Abstract
Scientists who have examined the gaze strategies employed by athletes have determined that longer quiet eye (QE) durations (QED) are characteristic of skilled compared to less-skilled performers. However, the cognitive mechanisms of the QE and, specifically, how the QED affects performance are not yet fully understood. We review research that has examined the functional mechanism underlying QE and discuss the neural networks that may be involved. We also highlight the limitations surrounding QE measurement and its definition and propose future research directions to address these shortcomings. Investigations into the behavioural and neural mechanisms of QE will aid the understanding of the perceptual and cognitive processes underlying expert performance and the factors that change as expertise develops.
Keywords:
The QE
In recent years, attention has been devoted to examining the gaze behaviours employed by expert performers across different sports, as well as in other domains. Vickers (Citation1992) highlighted distinct gaze patterns that differentiate expert and novice golfers while performing putts and identified that experts kept a steady fixation at a specific location before ball contact. This steady fixation just before movement initiation was later identified in basketball players and termed “quiet eye” (QE; Vickers, Citation1996).
The QE corresponds to the final fixation within 1–3 degrees of visual angle and with a duration of at least 100 ms prior to a movement. Longer quiet eye durations (QED) are exhibited by experts compared with non-experts, and within-participant analyses show that they are characteristic of successful rather than unsuccessful attempts (Vickers, Citation1996; Vickers & Williams, Citation2007). In addition, with the use of video-based mobile eye-tracking systems, these findings have been replicated across various types of aiming and interceptive sports, including shooting (Causer, Bennett, Holmes, Janelle, & Williams, Citation2010), darts (Rienhoff et al., Citation2013), billiards (Williams, Singer, & Frehlich, Citation2002), table tennis (Rodrigues, Vickers, & Williams, Citation2002) and football (Piras & Vickers, Citation2011).
A number of researchers have successfully used the QE as a training tool to improve performance in different targeting sports (Causer, Holmes, & Williams, Citation2011; Moore, Vine, Cooke, Ring, & Wilson, Citation2012; Vine & Wilson, Citation2011) and recently, outside the sporting area such as when training surgical skills (Causer, Harvey, Snelgrove, Arsenault, & Vickers, Citation2014) and in clinical populations (Miles, Wood, Vine, Vickers, & Wilson, Citation2015). In these studies, QE training (where to look and for how long) results in enhanced performance linked to relative increases in QED (for a detailed review, see Vine, Moore, & Wilson, Citation2014). However, whether or not the duration of the QE per se causes these improvements in performance and how these benefits come into place are still subjects of interest. This research has highlighted the need to better understand the underlying mechanisms of QE and, in particular, investigate the beneficial effects of the QE on performance to implement effective training protocols (Behan & Wilson, Citation2008).
Much of the research on QE has focused on identifying distinct QED effects amongst expert and novice performers and in evaluating the training of QE as a performance-enhancing technique. A review that focuses on the latter and on learning under anxiety can be found in Vine et al. (Citation2014). Vine et al. (Citation2014) propose three potential explanations (attention control, focus of attention and response planning) of how QE may benefit performance and expedite learning in target sports. However, the authors only present one study in which the QE was directly manipulated and questions about the causality of the QED on performance still remain. In addition, limitations in the QE methodology have not yet been adequately addressed. Thus, in this paper, we review current research examining the underlying mechanisms of the QE. Moreover, based on this current research, we propose potential neural networks that may be involved in QE. These notions may provide further insights into the facilitatory effects of longer QED on performance. In addition, we aim to highlight limitations surrounding the QE definition and measurement techniques, as well as the potential impact these may have on the interpretation of current literature. Finally, we propose future research directions to better understand the critical processes involved in QE.
