1. Introduction
An increasing clinical burden of fungal disease is associated with chronic (e.g. chronic pulmonary aspergillosis), systemic (e.g. candidemia and cryptococcal meningoencephalitis), and recurrent (e.g. vulvovaginal candidiasis) infections. Patient populations, such as those with drug-induced immunosuppression or comorbidities including HIV or stem cell and solid organ transplantation, are at high risk of life-threatening invasive fungal infections (IFIs) [Citation1]. Additionally, the development of resistance to current antifungal therapies, including the emergence of multi-drug resistant species of Candida auris [Citation2] and the increased incidence of voriconazole-resistant Aspergillus fumigatus isolates [Citation3], underscore the need for new approaches in the management of fungal diseases. Discovery and development of agents that overcome resistance mechanisms is crucial. Common mechanisms responsible for innate and acquired drug resistance include the overexpression of plasma membrane efflux pumps belonging to the ATP-binding cassette (ABC) or the major facilitator superfamily (MFS) transporters [Citation4]. Additionally, single mutations in genes of drug targets are also responsible for the development of resistance as it happens in the case of triazoles and mutations of lanosterol 14α-demethylase (Erg11p/CYP51) [Citation5].
Antifungal treatments under evaluation can be grouped into four categories: a. new antifungal classes, b. new agents that belong to an existing class of antifungal agents, c. new indications for established antifungal agents, and d. new antifungal strategies. notes some ongoing clinical trials evaluating antifungal agents. The chemical structures of some representative antifungal agents under clinical drug discovery evaluation are presented in . This editorial outlines some new antifungal concepts and strategies at different stages of evaluation that may supplement the limited existing options for antifungal therapy.
Figure 1. Chemical structures of antifungal agents under clinical evaluation A) Olorofim PubChem Identifier: CID 91885568, URL: https://pubchem.ncbi.nlm.nih.gov/compound/91885568#section=2D-Structure, B) Fosmanogepix PubChem Identifier: 44123754, URL: https://pubchem.ncbi.nlm.nih.gov/compound/44123754#section=2D-Structure C) Rezafungin PubChem Identifier: 78318119, URL: https://pubchem.ncbi.nlm.nih.gov/compound/78318119#section=2D-Structure, D) Ibrexafungerp PubChem Identifier: 46871657, URL: https://pubchem.ncbi.nlm.nih.gov/compound/46871657#section=2D-Structure, E) Isavuconazonium sulfate PubChem Identifier: 72196309, URL: https://pubchem.ncbi.nlm.nih.gov/compound/72196309#section=2D-Structure.
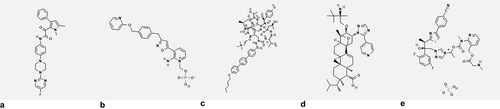
Table 1. Representative recruiting studies.
2. New therapeutic options
The eukaryotic nature of mammalian and fungal cells is a challenge in the discovery and development of new antifungal agents because their shared cellular machinery limits the targets that are specific to fungal pathogens. Current strategies for antifungal drug discovery and development have mainly focused on identifying agents that disrupt the cell wall, cell membrane, or fungal-specific metabolic pathways [Citation6]. Better insights into fungal cell properties, genomics, proteomics, and structural biology have enabled researchers to better understand existing antifungal targets and identify new potential drug targets [Citation6,Citation7]. A list of selected antifungal agents under preclinical development is presented in . A renewed focus on repurposing of drugs used for other diseases provides another promising approach for antifungal drug discovery because their pharmacodynamic, pharmacokinetic, and toxicity profiles are established. For example, tamoxifen, sertraline, and of the celecoxib derivative AR-12 [Citation8] have shown promise as antimycotics [Citation9].
Table 2. Representative antifungal agents under preclinical development.
Identification and discovery of lead compounds that target fungal virulence factors has the potential to prevent fungal disease development. As virulence factors are not essential for antifungal survival agents that target them are less likely to lead to the emergence of resistance [Citation12]. Agents that target morphological transition, adhesion, and biofilm formation in Candida albicans present an example of this approach [Citation13]. This example is species-specific and thus agents with broad spectrum should be evaluated so that commercial interest is drawn. An innovative method for the discovery of compounds with antifungal activity involves the use of probiotics. Probiotics can stimulate the immune system and regulate mycoses [Citation14]. A recent report used the invertebrate model host Galleria mellonella to screen isolates for Lactobacillus paracasei conferring protection against Candida albicans infection [Citation15].
