Abstract
Carbohydrate ingestion can improve endurance capacity and performance. Since the 1980s, research has focused on optimizing the delivery strategies of these carbohydrates. The optimal dose of carbohydrate is still subject to debate, but recent evidence suggests that there may be a dose–response effect as long as the carbohydrate ingested is also oxidized and does not result in gastrointestinal distress. Oxidation rates of a single type of carbohydrate do not exceed 60 g·h−1. However, when multiple transportable carbohydrates are ingested (i.e. glucose and fructose), these oxidation rates can be increased significantly (up to 105 g·h−1). To achieve these high oxidation rates, carbohydrate needs to be ingested at high rates and this has often been associated with poor fluid delivery as well as gastrointestinal distress. However, it has been suggested that using multiple transportable carbohydrates may enhance fluid delivery compared with a single carbohydrate and may cause relatively little gastrointestinal distress. More research is needed to investigate the practical applications of some of the recent findings discussed in this review.
Introduction
In the late 1800s, it was believed that protein was the most important energy source during exercise (von Liebig, Citation1842). This belief gradually changed in the early 1900s when research showed both carbohydrate and fat where important fuels (Zuntz, Citation1901). Nevertheless, the consumption of beef by athletes before important competition and the use of chicken wings during cycling prevailed until the 1970s (Jeukendrup, Craig, & Hawley, Citation2000a). In the late 1960s, it was discovered that carbohydrate was stored as muscle glycogen and glycogen supercompensation protocols were developed and used by many endurance athletes (Bergstrom & Hultman, Citation1967). It was not until the 1980s that research convincingly demonstrated that carbohydrate feeding during prolonged exercise could improve exercise capacity (Coyle & Coggan, Citation1984; Coyle et al., Citation1983). More recently, it has been shown that carbohydrate feeding can also be beneficial in a number of other sporting contexts, such as tennis and football (Ali, Williams, Nicholas, & Foskett, Citation2007; Kovacs, Citation2006; Williams & Serratosa, Citation2006). Although generally carbohydrate feeding may be ergogenic, many questions remain regarding the optimal type and amount of carbohydrate, as well as the timing of intake. The aim of this review is to summarize the current literature and identify the gaps in knowledge that still exist.
Ergogenic effects of carbohydrate intake during prolonged exercise
The beneficial effects of carbohydrate feeding on exercise performance have been well documented. In early studies, the positive effects of carbohydrate feeding were typically seen during exercise lasting at least 2 h (Bjorkman, Sahlin, Hagenfeldt, & Wahren, Citation1984; Coyle et al., Citation1983; Hargreaves, Costill, Coggan, Fink, & Nishibata, Citation1984; Ivy et al., Citation1983; Murray, Seifert, Eddy, Paul, & Halaby, Citation1989; Neufer et al., Citation1987). Most of these studies investigated endurance capacity (measured as time to exhaustion at a constant exercise intensity). A few studies, however, observed positive effects with time-trial protocols in which cyclists had to complete a certain distance as fast as possible. The mechanism behind the ergogenic effect is most likely related to a greater contribution of exogenous carbohydrate (carbohydrate ingested in beverages or other foods), sparing of liver glycogen, prevention of hypoglycaemia, and the maintenance of high rates of carbohydrate oxidation necessary to sustain exercise intensity.
Ergogenic effects of carbohydrate intake during high-intensity exercise
More recent studies have found positive effects of carbohydrate feeding during exercise of relatively high intensity (>75% VO2max) lasting approximately 1 h (Anantaraman, Citation1995; Below, Citation1995; Carter, Citation2003; El-Sayed, Citation1997).For example, Jeukendrup and colleagues (Jeukendrup, Brouns, Wagenmakers, & Saris, 1997) investigated the effects of carbohydrate ingestion during the equivalent of a 40-km time-trial (∼1 h) in well-trained cyclists and found that performance was improved by 2.3%. However, some investigators failed to detect an ergogenic effect of carbohydrate feedings for high-intensity exercise (Clark, Hopkins, Hawley, & Burke, Citation2000; McConell, Canny, Daddo, Nance, & Snow, Citation2000; Powers et al., Citation1990). Carter and colleagues (Carter, Jeukendrup, Mann, & Jones, Citation2004b) concluded that any beneficial effect was unrelated to substrate availability because glucose infusion at high rates did not affect performance; rather, Carter et al. suggested that the effects might operate via the central nervous system. Consistent with this idea, Carter and colleagues (Carter, Jeukendrup, & Jones, Citation2004a) showed that rinsing the mouth with a carbohydrate solution improved cycling performance during a 1-h time-trial by 2–3% even when the participants did not swallow the carbohydrate. This performance improvement was of the same magnitude as that seen with carbohydrate ingestion during a similar exercise task (Jeukendrup et al., Citation1997). These results suggest the existence of receptors in the mouth that communicate with the brain to affect exercise performance. Although direct evidence for such receptors is lacking, it is clear that the brain can sense changes in the composition of the contents of the mouth and stomach. Oropharyngeal receptors, including those situated in the oral cavity, are known to have important roles in perceptual responses during rehydration and exercise in the heat (Maresh et al., Citation2001; Riebe et al., Citation1997). In these studies, ratings of perceived exertion (RPE) and thirst sensations were lower when fluid intake was by mouth compared with intravenous infusion. These findings are supported by reports of temporary reductions in thirst due to the gargling of tap water (Seckl, Williams, & Lightman, Citation1986). Although speculative, it is possible that the triggering of stimuli within the oral cavity by the carbohydrate solution could initiate a chain of neural messages in the central nervous system, resulting in the stimulation of the reward and/or pleasure centres in the brain.
It must be noted that maximal continuous exercise lasting less than 45 min may not benefit from carbohydrate feeding (Palmer et al., Citation1998). At such high exercise intensities, other factors may override a possible beneficial central effect of carbohydrate. Relatively few studies have been conducted using exercise durations less than 1 h, so additional work in this area is needed. However, some laboratories have observed positive effects of carbohydrate drinks on high-intensity intermittent exercise using a shuttle run as a model for team sports such as basketball and soccer (Davis, Welsh, De Volve, & Alderson, Citation1999; Nicholas, Williams, Lakomy, Phillips, & Nowitz, Citation1995; Welsh, Davis, Burke, & Williams, Citation2002).