The programming hypothesis
The QE is suggested to facilitate information processing and its duration reflects the time needed to programme and fine-tune a response (Vickers, Citation2009). Long QEDs are suggested to extend the critical motor preparation period that consists of response selection and the fine tuning of movement parameters for motor programming (Moore et al., Citation2012). Williams et al. (Citation2002) manipulated the complexity of a billiards shot (near versus far) as well as the time allowed to complete a specific shot (constrained versus unconstrained time) and demonstrated that QEDs were longer in skilled performers and in successful compared with unsuccessful shots. These authors further demonstrated longer QEDs with increasing levels of task complexity and when increasing the time allowed to complete the task (time available for motor programming), in skilled and less-skilled billiard players. This evidence supports the programming hypothesis, as longer QEDs were observed in more complex tasks that required more information processing and longer programming times (Vickers, Citation2011).
Horn, Okumura, Alexander, Gardin, and Sylvester (Citation2012) investigated QED and the effects of variable versus blocked practice in a dart-throwing task. They hypothesised that random target changes (to different targets at similar distances) would produce longer QED due to the increased programming demands of having to parameterise the response from one trial to the next, compared to the consistency of the blocked target presentation (same target and distance). Although performance (accuracy) differences between the practice groups were not apparent, longer QEDs were observed during random compared to blocked practice, which likely reflected an increase in cognitive effort during random practice to compensate for the additional task demands (of online movement parameterisation) (). Horn et al. (Citation2012) concluded that the longer QED was a functional element of programming demand rather than a by-product of successful aiming.
Figure 1. The graph displays the mean QED and standard deviations (SD) of random and blocked practice (RP and BP, respectively) groups in targets aimed in the horizontal and vertical axes (HA and VA, respectively) during a dart-throwing task (adapted from Horn et al., Citation2012). The random practice group exhibited longer QED compared to participants trained under blocked practice conditions. Despite these QED differences, the authors reported no significant correlations between accuracy of the throw and QED.
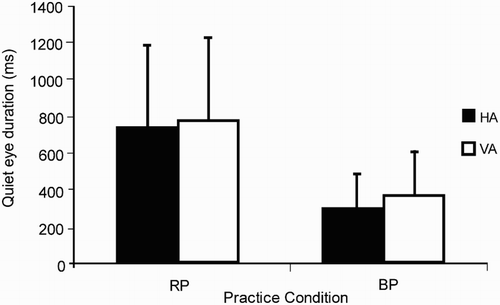
As with previous research, however, the QED effects on performance found in Williams et al.’s (Citation2002) and Horn et al.’s (Citation2012) studies were shown as a correlation of performance and did not reveal a causal relationship between QE and performance. In a recent study, Klostermann, Kredel, and Hossner (Citation2013) investigated the performance-enhancing effects of experimentally manipulated QEDs by varying response selection and stimulus identification demands. In their experiments, participants took part in an externally paced throwing task in which the onset of the last fixation and the amount of information to be processed were manipulated by presenting the target at different timings (short and long presentations) and locations (random and predictive) during movement unfolding. They showed that the facilitatory effects of longer QED on performance were apparent under a high information-processing load; however, QED effects seemed to disappear with increased predictability of the target's location and lower task demands (LTD) ().
Figure 2. The figure displays the externally paced throwing task (a) implemented by Klostermann et al. (Citation2013). Figure (b) shows the mean and SD radial error (measure of performance) and (c) displays the mean and SD QE onset and offset during the low task demands and high task demands conditions (LTD and HTD, respectively) in short and long target presentations (SP and LP, respectively). Statistically significant findings are highlighted (p < .05 * and **). Complexity was manipulated by implementing a random and a predictive target presentation during movement unfolding. Their results showed earlier QE onsets, which resulted in longer QED, during more complex tasks (random targets) and during longer compared to short target presentations. A significant interaction was found between task demands and target duration presentation which revealed that participants threw more accurately in LP compared to SP but only in HTDs conditions. Adapted from Klostermann et al. (Citation2013).
