learning software called FishInspector was also recently developed for highly reliable automatic detection and quantification of morphometric zebrafish features such as eye size, body length, notochord, head and yolk-sac size, tail malformations, and pigmentation level [Citation20].
At present, there are, however, still no examples of deep learning bioinformatic approaches for large scale analysis of inherently highly variable and data-rich behavioral data obtained from experiments on small model organisms such as zebrafish. In this regard, there is a need for automated techniques that go well beyond software that can simply reconstruct animal trajectories and obtain basic behavioral endpoints [Citation3,Citation5,Citation14]. Diverse AI-enabled paradigms should be explored for elucidation of discrete patterns of behavioral responses as well as generation of digitized behavioral barcodes, construction of behavioral phenoclusters, and development of nonlinear time-course system-level modeling [Citation3,Citation5,Citation14].
In closing the most urgent improvements required for those emergent strategies to mature is thus development of new laboratory automation dedicated for small model organisms as well as data-intensive computational approaches to mine rich biometric data. Behavioral phenotypic databases will also be required for building prospective behavior-based connectivity maps that can in the future provide new vistas for accelerated discovery of neuroceuticals.
Review disclosure
Peer reviewers on this manuscript have no relevant financial or other relationships to disclose.
Declaration of interest
The author has no relevant affiliations or financial involvement with any organization or entity with a financial interest in or financial conflict with the subject matter or materials discussed in the manuscript. This includes employment, consultancies, honoraria, stock ownership or options, expert testimony, grants or patents received or pending, or royalties.
References
- Lee JA, Berg EL. Neoclassic drug discovery: the case for lead generation using phenotypic and functional approaches. J Biomol Screen. 2013 Dec;18(10):1143–1155.
- Zheng W, Thorne N, McKew JC. Phenotypic screens as a renewed approach for drug discovery. Drug Discov Today. 2013 Nov;18(21–22):1067–1073.
- Henry J, Wlodkowic D. Towards high-throughput chemobehavioural phenomics in neuropsychiatric drug discovery. Mar Drugs. 2019 Jun;17(6):340.
- Kokel D, Peterson RT. Using the zebrafish photomotor response for psychotropic drug screening. zebrafish: disease models and chemical screens, 3rd edition. Methods in Cell Biology. 2011;105:517–524.
- Kokel D, Rennekamp AJ, Shah AH, et al. Behavioral barcoding in the cloud: embracing data-intensive digital phenotyping in neuropharmacology. Trends Biotechnol. 2012 Aug;30(8):421–425.
- Kokel D, Peterson RT. Chemobehavioural phenomics and behaviour-based psychiatric drug discovery in the zebrafish. Brief Funct Genomic Proteomic. 2008 Nov;7(6):483–490.
- Kokel D, Bryan J, Laggner C, et al. Rapid behavior-based identification of neuroactive small molecules in the zebrafish. Nat Chem Biol. 2010 Mar;6(3):231–237.
- McCarroll MN, Gendelev L, Keiser MJ, et al. Leveraging large-scale behavioral profiling in zebrafish to explore neuroactive polypharmacology. ACS Chem Biol. 2016 Apr;11(4):842–849.
- MacRae CA, Peterson RT. Zebrafish-based small molecule discovery. Chem Biol. 2003 Oct;10(10):901–908.
- Zon LI, Peterson RT. In vivo drug discovery in the zebrafish. Nat Rev Drug Discov. 2005 Jan;4(1):35–44.
- Rennekamp AJ, Peterson RT. 15 years of zebrafish chemical screening. Curr Opin Chem Biol. 2015 Feb;24:58–70.
- Jenkins JL, Urban L. Fishing for neuroactive compounds. Nat Chem Biol. 2010 Mar;6(3):172–173.
- Laggner C, Kokel D, Setola V, et al. Chemical informatics and target identification in a zebrafish phenotypic screen. Nat Chem Biol. 2012 Feb;8(2):144–146.
- Henry J, and Wlodkowic D. High-throughput animal tracking in chemobehavioral phenotyping: current limitations and future perspectives. Behavioural Processes. 2020 Nov;180:104226.
- Bruni G, Lakhani P, Kokel D. Discovering novel neuroactive drugs through high-throughput behavior-based chemical screening in the zebrafish. Front Pharmacol. 2014 Jul 24; 5. DOI:https://doi.org/10.3389/fphar.2014.00153.
- Bruni G, Rennekamp AJ, Velenich A, et al. Zebrafish behavioral profiling identifies multitarget antipsychotic-like compounds. Nat Chem Biol. 2016 Jul;12(7):559-+.
- Vogt A, Cholewinski A, Shen X, et al. Automated image-based phenotypic analysis in zebrafish embryos. Dev Dyn. 2009 Mar;238(3):656–663.
- Vogt A, Codore H, Day BW, et al. Development of automated imaging and analysis for zebrafish chemical screens. J Vis Exp. 2010. 40. DOI:https://doi.org/10.3791/1900.
- Neukum A, Bartschat A, Breitwieser H, et al. Automated classification of fertilized zebrafish embryos. Zebrafish. 2019 Jun;16(3):326–328.
- Teixido E, Kiessling TR, Krupp E, et al. Automated morphological feature assessment for zebrafish embryo developmental toxicity screens. Toxicol Sci. 2019 Feb 1;167(2):438–449.