Although central mechanisms might play a role in enhancing performance during exercise lasting approximately 1 h, the long-established mechanism during more prolonged exercise remains the maintenance of blood glucose concentrations and relatively high rates of carbohydrate oxidation. In this review, the focus will be on more prolonged exercise where the effects of carbohydrate are metabolic rather than central. After the ergogenic effect of carbohydrate on endurance performance had been established in the 1980s, the next obvious objective was to determine the optimal dose.
The optimal dose
There are few published reports of the effects of different doses of carbohydrate on exercise performance. Mitchell and co-workers (Citation1989) compared ingestion of 37, 74, and 111 g of carbohydrate per hour (6%, 12%, and 18% carbohydrate solutions, respectively) or flavoured water. Compared with water, only the trial using 74 g of carbohydrate per hour significantly enhanced the performance of a 12-min isokinetic cycling time-trial following 105 min of continuous exercise. However, all of the performance results for the three carbohydrate trials were statistically similar. In an earlier investigation using a similar isokinetic performance ride, but following 105 min of intermittent exercise, the same authors reported improved performance compared with a water trial for 5%, 6%, and 7.5% carbohydrate solutions (33, 40, and 50 g · h−1, respectively), with no significant differences among the carbohydrate trials (Mitchell et al., Citation1988).However, in this study both the amount and type of carbohydrate ingested were varied.
Fielding and colleagues (Citation1985) reported that a minimum of 22 g of carbohydrate per hour is required to achieve a performance benefit. They had participants perform a cycling sprint after having exercised for 4 h. Performance improvements were observed when 22 g of carbohydrate were ingested every hour, whereas no effects were observed when half this dose was consumed (11 g · h−1). But in an experiment by Maughan and colleagues (Maughan, Bethell, & Leiper, Citation1996), the intake of 16 g of glucose per hour improved endurance capacity by 14% compared with water. However, no placebo was given in this study and therefore the results are less easy to interpret. To add to the uncertainty, Flynn et al. (Citation1987) did not observed any differences in performance with the ingestion of placebo, 5% or 10% carbohydrate solutions that provided 0, 15, and 30 g of carbohydrate per hour, respectively, during 2 h of cycling. The most recent study to address this issue was that by Galloway and colleagues (Galloway, Wootton, Murphy, & Maughan, Citation2001). In this study a 2%, 6% or 12% carbohydrate solution was ingested by individuals exercising in cold conditions (10°C). There was no difference in time to exhaustion at 80% maximum oxygen uptake (VO2max).
In most studies, researchers have provided 40–75 g of carbohydrate per hour and observed performance benefits. Ingesting carbohydrate from a single source (e.g. glucose or maltodextrins) at a rate greater than 60–70 g · h−1 does not appear to be any more effective at improving performance than ingesting carbohydrate at 60–70 g · h−1, perhaps, as will be discussed later, because of limitations in the rate of absorption of a single type of carbohydrate from the intestine. It is also possible that the current performance measurements are not sensitive enough to identify the small differences in performance that may exist when comparing different carbohydrate solutions.
One might conclude that performance benefits can sometimes be observed with the ingestion of relatively small amounts of carbohydrate (e.g., 16 g · h−1), but more reliably so with larger amounts. If carbohydrate ingestion is to improve endurance performance, it is likely that the beneficial effect is primarily dependent on the oxidation of that carbohydrate.
Oxidation of ingested carbohydrate
Several factors can influence the oxidation of exogenous carbohydrate supplied in liquid and solid foods, including feeding schedule, type and amount of carbohydrate ingested, and the exercise intensity. These factors independently affect the rate of carbohydrate oxidation and have been discussed in detail in a recent review.
Type of single-source carbohydrate
Some types of carbohydrate are oxidized more readily than others (Jeukendrup & Jentjens, Citation2000). Clearly, glucose is a carbohydrate that is oxidized quickly as it needs no digestion and is readily absorbed. However, the disaccharides maltose and sucrose can also be oxidized at high rates. Interestingly, glucose polymers are just as rapid and even amylopectin (a branched type of starch) can be oxidized at very high rates. Rowlands and colleagues (Rowlands, Wallis, Shaw, Jentjens, & Jeukendrup, 2005) studied a very high molecular weight glucose polymer. When dissolved in water, this carbohydrate has an extremely low osmolality even at high concentrations. Previous studies with this carbohydrate had shown increased post-exercise glycogen synthesis (Piehl Aulin, Soderlund, & Hultman, Citation2000). The oxidation rate of the high molecular weight glucose polymer, however, was similar to that of glucose (Rowlands et al., Citation2005). This is interesting in itself and shows that the hydrolysis of the bonds within the glucose polymer is not a limiting factor.
Recently, we investigated the oxidation of less common carbohydrates such as isomaltulose and trehalose. Trehalose is a disaccharide similar to maltose in that it is composed of two glucose molecules. The difference is that the glucose molecules in maltose are coupled by a α-1, 4 glycosidic linkage, whereas in trehalose they are bound by an α-1, 1 glycosidic linkage. The different linkages between the glucose molecules give trehalose some interesting properties. Trehalose is only mildly sweet, approximately 45% of the sweetness of sucrose (Higashiyama, Citation2002), which might make it a more palatable solution for athletes. More importantly, it has also been reported to have a low cariogenic effect when ingested either on its own or when added to other sugars (Neta, Takada, & Hirasawa, Citation2000) and could therefore have more favourable effects on dental health. The oxidation of trehalose is significantly lower than that of glucose or maltose (Venables, Brouns, & Jeukendrup, review). Similarly, isomaltulose oxidation during exercise was much lower than sucrose oxidation (Achten, Jentjens, Brouns, & Jeukendrup, Citation2007). Isomaltulose, or 6-0-(∞-d-glucopyranosyl)-d-fructofuranose, is hydrolysed by the sucrase–isomaltase complex located on the outer surface of the brush border membrane of the small intestinal epithelial cells. The resulting monosaccharides glucose and fructose are efficiently taken up into the portal blood. Oxidation rates of isomaltulose are low, probably because disaccharides with α-1,6-glycosidic bonds are hydrolysed at very low rates. Isomaltulose is also interesting from the point of view of oral health, since several studies have suggested a causal relationship between sports drink consumption and dental erosion or plaque formation (Milosevic, Citation1997; Milosevic, Brodie, & Slade, Citation1997). Isomaltulose is not fermented in the oral cavity and does not reduce oral pH, making it less cariogenic (Lingstrom, Lundgren, Birkhed, Takazoe, & Frostell, Citation1997; Peltroche-Llacsahuanga et al., Citation2001).