Novel antifungal strategies not involving direct antifungal action exploit immunotherapies that stimulate responses similar to those of the innate and adaptive antifungal immunity. In this context, the use of various cytokines, monoclonal antibodies, immune cells, and growth factors that stimulate host antifungal activity has been proposed [Citation16].
Immune modulating agents with potential antifungal activity have been evaluated in in vitro, animal models, and early case study results which support the importance of larger clinical trials to assess efficacy and safety. Examples of such immunotherapeutic options under evaluation include the use of colony-stimulating factors, IFNγ, IL-12, and IL-15, neutrophilic enrichment by granulocyte infusion (GTX), CAR T cells that target specific fungal antigens, monoclonal antibodies, and checkpoint inhibitors [Citation17].
These new antifungal strategies might benefit from the application of checkpoint inhibitor immunotherapies currently used in cancer patients. For example, inhibition of PD-L1, a common target of checkpoint inhibitors, gave increased levels of autoinflammatory cytokines and upregulation of CTLA-4 activity during A. fumigatus infection [Citation18]. A Phase 2 clinical trial currently recruiting patients will evaluate the efficacy and safety of subcutaneous interferon gamma (IFNγ)-1B in patients with candidemia. Some case reports and case series found infected patients showed increased release of pro-inflammatory cytokines after the administration of IFNγ [Citation16].
An in vivo model using Drosophila melanogaster allows assessment of the antifungal activity, fungal burden, and histopathology caused by azoles directed against C. auris [Citation19]. This model can be exploited in the preclinical testing of candidate antifungals. Insect models, including D. melanogaster and G. mellonella have been used to study the antifungal activity of haemofungin against A. fumigatus and Rhizopus oryzae, respectively [Citation20]. The main mechanism of action of this compound involves the inhibition of ferrochelatase, an enzyme involved in heme biosynthesis, which leads to fungal swelling and lysis [Citation20].
Standard methods for estimating fungistatic and fungicidal activity measure in vitro susceptibility assays by the Clinical Laboratory Standards Institute (CLSI) and the European Committee on Antimicrobial Susceptibility Testing (EUCAST) broth microdilution methods [Citation21]. A study that evaluated the agent MK-3118 (now known as ibrexafungerp) against 71 isolates of Aspergillus spp., showed essential agreement between these two methods [Citation22]. Whole animal high throughput screens based on facile invertebrate models enabled evaluation of the antifungal activity of new compounds. The use of Caenorhabditis elegans, for example, allows high throughput screens both for efficacy and toxicity [Citation23].
Ibrexafungerp, olorofim, and fosmanogepix are promising antifungal agents under clinical development. Ibrexafungerp (or SCY-078) belongs to the newer class of glucan synthase inhibitors (GSI) that is structurally different from echinocandins and binds to a different site in the enzyme. Ibrexfungerp is a semisynthetic modification of a natural product that was identified in a murine model of disseminated candidiasis and gave improved oral efficacy and pharmacokinetics [Citation24]. The agent is active against C. auris [Citation25] and ongoing trials (NCT03059992, NCT03363841, NCT03672292) are evaluating its activity against multi-drug resistant Candida spp. and invasive pulmonary aspergillosis [Citation22]. Significant advantages of this agent may include high tissue penetration and limited drug interactions [Citation26]. In addition to studies that evaluate new antifungal agents as single agents, clinical trials assess drug candidates as part of treatment along with the current standard of care. For example, the SCYNERGIA clinical trial () will evaluate ibrexafungerp along with voriconazole in patients with invasive aspergillosis as a part of a treatment regimen (NCT03672292).
Rezafungin is an example of a new agent under development that may expand the established antifungal class of echinocandins. Similar to other echinocandins, rezafungin appears to have few side effects and limited drug–drug interactions [Citation27,Citation28], while its significantly longer half-life allows for once-weekly dosing [Citation28]. This second-generation echinocandin has shown promising results in treating invasive candidiasis, including candidemia. Preclinical studies in mouse models of disseminated candidiasis and aspergillosis support the efficacy of this agent [Citation29]. Assessment of its efficacy against azole-resistant and azole-susceptible Candida spp. also gave promising results. Rezafungin has the potential for use as prophylaxis against infections caused by Candida spp., Aspergillus spp., and Pneumocystis jirovecii. Rezafungin is currently being evaluated for prophylaxis against invasive fungal infections in the phase 3 ‘ReSPECT’ (NCT04368559) clinical trial () among patients undergoing allogeneic blood and marrow transplantation.