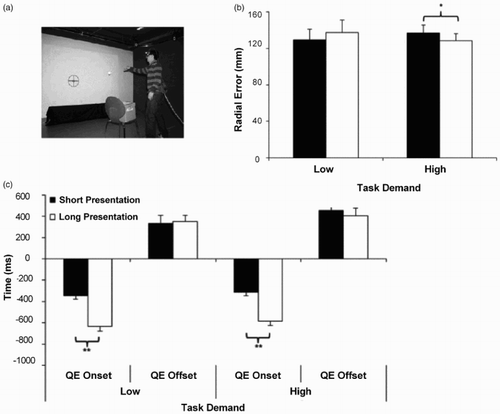
Klostermann et al. (Citation2013) argued that the predictability of the target might have facilitated relevant information processing that was not required during the QE period, compared to random target conditions. Consequently, QED effects were “dispensable” with high predictability and LTDs. This finding suggests that continuous monitoring and online control may play an important role during aiming tasks (cf., Horn et al., Citation2012), highlighting the need to investigate these mechanisms while maintaining information load constant, as proposed by Klostermann et al. (Citation2013). This finding of online control is in accordance to De Oliveira, Huys, Oudejans, van de Langenberg, and Beek (Citation2007) and Vine, Lee, Moore, and Wilson (Citation2013), who suggested that this mechanism is important for the maintenance of QE; thus, it is unlikely that the QE involves only open-loop early programming. This conclusion comes from evidence suggesting that late information pick-up is important for accurate basketball shooting (De Oliveira et al., Citation2007) and continued accurate gaze control is critical for preventing “choking” effects and performance failure in expert golfers (Vine et al., Citation2013). These data, together with Williams et al.’s (Citation2002) and Horn et al.’s (Citation2012) findings, provide evidence for functional links between information processing and the effects of prolonged QED on performance. However, the precise information that is important remains unclear. Furthermore, additional mechanisms could be involved in QE and may prove to be more critical than the programming itself, such as the continued integration of sensory information, which may be facilitated by attention allocation.
QED and attentional focus
The allocation of visual attention is influenced by two control processes, which include a volitional (top-down) network and a stimulus-driven (bottom-up) network (Corbetta & Shulman, Citation2002). Current explanations of the QE have integrated these processes and the QE has been suggested to aid the allocation of attention to relevant cues, while suppressing responses from other stimuli (top-down control) (Vickers, Citation1996). Also, findings from QE training studies suggest that external allocation of attention is responsible for the beneficial effects of the QED on performance. Moore et al. (Citation2012) showed that a QE trained group developed longer QED and better performance compared to a technical trained group during a golf-putting task. The authors suggested that the benefits of the QED derive from better response programming; yet the role of attentional focus (external focus) was also highlighted, which possibly promotes longer QED or is enhanced by the longer QED (Moore et al., Citation2012; Vine & Wilson, Citation2011).
The facilitatory effects of an external focus of attention on motor performance and skill acquisition have been previously described (for a review, see Wulf, Citation2013). It has been suggested that an internal or an external focus of attention disrupts or facilitates automatic control mechanisms, respectively, and that the utilisation of these automatic processes results in decreased attentional demands, smaller movement adjustments and faster learning (Wulf, Shea, & Lewthwaite, Citation2010). Klostermann, Kredel, and Hossner (Citation2014) also examined the links between QED and focus of attention. Their results replicated previous findings in that instructed external focus resulted in better performance and experts exhibited longer QEDs. However, their correlation-based findings did not provide support for a performance-enhancing effect of the longer QED with an external focus of attention. It is worth noting that their instructions varied from previous research in order to exclude the impact of directing the participant's gaze behaviour, and the authors themselves expressed caution when interpreting these results. Klostermann et al. (Citation2014) suggested an alternate explanation for their findings related to an inhibition hypothesis. It follows that the positive effects of longer QED are explained by the need to inhibit alternate movement variables to allow for the effective parameterisation of a single movement. Further research is needed to replicate these findings in order to provide support for this hypothesis.