Carbohydrates can generally be divided into two arbitrary categories: carbohydrates that can be oxidized at rates up to approximately 30–50 g · h−1 and those up to 60–70 g · h−1 ().
Table I. Oxidation of different carbohydrates
Amount of carbohydrate
The optimal amount of ingested carbohydrate is that which results in the maximal rate of exogenous carbohydrate oxidation without causing gastrointestinal discomfort. Rehrer et al. (Citation1992b) studied the oxidation of different amounts of carbohydrate ingested during 80 min of cycling exercise at 70% VO2max. Their participants received either a 4.5% glucose solution (44 g · h−1) or a 17% glucose solution (16 g · h−1). Total exogenous carbohydrate oxidation was only slightly higher with the larger dose of carbohydrate (32 vs. 24 g · h−1). Even though the amount of carbohydrate ingested was increased almost four-fold, the oxidation rate was hardly affected. Jeukendrup et al. (Citation1999) investigated even larger carbohydrate intakes (up to 180 g · h−1) and found that oxidation rates peaked at 56 g · h−1 at the end of 120 min of cycling exercise. These results suggest a limitation in the maximal rate of oxidation of ingested carbohydrates.
Based on the scientific literature in this area, it must be concluded that the maximal rate at which a single source of ingested carbohydrate can be oxidized is about 60–70 g · h−1. Although most research has been conducted with males, the same conclusion seems to hold true for endurance-trained females – that is, the highest rates of exogenous glucose oxidation and the greatest endogenous carbohydrate sparing were observed when carbohydrate was ingested at moderate rates (60 g · h−1) during exercise (Wallis, Yeo, Blannin, & Jeukendrup, 2007). This knowledge implies that athletes who ingest a single type of carbohydrate should ingest about 60–70 g · h−1 for optimal carbohydrate delivery. Ingesting more than this will not increase carbohydrate oxidation rates any further and is likely to be associated with gastrointestinal discomfort.
Multiple transportable carbohydrates
As reviewed by Jeukendrup (Citation2004), it is likely that oxidation of a single exogenous carbohydrate is limited to approximately 60 g · h−1 because there is a limitation in the rate of intestinal absorption of that carbohydrate. It is suggested that by feeding a single carbohydrate source (e.g. glucose, fructose or maltodextrins) at high rates, the specific transporter proteins that aid in absorbing that carbohydrate from the intestine become saturated. Once these transporters are saturated, feeding more of that carbohydrate will not result in greater intestinal absorption and increased oxidation rates.
Shi and colleagues (Citation1995) suggested that the ingestion of carbohydrates that use different transporters might increase total carbohydrate absorption. Subsequently, we began a series of studies using different combinations of carbohydrates to determine their effects on exogenous carbohydrate oxidation. In the first study, participants ingested a drink containing glucose and fructose (Jentjens, Moseley, Waring, Harding, & Jeukendrup, 2004a). Glucose was ingested at a rate of 72 g · h−1 and fructose at a rate of 36 g · h−1. In the control trials, the participants ingested glucose at a rate of 72 g · h−1 and 108 g · h−1 (matching glucose intake or energy intake). We found that the ingestion of glucose at a rate of 72 g · h−1 resulted in oxidation rates of about 48 g · h−1. Ingesting glucose at 108 g · h−1 did not increase the oxidation rate (). However, after ingesting glucose plus fructose, the rate of total exogenous carbohydrate oxidation increased to 76 g · h−1, an increase in oxidation of 45% compared with a similar amount of glucose. In the following years, we tried different combinations and amounts of carbohydrates in an attempt to determine the maximal rate of oxidation of mixtures of exogenous carbohydrates (Jentjens & Jeukendrup, Citation2005; Jentjens et al., Citation2004a, 2005b, Jentjens et al., Citation2006; Jentjens, Venables, & Jeukendrup, 2004b; Wallis et al., Citation2007). We observed very high oxidation rates with combinations of glucose plus fructose, with maltodextrins plus fructose, and with glucose plus sucrose plus fructose (). The highest rates were observed with a mixture of glucose and fructose ingested at a rate of 144 g · h−1. With this feeding regimen, exogenous carbohydrate oxidation peaked at 105 g · h−1 (). This is 75% greater than what was previously thought to be the absolute maximum.
Figure 1. Oxidation of ingested carbohydrate. This figure is compiled from a number of studies in our laboratory investigating the oxidation of exogenous (ingested) carbohydrate during exercise (Jentjens & Jeukendrup, Citation2005; Jentjens et al., Citation2004a, Citationb, Citation2005; Wallis et al., Citation2005). The bars on the left indicate the amount of carbohydrate ingested (g min−1) and those on the right the exogenous carbohydrate oxidation rate. The shaded area shows the maximum range of oxidation rates that can be expected from a single carbohydrate with a maximum oxidation rate of about 1 g min−1. When multiple transportable carbohydrates are ingested at high rates, the oxidation rates can easily exceed 1 g min−1 and the highest oxidation rates were found with a mixture of glucose and fructose (1.75 g min−1).
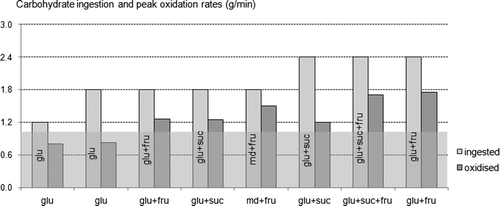
The increased oxidation resulting from the ingestion of multiple types of carbohydrate is theoretically beneficial, although considerably more research needs to be done in this area. In a study in which participants cycled for 5 h at 50% of their maximal work rates (∼58% VO2max) with water, glucose, or glucose plus fructose, there was some indication that ingesting multiple carbohydrates may result in greater improvements in performance (Jeukendrup et al., Citation2006). In this study, carbohydrate was ingested at a rate of 90 g · h−1. The first indication of improved performance was that the participants’ ratings of perceived exertion tended to be lower with the mixture of glucose and fructose compared with glucose alone; the water placebo treatment produced the highest ratings of perceived exertion. In fact, not all participants were able to complete the 5-h ride when they drank the water placebo. In addition, the self-selected cadence dropped significantly with water, which is generally acknowledged as an indication of developing fatigue. With glucose, cycling cadence was somewhat greater than with water, but with glucose plus fructose, cadence was highest and remained almost unchanged from the beginning of exercise. We have since confirmed the beneficial effects on prolonged exercise performance of drinking solutions of glucose plus fructose compared with glucose alone (Currell & Jeukendrup, Citation2008).