The antifungal olorofim (or F901318) has a novel mode of action. It belongs to the orotomide class and acts by inhibiting dihydroorotate dehydrogenase (an enzyme in the pyrimidine synthesis pathway needed for deoxyribonucleic acid synthesis). Olorofim was discovered by screening of a library of 340,292 compounds using in vitro antifungal susceptibility testing methods [Citation30]. Assessment of olorofim supports its possible use azole against resistant Aspergillus spp. Treatment of central nervous system infections by Coccidioides immitis with olorofim gave encouraging results in a murine model of CNS infection [Citation31]. Notably, olorofim showed anti-biofilm action by inhibiting metabolic activity in early stages of biofilm formation. Olorofim does not show cross-resistance with existing antifungal agents, and can be administered both orally and intravenously [Citation28,Citation30]. Olorofim is currently being evaluated against triazole-resistant Aspergillus spp., Scedosporium/Pseudallescheria complex, Lomentospora prolificans (formerly Scedosporium prolificans), and other difficult to treat mycoses (NCT03583164) but does not appear to have activity against yeast such as Candida spp. due to structural differences in the target enzyme.
Fosmanogepix (or APX001) inhibits the glycosylphosphatidylinositol (GPI)-anchored wall transfer protein 1 (Gwt1), an inositol acyltransferase thereby preventing the maturation of glycosylphosphatidylinositol-anchored (GPI) protein needed for the trafficking and anchoring of mannoproteins to the cell membranes and walls of fungi [Citation32]. Fosmanogepix has shown antifungal activity against Candida spp. resistant to azoles and echinocandins (including C. auris), Cryptococcus neoformans, Cryptococcus gatti, Coccidioides spp., Aspergillus spp. (including azole-resistant Aspergillus fumigatus), Fusarium spp., Scedosporium spp., L. prolificans, and rare or endemic fungal pathogens [Citation31,Citation33]. Fosmanogepix may act synergistically with established antifungal agents including amphotericin B [Citation32] and such combined treatments will be explored in future trials (NCT04240886) for the management of difficult to treat mycoses.
Regarding new indications for existing antifungal agents, isavuconazole (administered in the form of the prodrug isavuconazonium sulfate) is a second-generation broad spectrum triazole recently approved by the Food and Drug Administration (FDA) for adults with invasive aspergillosis and invasive mucormycosis. Isavuconazole has been shown to effectively treat invasive aspergillosis, invasive mucormycosis, and infections by Candida spp and has a better side effect profile and improved pharmacokinetics compared with other broad-spectrum azoles [Citation34]. Currently, isavuconazole is under evaluation for the treatment of pediatric patients with invasive aspergillosis or invasive mucormycosis (NCT03816176) as well as for prophylaxis against pulmonary aspergillosis associated with COVID-19 (NCT04707703) [Citation35].
3. Expert opinion
Various approaches have been employed in the discovery and development of lead antifungal compounds and several promising compounds are in different stages of preclinical and clinical development. We anticipate that the next era of antifungal drug discovery will be based on repurposing of known drugs, the development of antivirulence agents, the use of whole animal high throughput screening assays, further development of existing classes and discovery of agents with new fungal targets and modes of action. Other possibilities for antifungal drug discovery include structure-directed drug discovery, agents that target or circumvent drug efflux mechanisms, and dual-targeting agents.
Antifungal treatment may become more versatile, efficient, and able to overcome the difficulties that affect current clinical practice. For example, new agents and multiple therapeutic combinations have the potential to address the challenge of resistant Aspergillus spp., Candidia spp. and other difficult to treat fungi. These new agents may be implemented alone or in combination with current prophylactic or treatment regimens. Combining drugs (new or established agents) compared with single-agent treatments provides promising efficacy [Citation36]. Drug combinations rapidly reduce fungal population and can act synergistically in resistant microbial strains [Citation37]. Interestingly, drug resistance development might be dealt with or even reversed with the process of inversion [Citation38]. Also, other new agents, such as rezafungin and ibrexafungerp, may provide ease of use, fewer drug–drug interactions, and favorable toxicity profiles [Citation26–28]. Finally, completely new strategies such as immunomodulatory agents may help the immune system fight difficult to treat mycoses.