It is possible that some aspects of external attention allocation are important for QED; however, to date the potential explanations with respect to attention allocation (i.e. movement automaticity or “constrained control theory” and a working memory load hypothesis) have not been experimentally related to QE and more research is needed to probe both phenomena. In addition, given that gaze is typically directed in most QE training protocols, the effects of focus of attention may be influenced by these instructions as suggested by Klostermann et al. (Citation2014). For example, Moore et al. (Citation2012) conducted a QE training study in golf and only included instructions related to gaze control for the QE training group compared to a technical trained group. They found that the QE trained group exhibited more expert-like putting kinematics indirectly associated with an external focus of attention (also see Causer et al., Citation2011). Moore et al. (Citation2012) suggested that future researchers should use more than one group to manipulate focus of attention and further understand the benefits of QE training.
The current QE research is mainly focused on identifying the functional links that explain the effects of performance-enhancing QEDs. To date, the hypothesis based on the information-processing load of motor programming (Williams et al., Citation2002) seems to be a suitable explanation for the QE phenomenon. However, in this review, we present evidence that programming may not be the only mechanisms that affects QED. Furthermore, from the studies included in this review, the effects of QED on performance are not clear-cut, in that longer QEDs do not always correlate with better performance, as in Horn et al. (Citation2012). Unlike other studies where successful and unsuccessful trials have been selected (e.g. Williams et al., Citation2002), excluding trials (e.g. only select the 10 best and 10 worst trials out of a sample and compare the means), Horn et al. performed correlations between performance and QED in novice performers. Their results did not show a QED and perfromance relationship but further suggested that increased familiarity with the task as a result of practice could have strengthened this relationship. This finding suggests that other factors may explain performance facilitation, such as the performer's skill at the task at hand.
There is also great difficulty in obtaining sufficient distribution of performance in highly accurate experts to be able to correlate with QED, which may explain why Reinhoff, Baker, Fischer, Strauss, and Schorer (Citation2012) only observed a correlation between dart throw accuracy and QED in a novice group, in contrast to Horn et al. (Citation2012). Furthermore, comparisons between task difficulty may show worse performance in a highly complex task compared to an easier task, but the former may show longer QED compared to the latter. Whether or not practice effects result in this task-dependant QED compared to the accuracy or skill-related QED remains unclear. The relative contribution of the QED on performance may be addressed through controlled manipulations of QED to identify why, how and when the QED effects manifest during targeting tasks and whether or not training in these tasks results in QED facilitation effects across tasks of varying complexity. We further propose the investigation of the neural mechanisms that are involved in QE using these controlled manipulations to replicate the QE effects obtained in previous field-based research.
Functional mechanisms of QE: psychophysiological evidence
Attention, eye movements and QE
The networks activated during the allocation of spatial attention include the frontal eye fields (FEF), dorsolateral prefrontal cortex (DLPFC) and posterior parietal cortex (PPC); areas that have been individually associated with the control of eye movements. There is evidence supporting the strong links between attention and eye movements (Moore & Fallah, Citation2001, Citation2004; Rizzolatti, Riggio, Dascola, & Umiltá, Citation1987). Furthermore, it has been shown that attention allocated to a fixation point results in a “suppression” (reduced saccade amplitude and velocity) of the oculomotor system (Goldberg, Bushnell, & Bruce, Citation1986), suggesting that actively fixating on a location results in attention allocated to that point and not to peripheral locations. Thus, there seems to be an extensive overlap between attention networks and areas involved in oculomotor control.