We introduced the term “oxidation efficiency” to describe the percentage of the ingested carbohydrate that is oxidized (Jeukendrup & Jentjens, Citation2000). High oxidation efficiency means that smaller amounts of carbohydrate remain in the gastrointestinal tract, reducing the risk of gastrointestinal discomfort that is frequently reported during prolonged exercise (Brouns & Beckers, Citation1993; Rehrer, van Kemenade, Meester, Brouns, & Saris, Citation1992a). Importantly, in our studies, the oxidation efficiency of drinks containing carbohydrates that use different transporters for intestinal absorption was higher than for drinks with a single carbohydrate source. Therefore, compared with a single source of carbohydrate, ingesting multiple carbohydrate sources results in a smaller amount of carbohydrate remaining in the intestine, and osmotic shifts and malabsorption may be reduced. This probably means that drinks with multiple transportable carbohydrates are less likely to cause gastrointestinal discomfort. Interestingly, this is a consistent finding in studies that have attempted to evaluate gastrointestinal discomfort during exercise (Jentjens et al., Citation2004a, Citationb, Citation2005, Citation2006; Jeukendrup et al., Citation2006; Wallis et al., Citation2007). Participants tended to feel less bloated with the glucose plus fructose drinks compared with drinking glucose solutions. A larger-scale study of the effects of drinks with different types of carbohydrates on gastrointestinal discomfort has yet to be conducted.
Exercise intensity
With increasing exercise intensity, the active muscle mass becomes progressively more dependent on carbohydrate as a source of energy. However, the oxidation of exogenous carbohydrate seems to remain constant at intensities of 50–60% VO2max or above (Pirnay et al., Citation1982).
Body size and body mass
The oxidation rate of ingested carbohydrate does not seem to be affected significantly by body size or body mass. The maximal oxidation rate for a single carbohydrate of 60 g min−1 seems to apply to athletes of all body types (A.E. Jeukendrup, unpublished observations). This is why in our studies we have always provided carbohydrate independent of body mass. Although at first sight this might seem counterintuitive, it could be that the gastrointestinal tract, where, most likely the limitation lies, is not different among adult endurance athletes despite differences in body size. In growing children this may be a different story as their gastrointestinal tract is still developing, and studies have generally scaled the oxidation values to body mass (Riddell, Bar-Or, Schwarcz, & Heigenhauser, Citation2000; Riddell, Bar-Or, Wilk, Parolin, & Heigenhauser, Citation2001). Because scaling is an important issue in studies with children, the results are somewhat difficult to interpret and the conclusions depend on the type of scaling used. Nevertheless, it was concluded that the relative oxidation of ingested carbohydrate is considerably higher in boys than in men (Timmons, Bar-Or, & Riddell, Citation2003) when exogenous carbohydrate oxidation was expressed per kilogram of body mass. With the boys in this study having less than half the body mass of the men, different conclusions would have been reached if the data had been expressed differently. Timmons and colleagues (Timmons, Bar-Or, & Riddell, Citation2007) also concluded that exogenous carbohydrate oxidation during exercise was not different between young girls and older adolescent girls when the data were expressed per kilogram of body mass.
Gastrointestinal discomfort during exercise
Gastrointestinal discomfort is very common during exercise, especially in endurance and ultra-endurance sports. Peters et al. (Citation1999) mailed a questionnaire to 606 athletes (runners, cyclists, and triathletes) to assess the prevalence of gastrointestinal problems as well as their training background and nutrition habits. Symptoms presumably originating in the upper gastrointestinal tract (nausea, vomiting, belching, heartburn, chest pain) and in the lower gastrointestinal tract (bloating, abdominal cramps, side ache, urge to defecate, and diarrhoea) were evaluated in all participants; 45–79% reported symptoms of lower gastrointestinal distress, and 36–67% had upper gastrointestinal symptoms. Symptoms generally seem to be more severe during running than cycling, are more prevalent in women than in men, and appear to be more frequent during prolonged exercise. For example, in an extreme long-distance triathlon event, 93% of the participants reported some type of gastrointestinal disturbance, and 45% of these problems were classified as serious (Jeukendrup et al., Citation2000b).
The occurrence of gastrointestinal disturbances has been related to carbohydrate intake during exercise (Brouns & Beckers, Citation1993). A relatively high intake of carbohydrate during exercise may increase the incidence of gastrointestinal symptoms such as diarrhoea and abdominal cramps, either by the osmotic attraction of fluid from the blood into the intestine (Brouns & Beckers, Citation1993) or by malabsorption. The fact that mesenteric blood flow to the intestines is reduced during high-intensity exercise and even more so with dehydration (Brouns & Beckers, Citation1993) might explain the fact that symptoms seem to be more prevalent if exercise is prolonged and performed in hot conditions. Although the occurrence of gastrointestinal distress has been related to carbohydrate intake during exercise, this may be more related to the hyperosmolality of solutions than the actual carbohydrate content (Rehrer et al., 1992b). In fact, in a laboratory study, 7% hypotonic carbohydrate drinks did not result in significantly greater discomfort during 2.5 h of running and cycling compared with water (Peters et al., Citation2000). Although direct evidence is lacking, it is likely that carbohydrate ingested at very high rates (>60 g · h−1), which almost certainly results in hyperosmolality of the stomach contents, will cause an increased incidence of gastrointestinal problems. It is also likely, however, that the gastrointestinal discomfort associated with a particular source or sources of carbohydrate is mainly dictated by the oxidation efficiency of the carbohydrate. It is therefore tempting to speculate that multiple transportable carbohydrates ingested at high rates will be associated with reduced gastrointestinal discomfort while providing carbohydrate at high rates. The tolerance of athletes to large doses of various carbohydrate drinks and the likelihood that an athlete will develop gastrointestinal discomfort seem highly individualized. Therefore, strategies for carbohydrate intake should always be developed on an individual basis, largely by trial and error.