Incorporating new antifungal treatments into clinical practice depends on the timely completion of large clinical trials. Because systemic fungal infections are relatively rare compared to infections such as influenza or COVID-19, these trials are costly and difficult to run. Also, the patient population at risk is very diverse and stratifying the study participants is particularly challenging. To overcome these problems, a platform that facilitates adaptive design of international clinical trials is needed [Citation39]. In this type of trials, design modifications can be made to one or more aspects of clinical trials based on rules that have been predetermined and by exploiting available trial results. This approach allows for timely and less costly study design. For example, a clinical trial that uses an adaptive dose design could provide improved data on dose-response relationship and facilitate efficacy and safety improvements in studied compounds [Citation39].
Optimal use of existing and new antifungal treatments also depends on accurate and timely diagnosis of fungal infections and the implementation of effective antifungal stewardship programs. Several biomarkers are now under evaluation and may provide assistance in diagnosing and monitoring fungal infections [Citation40]. One example that could be included in clinical trials in order to monitor efficacy of new drugs is use of the T2 magnetic resonance (T2MR) assay that combines magnetic resonance with nanotechnology to identify whole cells of Candida species. This diagnostic tool performed better than blood cultures in monitoring clearance of candidemia [Citation40].
Antifungal stewardship programs improve clinical outcomes, antifungal resistance development. Such stewardship programs are based on adjusting treatment based on diagnostic results, selection of appropriate antifungal agents/dosing, monitoring for toxicity and drug interactions, and minimizing the costs to the health-care system [Citation41].
Fungal infections caused by C. auris, which might have emerged due to of climate change, highlight the potential for global warming to increase the incidence of serious fungal infections and place additional stress on economies with limited resources [Citation42]. Reports on the clinical impact of fungal infections in patients from countries with a limited access to established antifungal treatments and standard of care [Citation43] demonstrate a pressing need for existing and new antifungal treatments to be made available and affordable for all patients who can benefit from them.
In conclusion, new antifungal treatments and strategies can be expected to change clinical practice. Optimal implementation of these treatments will require improved understanding of target and drug efflux-based antifungal resistance mechanisms, the development of rapid molecular diagnostics, and the expansion of antifungal stewardship programs. Global access to antifungal treatment and the development of a new platform for trials evaluating antifungal therapies are major barriers that we have to overcome in order to develop and implement new treatments that decrease the high morbidity and mortality associated with fungal infections.
Article highlights
Description of new antifungal classes under evaluation.
Reports of new agents from established classes and the utilization of commonly used drugs in patients with different indications.
Addition of immunotherapy to the antifungal arsenal.
Dealing with rising antifungal resistance.
Declaration of interest
The authors have no relevant affiliations or financial involvement with any organization or entity with a financial interest in or financial conflict with the subject matter or materials discussed in the manuscript. This includes employment, consultancies, honoraria, stock ownership or options, expert testimony, grants or patents received or pending, or royalties
Reviewer disclosures
A reviewer on this manuscript has disclosed that they are affiliated with Sano Chemicals which is also developing antifungal drugs.
Patient consent statement
This study does not include factors necessitating patient consent.
Additional information
Funding
References
- Brown GD, Denning DW, Gow NA, et al. Hidden killers: human fungal infections. Sci Transl Med. 2012 Dec 19; 4(165):165rv13.
- Forsberg K, Woodworth K, and Walters M, et al. Candida auris: the recent emergence of a multidrug-resistant fungal pathogen. Medical Mycology. 2019 Jan;57(1):1–2. DOI:https://doi.org/10.1093/mmy/myy054.
- van der Linden JW, Camps SM, Kampinga GA, et al. Aspergillosis due to voriconazole highly resistant Aspergillus fumigatus and recovery of genetically related resistant isolates from domiciles. Clin Infect Dis. 2013 Aug;57(4):513–520.
- Cannon RD, Lamping E, Holmes AR, et al. Efflux-mediated antifungal drug resistance. Clin Microbiol Rev. 2009;22(2):291–321.
- Sagatova AA, Keniya MV, Wilson RK, et al. Triazole resistance mediated by mutations of a conserved active site tyrosine in fungal lanosterol 14α-demethylase. Sci Rep. 2016 May 18; 6:26213.
- McCarthy MW, Kontoyiannis DP, Cornely OA, et al. Novel agents and drug targets to meet the challenges of resistant fungi. J Infect Dis. 2017;216(suppl_3):S474–S83.