The definition of the QE implies the suppression of large eye movements within 1–3 degrees of visual angle and an enhanced ability to fixate on relevant cues and suppress responses to irrelevant stimuli for a more efficient extraction of information. It is important to note that the term “fixation” at 1–3 degrees of visual angle is determined by the technological limitations of current gaze trackers and may encompass other types of eye movements within this definition (e.g. small saccades and pursuit). These saccadic and pursuit eye movements may be used as a favourable strategy for a given task and yet do not fit within normal definitions of fixation. FEF is likely to be involved in QE given the close integration between the frontoparietal network and eye movement (fixation, saccade and pursuit) networks (Corbetta et al., Citation1998). The FEF and the prefrontal cortex also have an important role in eye movement control, needed for maintaining fixation and inhibiting responses (DeSouza, Menon, & Everling, Citation2003). It is conceivable that poorer performance and shorter QED (∼100 ms, see Williams et al., Citation2002) may be associated with distinct activation in these networks compared to when longer QED facilitate performance.
With respect to the “quiet eye” terminology, it is worth noting that the eyes are seldom “quiet” in that small eye movements occur during visual fixation. Fixational eye movements are essential for natural vision (McCamy et al., Citation2012) and include small low velocity drifts as well as high velocity microsaccades (≤1° that occur 1–2 per second); however the precise role of microsaccades in long fixations remains controversial (for a review, see Collewijn & Kowler, Citation2008). A recent study investigating fixational eye movements (drifts and microsaccades) during distinct fading conditions, supports previous notions that microsaccades prevent and correct visual fading during a prolonged fixation (McCamy et al., Citation2012). Microsaccades can be suppressed during fine visual tasks, suggesting they may be modulated by attention and appear functionally related to saccadic intrusions, which are also influenced by the shift of attention (Gowen, Abadi, Poliakoff, Hansen, & Miall, Citation2007). Thus, the amplitude and frequency of eye movements may provide important insights into the links between oculomotor control, visual perception and the allocation of attention in expert performance, which may be explored within the QE.
For example, a low frequency of microsaccades (<1°) in experts compared to novices may suggest enhanced attention to a small target area only and no or very little peripheral visual information pick-up, similar to a needle and thread task. In contrast, higher frequency of microsaccades may suggest that peripheral visual fading is avoided, thus a very accurate fixation may not be required. However, a high frequency of microsaccades may facilitate larger saccadic intrusions that may fall outside of the QE threshold. Finally, it is worth noting that very small amplitude oculomotor noise is unlikely to disrupt vision; however, even moderate head motion requires the involvement of oculomotor compensatory mechanisms, such as the vestibulo-ocular response, optokinetic reflexes, smooth pursuit or saccades. This conclusion again suggests that other gaze behaviours may be occurring in the measured QE period, particularly at the larger 3° threshold.
Motor programming, response selection and online control networks
It is suggested that the ability to maintain attention and ignore/suppress external stimuli is also found to be frontally controlled (FEF and DLPFC, DeSouza et al., Citation2003) and a characteristic observed in expert performers, which aids the programming and execution of a motor response. This selective process is therefore important during the aiming period in a targeting task. Vickers (Citation1992, Citation1996) supported this suppression hypothesis (also see Klostermann et al., Citation2014) after observations of a QE offset during movement initiation, that is, eye movements outside of the QE threshold. Vickers (Citation1992, Citation1996) suggested that this suppression behaviour prevents the interference of the movement plan from online visual feedback. Given that QED effects have been recently associated with more complex tasks such as during variable practice and the presentation of random targets, it may be that feedback and continuous monitoring (afferent) signals, including visual and proprioceptive information, are integrated during the QED. Suppression of eye movements may then be related to distractors or reflect enhanced attention. This finding suggests interactions between top-down and bottom-up control networks (or dorsal and ventral; Corbetta & Shulman, Citation2002) involved in target selection and computations for movement parameterisation during the QE. Thus, the QE may involve a more complex neural network than the hypothesised programming network.