Carbohydrate and fluid delivery
The intake of highly concentrated carbohydrate solutions has been associated with delayed gastric emptying and fluid absorption. However, impairment of fluid delivery may be minimized when combinations of multiple transportable carbohydrates are ingested. We found that fluid delivery with a solution of glucose plus fructose is greater than with a glucose solution (Jentjens et al., Citation2006). Both of these carbohydrate solutions contained about 15 g of carbohydrate per 100 ml (i.e. a 15% carbohydrate solution), and such highly concentrated carbohydrate solutions would normally result in severely impaired fluid delivery (Rehrer et al., Citation1992b). Interestingly, the rate of fluid delivery to the blood, estimated by deuterium concentration, was better with the glucose plus fructose drink compared with glucose alone.
Carbohydrate in hot conditions
Carbohydrate needs in endurance athletes are fairly constant in different environmental conditions, although carbohydrate oxidation rates are somewhat increased in the heat. This increased carbohydrate oxidation is mainly from muscle glycogenolysis, and the contribution from exogenous carbohydrate may actually be decreased (Jentjens, Wagenmakers, & Jeukendrup, 2002). The most logical explanation for this decrease is a redistribution of blood flow to the skin and muscle, with a reduction in blood flow to the intestines. This redistribution of blood would presumably have an adverse effect on carbohydrate absorption. However, combinations of multiple carbohydrates can at least partly overcome this problem, and high rates of exogenous carbohydrate oxidation can be achieved even in these hot conditions (Jentjens et al., Citation2002).
Does carbohydrate ingestion impair metabolic adaptations to training?
Civitarese and colleagues (Civitarese, Hesselink, Russell, Ravussin, & Schrauwen, Citation2005) suggested that carbohydrate ingestion during exercise may suppress the gene expression of oxidative enzymes involved in fat metabolism and might therefore interfere with the process of training adaptations that involve a greater reliance on fat metabolism for energy. They showed that the transcription of several genes related to fat metabolism is transiently induced after exercise when no feeding is provided during exercise, and that glucose feeding interfered with these adaptations. Moreover, Cluberton and co-workers (Cluberton, McGee, Murphy, & Hargreaves, Citation2005) demonstrated that glucose ingestion attenuated the exercise-induced increase in other enzymes involved in energy metabolism and of certain types of messenger RNA. However, there may be a flaw in extrapolating these results to practical use for the athlete; carbohydrate ingestion may allow the athlete to train harder, which would then most likely result in enhanced transcription of metabolic genes. So it may be too early to provide practical advice based on the small number of laboratory studies currently published (Hawley, Tipton, & Millard-Stafford, Citation2006).
Conclusion
Carbohydrate ingestion can improve endurance capacity and performance. The optimal dose of carbohydrate is still subject to debate, but recent evidence suggests that there may be a dose–response effect as long as the carbohydrate ingested is also oxidized and does not result in gastrointestinal distress. Oxidation rates of a single type of carbohydrate do not exceed 60 g · h−1. However, when multiple transportable carbohydrates are ingested (i.e. glucose and fructose), oxidation rates can be increased significantly. To achieve these high oxidation rates, carbohydrate needs to be ingested at high rates and this has usually been associated with poor fluid delivery. There are suggestions, however, that using multiple transportable carbohydrates may enhance fluid delivery compared with a single carbohydrate. More research is needed to investigate the practical applications of some of the findings discussed in this review.
References
- Achten , J. , Jentjens , R. L. , Brouns , F. and Jeukendrup , A. E. 2007 . Exogenous oxidation of isomaltulose is lower than that of sucrose during exercise in men . Journal of Nutrition , 137 : 1143 – 1148 .
- Ali , A. , Williams , C. , Nicholas , C. W. and Foskett , A. 2007 . The influence of carbohydrate-electrolyte ingestion on soccer skill performance . Medicine and Science in Sports and Exercise , 39 : 1969 – 1976 .
- Anantaraman , R. , Carmines , A. A. , Gaesser , G. A. and Weltman , A. 1995 . Effects of carbohydrate supplementation on performance during 1 h of high intensity exercise . International Journal of Sports Medicine , 16 ( 7 ) : 461 – 465 .
- Below , P. R. , Mora-Rodríguez , R. , Gonzáles Alonso , J. and Coyle , E. F. 1995 . Fluid and carbohydrate ingestion independently improve performance during 1 h of intense exercise . Medicine & Science in Sports & Exercise , 27 ( 2 ) : 200 – 210 .
- Bergstrom , J. and Hultman , E. 1967 . A study of glycogen metabolism during exercise in man . Scandinavian Journal of Clinical Investigation , 19 : 218 – 228 .
- Bjorkman , O. , Sahlin , K. , Hagenfeldt , L. and Wahren , J. 1984 . Influence of glucose and fructose ingestion on the capacity for long term exercise in well trained men . Clinical Physiology , 4 : 483 – 494 .
- Brouns , F. and Beckers , E. 1993 . Is the gut an athletic organ? Digestion, absorption and exercise . Sports Medicine , 15 : 242 – 257 .
- Carter , J. M. , Jeukendrup , A. E. and Jones , D. A. 2004a . The effect of carbohydrate mouth rinse on 1-h cycle time-trial performance . Medicine and Science in Sports and Exercise , 36 : 2107 – 2111 .
- Carter , J. M. , Jeukendrup , A. E. , Mann , C. H. and Jones , D. A. 2004b . The effect of glucose infusion on glucose kinetics during a 1-h time-trial . Medicine and Science in Sports and Exercise , 36 : 1543 – 1550 .
- Carter , J. , Jeukendrup , A. E. , Mundel , T. and Jones , D. A. 2003 . Carbohydrate supplementation improves moderate and high-intensity exercise in the heat . Pflügers Archiv European Journal of Physiology , 446 ( 2 ) : 211 – 219 .