- Abadio AKR, Kioshima ES, Teixeira MM, et al. Comparative genomics allowed the identification of drug targets against human fungal pathogens. BMC Genomics. 2011 Jan 27; 12(1):75.
- Koselny K, Green J, DiDone L, et al. The celecoxib derivative AR-12 has broad-spectrum antifungal activity in vitro and improves the activity of fluconazole in a murine model of cryptococcosis. Antimicrob Agents Chemother. 2016 Dec;60(12):7115–7127.
- Bouz G, Doležal M. Advances in antifungal drug development: an up-to-date mini review. Pharmaceuticals (Basel). 2021 Dec 16; 14(12):1312.
- Li Z, Tu J, Han G, et al. Novel Carboline Fungal Histone Deacetylase (HDAC) inhibitors for combinational treatment of azole-resistant candidiasis. J Med Chem. 2021 Jan 28; 64(2):1116–1126.
- Roscetto E, Contursi P, Vollaro A, et al. Antifungal and anti-biofilm activity of the first cryptic antimicrobial peptide from an archaeal protein against Candida spp. clinical isolates. Sci Rep. 2018 Dec 4; 8(1):17570.
- Vij R, Hube B, Brunke S. Uncharted territories in the discovery of antifungal and antivirulence natural products from bacteria. Comput Struct Biotechnol J. 2021;19:1244–1252.
- Graham CE, Cruz MR, Garsin DA, et al. Enterococcus faecalis bacteriocin EntV inhibits hyphal morphogenesis, biofilm formation, and virulence of Candida albicans. Proc Natl Acad Sci USA. 2017 Apr 25; 114(17):4507–4512.
- Jorjão AL, de Oliveira FE, Leão MV, et al. Live and heat-killed Lactobacillus rhamnosus ATCC 7469 may induce modulatory cytokines profiles on macrophages RAW 264.7. Sci World J. 2015;2015:716749.
- Rossoni RD, Fuchs BB, de Barros PP, et al. Lactobacillus paracasei modulates the immune system of Galleria mellonella and protects against Candida albicans infection. PLoS One. 2017;12(3):e0173332.
- Posch W, Wilflingseder D, Lass-Flörl C. Immunotherapy as an antifungal strategy in immune compromised hosts. Curr Clin Microbiol Rep. 2020;7(3):57–66.
- Wan L, Zhang Y, Lai Y, et al. Effect of granulocyte-macrophage colony-stimulating factor on prevention and treatment of invasive fungal disease in recipients of allogeneic stem-cell transplantation: a prospective multicenter randomized phase IV trial. J Clin Oncol. 2015 Dec 1;33(34):3999–4006.
- Stephen-Victor E, Karnam A, Fontaine T, et al. Aspergillus fumigatus cell wall α-(1,3)-Glucan stimulates regulatory T-cell polarization by inducing PD-L1 expression on human dendritic cells. J Infect Dis. 2017 Dec 5;216(10):1281–1294.
- Wurster S, Bandi A, Beyda ND, et al. Drosophila melanogaster as a model to study virulence and azole treatment of the emerging pathogen Candida auris. J Antimicrob Chemother. 2019 Jul 1 74(7):1904–1910.
- Ben Yaakov D, Rivkin A, Mircus G, et al. Identification and characterization of haemofungin, a novel antifungal compound that inhibits the final step of haem biosynthesis. J Antimicrob Chemother. 2016 Apr;71(4):946–952.
- Pfaller MA, Watanabe N, Castanheira M, et al. Pre-clinical development of antifungal susceptibility test methods for the testing of the novel antifungal agent E1210 versus Candida: comparison of CLSI and European Committee on Antimicrobial Susceptibility Testing methods. J Antimicrob Chemother. 2011 Nov;66(11):2581–2584.
- Pfaller MA, Messer SA, Motyl MR, et al. In vitro activity of a new oral glucan synthase inhibitor (MK-3118) tested against Aspergillus spp. by CLSI and EUCAST broth microdilution methods. Antimicrob Agents Chemother. 2013 Feb;57(2):1065–1068.
- Pukkila-Worley R, Holson E, Wagner F, et al. Antifungal drug discovery through the study of invertebrate model hosts. Curr Med Chem. 2009;16(13):1588–1595.
- Apgar JM, Wilkening RR, Greenlee ML, et al. Novel orally active inhibitors of β-1,3-glucan synthesis derived from enfumafungin. Bioorg Med Chem Lett. 2015;25(24):5813–5818.