Motor programming and action selection
Finding the optimal balance between the timings for information extraction, motor programming and movement execution is particularly important during time-constrained motor actions, which are encountered in many sporting contexts. The time spent preparing a movement facilitates the development of appropriate actions and often helps minimise errors (Battaglia & Schrater, Citation2007). Expert performers have earlier QE onsets which result in longer QED, suggesting that they are able to find the relevant visual information earlier and programme the movement for longer irrespective of task constraints (Vickers, Citation2009). The first neurophysiological evidence of the QE's role in motor planning was reported by Mann, Coombes, Mousseau, and Janelle (Citation2011), who investigated the Bereitschaftspotential (BP) during the QE period of expert and near-expert golfers. Their results showed increased cortical activation in right-central regions in experts compared to non-experts with an enhanced BP peak and greater negativity, which is suggested to be indicative of movement preparation. Furthermore, evidence from brain imaging and cortical stimulation studies show that the intraparietal sulcus and the premotor cortex (within frontoparietal networks) are involved in the programming of actions (Rushworth, Johansen-Berg, Göbel, & Devlin, Citation2003) and in particular, the pre-supplementary motor area (pre-SMA) has been associated with action selection (Mars, Piekema, Coles, Hulstijn, & Toni, Citation2007). There is evidence of stronger pre-SMA activation in the selection of appropriate actions and stronger inferior frontal cortex activation involved in the suppression of an incorrect response (Forstmann, van den Wildenberg, & Ridderinkhof, Citation2008). Activation of these areas would fit within the programming hypothesis for QE.
The anterior cingulate cortex (ACC) receives input from the prefrontal cortex, also playing a role in action selection (Halsband & Lange, Citation2006), with the pre-SMA. The cingulate cortex has also been associated with emotion regulation during goal-directed actions by manipulating goal outcomes in various tasks (Rolls, Citation2014). Emotion-related decision-making has been suggested to be an important aspect of the QE mechanism. More specifically, a longer QED aids emotional control by enabling the individual to get into a suitable level of arousal to complete the task. This seems important in aiming tasks where a low arousal level is related to better performance and a longer QE may enable the individual to “quieten” the body. For example, Vickers and Williams (Citation2007) tested gaze control of biathletes under low and high anxiety conditions and found that longer QEDs were indirectly related to better performance under pressure situations and coincided with a reduced heart rate and decreased physiological arousal. Furthermore, the QE trained group in Moore et al.’s (Citation2012) study, mentioned above, also showed a deceleration of heart rate prior to the golf putt combined with longer QED and improved putt-kinematics, compared to the technical trained group. However, phasic heart rate did not change during elevated anxiety conditions in either training group, suggesting that this “quieting” of the body may explain some of the benefits of QE training, but do not explain its effects during heightened anxiety situations. The ACC also acts together with the prefrontal cortex to process goal-relevant information (Benn et al., Citation2014) as well as to regulate emotion and peripheral physiology (with ventromedial prefrontal cortex), including heart rate variability and other brain stem modulated autonomic responses (for a review, see Thayer, Åhs, Fredrikson, Sollers, & Wager, Citation2012). Therefore, experimental protocols should focus on separating these processes to identify whether these areas are related to one or more process during the QE.
Online control
Visually guided tasks such as targeting require the accurate visual acquisition of a target, the integration of visual and proprioceptive (afferent) signals for motor programming and response selection prior to the executon of such motor response (Balslev, Miall, & Cole, Citation2007; Gowen & Miall, Citation2006). The frontoparietal networks, particularly the PPC, are suggested to be involved in the integration of signals related to eye and limb movements (Van Donkelaar & Staub, Citation2000). During these processes, internal representations of eyes, limbs and the target are formulated together with predictions of the sensory consequences of the motor response (Wolpert, Ghahramani, & Jordan, Citation1995), and may also engage the cerebellum in this predictive process (Miall & King, Citation2008). Similarly, the allocation of visuospatial attention includes areas within the frontal and parietal lobes (Corbetta et al., Citation1998). It is evident that a tight coupling between visual attention orientation and the processing of the motor components of the task is important for skilled performance (Behan & Wilson, Citation2008).