- Civitarese , A. E. , Hesselink , M. K. , Russell , A. P. , Ravussin , E. and Schrauwen , P. 2005 . Glucose ingestion during exercise blunts exercise-induced gene expression of skeletal muscle fat oxidative genes . American Journal of Physiology: Endocrinology and Metabolism , 289 : E1023 – E1029 .
- Clark , V. R. , Hopkins , W. G. , Hawley , J. A. and Burke , L. M. 2000 . Placebo effect of carbohydrate feedings during a 40-km cycling time-trial . Medicine and Science in Sports and Exercise , 32 : 1642 – 1647 .
- Cluberton , L. J. , McGee , S. L. , Murphy , R. M. and Hargreaves , M. 2005 . Effect of carbohydrate ingestion on exercise-induced alterations in metabolic gene expression . Journal of Applied Physiology , 99 : 1359 – 1363 .
- Coyle , E. F. and Coggan , A. R. 1984 . Effectiveness of carbohydrate feeding in delaying fatigue during prolonged exercise . Sports Medicine , 1 : 446 – 458 .
- Coyle , E. F. , Hagberg , J. M. , Hurley , B. F. , Martin , W. H. , Ehsani , A. A. and Holloszy , J. O. 1983 . Carbohydrate feeding during prolonged strenuous exercise . Journal of Applied Physiology , 55 : 230 – 235 .
- Currell , K. and Jeukendrup , A. E. 2008 . Superior endurance performance with ingestion of multiple transportable carbohydrates . Medicine and Science in Sports and Exercise , 40 : 275 – 281 .
- Davis , J. M. , Welsh , R. S. , De Volve , K. L. and Alderson , N. A. 1999 . Effects of branched-chain amino acids and carbohydrate on fatigue during intermittent, high-intensity running . International Journal of Sports Medicine , 20 : 309 – 314 .
- Décombaz , J. , Sartori , D. , Arnaurd , M. J. , Thelin , A. L. , Schurch , P. and Howald , H. 1985 . Oxidation and metabolic effects of fructose and glucose ingested before exercise . International Journal of Sports Medicine , 6 : 282 – 286 .
- el-Sayed , M. S. , Balmer , J. and Rattu , A. J. M. 1997 . Carbohydrate ingestion improves endurance performance during a 1 h simulated cycling time trial . Journal of Sports Sciences , 15 ( 2 ) : 223 – 230 .
- Fielding , R. A. , Costill , D. L. , Fink , W. J. , King , D. S. , Hargreaves , M. and Kovaleski , J. E. 1985 . Effect of carbohydrate feeding frequencies and dosage on muscle glycogen use during exercise . Medicine and Science in Sports and Exercise , 17 : 472 – 476 .
- Flynn , M. G. , Costill , D. L. , Hawley , J. A. , Fink , W. J. , Neufer , P. D. Fielding , R. A. 1987 . Influence of selected carbohydrate drinks on cycling performance and glycogen use . Medicine and Science in Sports and Exercise , 19 : 37 – 40 .
- Galloway , S. D. R. , Wootton , S. A. , Murphy , J. L. and Maughan , R. J. 2001 . Exogenous carbohydrate oxidation from drinks ingested during prolonged exercise in a cold environment in humans . Journal of Applied Physiology , 91 : 654 – 660 .
- Guezennec , C. Y. , Satabin , P. , Duforez , F. , Merino , D. , Peronnet , F. and Koziet , J. 1989 . Oxidation of corn starch, glucose, and fructose ingested before exercise . Medicine and Science in Sports and Exercise , 21 : 45 – 50 .
- Hargreaves , M. , Costill , D. L. , Coggan , A. , Fink , W. J. and Nishibata , I. 1984 . Effect of carbohydrate feedings on muscle glycogen utilisation and exercise performance . Medicine and Science in Sports and Exercise , 16 : 219 – 222 .
- Hawley , J. A. , Dennis , S. C. , Nowitz , A. , Brouns , F. and Noakes , T. D. 1992 . Exogenous carbohydrate oxidation from maltose and glucose ingested during prolonged exercise . European Journal of Applied Physiology , 64 : 523 – 527 .
- Hawley , J. A. , Tipton , K. D. and Millard-Stafford , M. L. 2006 . Promoting training adaptations through nutritional interventions . Journal of Sports Sciences , 24 : 709 – 721 .
- Higashiyama , T. 2002 . Novel functions and applications of trehalose . Pure and Applied Chemistry , 74 : 1263 – 1269 .
- Ivy , J. L. , Miller , W. , Dover , V. , Goodyear , L. G. , Sherman , W. M. Farrell , S. 1983 . Endurance improved by ingestion of a glucose polymer supplement . Medicine and Science in Sports and Exercise , 15 : 466 – 471 .
- Jentjens , R. L. P. G. and Jeukendrup , A. E. 2005 . High rates of exogenous carbohydrate oxidation from a mixture of glucose and fructose ingested during prolonged cycling exercise . British Journal of Nutrition , 93 : 485 – 492 .
- Jentjens , R. L. P. G. , Moseley , L. , Waring , R. H. , Harding , L. K. and Jeukendrup , A. E. 2004a . Oxidation of combined ingestion of glucose and fructose during exercise . Journal of Applied Physiology , 96 : 1277 – 1284 .
- Jentjens , R. L. P. G. , Shaw , C. , Birtles , T. , Waring , R. H. , Harding , L. K. and Jeukendrup , A. E. 2005 . Oxidation of combined ingestion of glucose and sucrose during exercise . Metabolism , 54 : 610 – 618 .
- Jentjens , R. L. P. G. , Underwood , K. , Achten , J. , Currell , K. , Mann , C. H. and Jeukendrup , A. E. 2006 . Exogenous carbohydrate oxidation rates are elevated after combined ingestion of glucose and fructose during exercise in the heat . J Appl Physiol , 100 ( 3 ) : 807 – 816 .
- Jentjens , R. L. P. G. , Venables , M. C. and Jeukendrup , A. E. 2004b . Oxidation of exogenous glucose, sucrose, and maltose during prolonged cycling exercise . Journal of Applied Physiology , 96 : 1285 – 1291 .
- Jentjens , R. L. P. G. , Wagenmakers , A. J. M. and Jeukendrup , A. E. 2002 . Heat stress increases muscle glycogen use but reduces the oxidation of ingested carbohydrates during exercise . Journal of Applied Physiology , 92 : 1562 – 1572 .