- Davis MR, Donnelley MA, Thompson GR. Ibrexafungerp: a novel oral glucan synthase inhibitor. Med Mycol. 2020 Jul 1;58(5):579–592.
- Wring S, Murphy G, Atiee G, et al. Lack of impact by SCY-078, a first-in-class oral fungicidal glucan synthase inhibitor, on the pharmacokinetics of rosiglitazone, a substrate for CYP450 2C8, supports the low risk for clinically relevant metabolic drug-drug interactions. J Clin Pharmacol. 2018 Oct;58(10):1305–1313.
- Thompson GR, Soriano A, Skoutelis A, et al. rezafungin versus caspofungin in a phase 2, randomized, double-blind study for the treatment of candidemia and invasive candidiasis: the STRIVE trial. Clin Infect Dis. 2021 Dec 6;73(11):e3647–e55.
- Rauseo AM, Coler-Reilly A, Larson L, et al. Hope on the horizon: novel fungal treatments in development. Open Forum Infect Dis. 2020;7(2):ofaa016.
- Miesel L, Lin KY, Ong V. Rezafungin treatment in mouse models of invasive candidiasis and aspergillosis: insights on the PK/PD pharmacometrics of rezafungin efficacy. Pharmacol Res Perspect. 2019 Dec;7(6):e00546.
- Oliver JD, Sibley GEM, Beckmann N, et al. F901318 represents a novel class of antifungal drug that inhibits dihydroorotate dehydrogenase. Proc Natl Acad Sci U S A. 2016 Nov 8;113(45):12809–12814.
- McCarty TP, Pappas PG. Antifungal pipeline. Front Cell Infect Microbiol. 2021;11:732223.
- Wiederhold NP, Najvar LK, Fothergill AW, et al. The investigational agent E1210 is effective in treatment of experimental invasive candidiasis caused by resistant Candida albicans. Antimicrob Agents Chemother. 2015 Jan;59(1):690–692.
- Hata K, Horii T, Miyazaki M, et al. Efficacy of oral E1210, a new broad-spectrum antifungal with a novel mechanism of action, in murine models of candidiasis, aspergillosis, and fusariosis. Antimicrob Agents Chemother. 2011 Oct;55(10):4543–4551.
- Ellsworth M, and Ostrosky-Zeichner L. Isavuconazole: mechanism of action, clinical efficacy, and resistance. Journal of Fungi. DOI:https://doi.org/10.3390/jof6040324.
- Koehler P, Bassetti M, Chakrabarti A, et al. Defining and managing COVID-19-associated pulmonary aspergillosis: the 2020 ECMM/ISHAM consensus criteria for research and clinical guidance. Lancet Infect Dis. 2021 Jun;21(6):e149–e62.
- Spitzer M, Robbins N, Wright GD. Combinatorial strategies for combating invasive fungal infections. Virulence. 2017 Feb 17; 8(2):169–185.
- Wood KB, Wood KC, Nishida S, et al. Uncovering scaling laws to infer multidrug response of resistant microbes and cancer cells. Cell Rep. 2014 Mar 27; 6(6):1073–1084.
- Baym M, Stone LK, Kishony R. Multidrug evolutionary strategies to reverse antibiotic resistance. Science. 2016 Jan 1; 351(6268):aad3292.
- Pallmann P, Bedding AW, Choodari-Oskooei B, et al. Adaptive designs in clinical trials: why use them, and how to run and report them. BMC Med. 2018 Feb 28 16(1):29.
- Thompson GR III, Boulware DR, Bahr NC, et al. Noninvasive testing and surrogate markers in invasive fungal diseases. Open Forum Infect Dis. 2022;9(6):ofac112.
- Johnson MD, Lewis RE, Dodds Ashley ES, et al. Core recommendations for antifungal stewardship: a statement of the mycoses study group education and research consortium. J Infect Dis. 2020 Aug 5 222(Suppl 3):S175–s98.
- Casadevall A, Kontoyiannis DP, Robert V. Environmental Candida auris and the global warming emergence hypothesis. mBio. 2021 Mar 16;12(2). DOI:https://doi.org/10.1128/mBio.00360-21.
- Pfavayi LT, Denning DW, Baker S, et al. Determining the burden of fungal infections in Zimbabwe. Sci Rep. 2021 Jun 24; 11(1):13240.