- Jeukendrup , A. E. 2004 . Carbohydrate intake during exercise and performance . Nutrition , 20 : 669 – 677 .
- Jeukendrup , A. E. , Brouns , F. , Wagenmakers , A. J. M. and Saris , W. H. M. 1997 . Carbohydrate-electrolyte feedings improve 1 h time-trial cycling performance . International Journal of Sports Medicine , 18 : 125 – 129 .
- Jeukendrup , A. E. , Craig , N. P. and Hawley , J. A. 2000a . The bioenergetics of World Class Cycling . Journal of Science and Medicine in Sport , 3 : 414 – 433 .
- Jeukendrup , A. E. and Jentjens , R. L. P. G. 2000 . Oxidation of carbohydrate feedings during prolonged exercise: current thoughts, guidelines and directions for future research . Sports Med , 29 ( 6 ) : 407 – 424 .
- Jeukendrup , A. E. , Moseley , L. , Mainwaring , G. I. , Samuels , S. , Perry , S. and Mann , C. H. 2006 . Exogenous carbohydrate oxidation during ultraendurance exercise . Journal of Applied Physiology , 100 : 1134 – 1141 .
- Jeukendrup , A. E. , Vet-Joop , K. , Sturk , A. , Stegen , J. H. , Senden , J. Saris , W. H. 2000b . Relationship between gastro-intestinal complaints and endotoxaemia, cytokine release and the acute-phase reaction during and after a long-distance triathlon in highly trained men . Clinical Science (Colchester) , 98 : 47 – 55 .
- Jeukendrup , A. E. , Wagenmakers , A. J. , Stegen , J. H. , Gijsen , A. P. , Brouns , F. and Saris , W. H. 1999 . Carbohydrate ingestion can completely suppress endogenous glucose production during exercise . American Journal of Physiology: Endocrinology and Metabolism , 276 : E672 – E683 .
- Kovacs , M. S. 2006 . Carbohydrate intake and tennis: are there benefits? . British Journal of Sports Medicinee , 40 : e13
- Leijssen , D. P. C. , Saris , W. H. M. , Jeukendrup , A. E. and Wagenmakers , A. J. M. 1995 . Oxidation of exogenous [13C]galactose and [13C]glucose during exercise . Journal of Applied Physiology , 79 : 720 – 725 .
- Lingstrom , P. , Lundgren , F. , Birkhed , D. , Takazoe , I. and Frostell , G. 1997 . Effects of frequent mouthrinses with palatinose and xylitol on dental plaque . European Journal of Oral Science , 105 : 162 – 169 .
- Maresh , C. M. , Herrera-Soto , J. A. , Armstrong , L. E. , Casa , D. J. , Kavouras , S. A. Hacker , F. T. 2001 . Perceptual responses in the heat after brief intravenous versus oral rehydration . Medicine and Science in Sports and Exercise , 33 : 1039 – 1045 .
- Maughan , R. J. , Bethell , L. R. and Leiper , J. B. 1996 . Effects of ingested fluids on exercise capacity and on cardiovascular and metabolic responses to prolonged exercise in man . Experimental Physiology , 81 : 847 – 859 .
- McConell , G. K. , Canny , B. J. , Daddo , M. C. , Nance , M. J. and Snow , R. J. 2000 . Effect of carbohydrate ingestion on glucose kinetics and muscle metabolism during intense endurance exercise . Journal of Applied Physiology , 89 : 1690 – 1698 .
- Milosevic , A. 1997 . Sports drinks hazard to teeth . British Journal of Sports Medicine , 31 : 28 – 30 .
- Milosevic , A. , Brodie , D. A. and Slade , P. D. 1997 . Dental erosion, oral hygiene, and nutrition in eating disorders . International Journal of Eating Disorders , 21 : 195 – 199 .
- Mitchell , J. B. , Costill , D. L. , Houmard , J. A. , Fink , W. J. , Pascoe , D. D. and Pearson , D. R. 1989 . Influence of carbohydrate dosage on exercise performance and glycogen use . Journal of Applied Physiology , 67 : 1843 – 1849 .
- Mitchell , J.B. , Costill , D. L. , Houmard , J. A. , Flynn , M. G. , Fink , W. J. and Beltz , J. D. 1988 . Effects of carbohydrate ingestion on gastric emptying and exercise performance . Medicine & Science in Sports & Exercise , 20 ( 2 ) : 110 – 115 .
- Murray , R. , Seifert , J. G. , Eddy , D. E. , Paul , G. L. and Halaby , G. A. 1989 . Carbohydrate feeding and exercise: effect of beverage carbohydrate content . European Journal of Applied Physiology , 59 : 152 – 158 .
- Neta , T. , Takada , K. and Hirasawa , M. 2000 . Low-cariogenicity of trehalose as a substrate . Journal of Dentistry , 28 : 571 – 576 .
- Neufer , D. , Costill , D. L. , Flynn , M. G. , Kirwan , J. P. , Mitchell , J. B. and Houmard , J. 1987 . Improvements in exercise performance: effects of carbohydrate feedings and diet . Journal of Applied Physiology , 62 : 983 – 988 .
- Nicholas , C. W. , Williams , C. , Lakomy , H. K. A. , Phillips , G. and Nowitz , A. 1995 . Influence of ingesting a carbohydrate-electrolyte solution on endurance capacity during intermittent, high-intensity shuttle running . Journal of Sports Sciences , 13 : 283 – 290 .
- Palmer , G. S. , Clancy , M. C. , Hawley , J. A. , Rodger , I. M. , Burke , L. M. and Noakes , T. D. 1998 . Carbohydrate ingestion immediately before exercise does not improve 20km time-trial performance in well trained cyclists . International Journal of Sports Medicine , 19 : 415 – 418 .
- Peltroche-Llacsahuanga , H. , Hauk , C. J. , Kock , R. , Lampert , F. , Lutticken , R. and Haagse , G. 2001 . Assessment of acid production by various human oral micro-organisms when palatinose or leucrose is utilized . Journal of Dental Research , 80 : 378 – 384 .
- Peters , H. P. F. , Bos , M. , Seebregts , L. , Akkermans , L. M. A. , van Berge Henegouwen , G. P. Bol , E. 1999 . Gastrointestinal symptoms in long-distance runners, cyclists, and triathletes: prevalence, medication, and etiology . American Journal of Gastroenterology , 94 : 1570 – 1581 .
- Peters , H. P. F. , Wiersma , J. W. C. , Koerselman , J. , Akkermans , L. M. A. , Bol , E. Mosterd , W. L. 2000 . The effect of a sports drink on gastroesophageal reflux during a run–bike–run test . International Journal of Sports Medicine , 21 : 65 – 70 .
- Piehl Aulin , K. , Soderlund , K. and Hultman , E. 2000 . Muscle glycogen resynthesis rate in humans after supplementation of drinks containing carbohydrates with low and high molecular masses . European Journal of Applied Physiology , 81 : 346 – 351 .
- Pirnay , F. , Crielaard , J. M. , Pallikarakis , N. , Lacroix , M. , Mosora , F. Krzentowski , G. 1982 . Fate of exogenous glucose during exercise of different intensities in humans . Journal of Applied Physiology , 53 : 1620 – 1624 .
- Powers , S. K. , Lawler , J. , Dodd , S. , Tulley , R. , Landry , G. and Wheeler , K. 1990 . Fluid replacement drinks during high intensity exercise: effects on minimizing exercise-induced disturbances in homeostasis . European Journal of Applied Physiology , 60 : 54 – 60 .
- Rehrer , N. J. , van Kemenade , M. , Meester , W. , Brouns , F. and Saris , W. H. 1992a . Gastrointestinal complaints in relation to dietary intake in triathletes . International Journal of Sport Nutrition , 2 : 48 – 59 .
- Rehrer , N. J. , Wagenmakers , A. J. M. , Beckers , E. J. , Halliday , D. , Leiper , J. B. Brouns , F. 1992b . Gastric emptying, absorption and carbohydrate oxidation during prolonged exercise . Journal of Applied Physiology , 72 : 468 – 475 .
- Riddell , M. C. , Bar-Or , O. , Schwarcz , H. P. and Heigenhauser , G. J. F. 2000 . Substrate utilization in boys during exercise with [13C]-glucose ingestion . European Journal of Applied Physiology , 83 : 441 – 448 .
- Riddell , M. C. , Bar-Or , O. , Wilk , B. , Parolin , M. L. and Heigenhauser , G. J. F. 2001 . Substrate utilization during exercise with glucose and glucose plus fructose ingestion in boys aged 10–14 yr . Journal of Applied Physiology , 90 : 903 – 911 .
- Riebe , D. , Maresh , C. M. , Armstrong , L. E. , Kenefick , R. W. , Castellani , J. W. Echegaray , M. E. 1997 . Effects of oral and intravenous rehydration on ratings of perceived exertion and thirst . Medicine and Science in Sports and Exercise , 29 : 117 – 124 .
- Rowlands , D. S. , Wallis , G. A. , Shaw , C. , Jentjens , R. L. P. G. and Jeukendrup , A. E. 2005 . Glucose polymer molecular weight does not affect exogenous carbohydrate oxidation . Medicine and Science in Sports and Exercise , 37 : 1510 – 1516 .
- Saris , W. H. M. , Goodpaster , B. H. , Jeukendrup , A. E. , Brouns , F. , Halliday , D. and Wagenmakers , A. J. M. 1993 . Exogenous carbohydrate oxidation from different carbohydrate sources during exercise . Journal of Applied Physiology , 75 : 2168 – 2172 .
- Seckl , J. R. , Williams , T. D. M. and Lightman , S. L. 1986 . Oral hypertonic saline causes transient fall of vasopressin in humans. American Journal of Physiology: Regulatory . Integrative and Comparative Physiology , 251 : R214 – R217 .
- Shi , X. , Summers , R. W. , Schedl , H. P. , Flanagan , S. W. , Chang , R. and Gisolfi , C. V. 1995 . Effects of carbohydrate type and concentration and solution osmolality on water absorption . Medicine and Science in Sports and Exercise , 27 ( 12 ) : 1607 – 1615 .
- Timmons , B. W. , Bar-Or , O. and Riddell , M. C. 2003 . Oxidation rate of exogenous carbohydrate during exercise is higher in boys than in men . Journal of Applied Physiology , 94 : 278 – 284 .
- Timmons , B. W. , Bar-Or , O. and Riddell , M. C. 2007 . Energy substrate utilization during prolonged exercise with and without carbohydrate intake in preadolescent and adolescent girls . Journal of Applied Physiology , 103 : 995 – 1000 .
- Venables , M. C. , Brouns , F. , & Jeukendrup , A. E. (review). Oxidation of maltose and trehalose during prolonged moderate intensity exercise . Medicine and Science in Sports and Exercise .
- von Liebig , J. 1842 . Animal chemistry or organic chemistry in its application to physiology and pathology , London : Taylor & Walton .
- Wagenmakers , A. J. M. , Brouns , F. , Saris , W. H. M. and Halliday , D. 1993 . Oxidation rates of orally ingested carbohydrates during prolonged exercise in man . Journal of Applied Physiology , 75 : 2774 – 2780 .
- Wallis , G. A. , Rowlands , D. S. , Shaw , C. , Jentjens , R. L. G. P. and Jeukendrup , A. E. 2005 . Oxidation of combined ingestion of maltodextrins and fructose during exercise . Medicine and Science in Sports and Exercise , 37 : 426 – 432 .
- Wallis , G. A. , Yeo , S. E. , Blannin , A. K. and Jeukendrup , A. E. 2007 . Dose–response effects of ingested carbohydrate on exercise metabolism in women . Medicine and Science in Sports and Exercise , 39 : 131 – 138 .
- Welsh , R. S. , Davis , J. M. , Burke , J. R. and Williams , H. G. 2002 . Carbohydrates and physical/mental performance during intermittent exercise to fatigue . Medicine and Science in Sports and Exercise , 34 : 723 – 731 .
- Williams , C. and Serratosa , L. 2006 . Nutrition on match day . Journal of Sports Sciences , 24 : 687 – 697 .
- Zuntz , N. 1901 . ber die Bedeutung der verschiedene Nahrstoffe als Erzeuber der Muskelkraft . Pflüglers Archive , 83 : 557 – 571 .