The involvement of these networks reflects higher order cognitive processing presumably occurring during the QED. Studying the neural networks engaged during a behavioural phenomenon in the sporting field is no easy task. However, researchers have reported promising results, such as the study by Wright, Bishop, Jackson, and Abernethy (Citation2011), in which fMRI was used to investigate the neural mechanisms associated with anticipation in badminton players of varying skill levels. The authors were able to identify distinct levels of activation in task-relevant areas in experts compared to novices and were able to correlate the activation of specific areas to show their influence on performance (also see Heinen, Rowland, Lee, & Wade, Citation2006). Another example of how these networks can be investigated is given by Mann et al.’s (Citation2011) findings of evoked potentials, which suggested distinct QED activation of cortical regions (SMA and M1) in expert golfers. Furthermore, given that the QED effects have been successfully observed under experimentally controlled conditions (Klostermann et al., Citation2013) and during virtual tasks (Behan & Wilson, Citation2008), it seems promising that the mechanisms explaining the QE can be explored in this manner, through manipulations of the proposed processes involved (i.e. programming, attention, inhibition, online control, gaze control, etc.) or by training participants into experts in such tasks. The findings from these studies can then be used to design training protocols and thus determine their validity in a sporting environment.
The relative contributions of inhibition and online feedback integration may be addressed by investigating the QE's temporal components (its onset and offset) during tasks with distinct requirements for these processes. For example, in Klostermann et al.’s (Citation2013) experiments, an early QE onset seemed to be important for accurate throwing, but Vine et al. (Citation2013) suggested it was the late components (offset) that seem to affect performance in golf putting, possibly due to higher demands of online control throughout the movement. These studies suggest that an important aspect of QE is not only its duration but also its timing relative to the movement. In addition, examining these temporal components during the QE may indicate a more efficient allocation of the timings that correspond to information extraction, motor programming and/or movement execution.
In summary, the neural networks and mechanisms responsible for the QE effects are not yet fully understood. Skilled movements have been suggested to involve the dorso-fronto-parietal networks, associated with the allocation of attention to relevant stimuli and the suppression of distractors in the environment (Corbetta & Shulman, Citation2002; Mevorach, Hodsoll, Allen, Shalev, & Humphreys, Citation2010; Mevorach, Humphreys, & Shalev, Citation2006); however, the extent to which these networks are involved in QE needs to be investigated. Currently, researchers have shown that QED is a function of task complexity, a finding that agrees with the current proposed response programming theory of QE. It is further suggested that the QE aids the maintenance of goal-directed (dorsal or top-down attention system) attention control, perhaps via superior gaze control and the effective allocation of visuospatial attention, while suppressing a stimulus-driven attentional system (ventral or bottom-up system) (Vickers, Citation2009). These processes then allow for motor programming to take place during the QED. However, the programming hypothesis may be simplifying a more complex sequence of events that occur during the QE of a targeting task (e.g. integration of online control). Understanding the mechanisms of QE will aid the formulation of training programmes, which are often based on accepted practice rather than on procedures that try to optimise the critical processes involved (Causer et al., Citation2011).
Future directions and recommendations
There is still much to be addressed when attempting to explain the links between gaze and performance and the beneficial effects of a prolonged QED. The formulation of controlled manipulations of QE and the investigation of QE neural networks using neuroimaging and event related potentials (ERP) may shed some light on these correlations (e.g. Mann et al., Citation2011). Furthermore, with current neurophysiological techniques such as transcranial magnetic stimulation (TMS), causal relationships between anatomical regions and behaviour can be established, in addition to determining the extent and timing of their involvement. Neuroimaging reveals activation of specific brain areas during a performed task, but does not determine the functional role of these regions in the observed behaviour. TMS is often used in conjunction with fMRI to determine the links between brain and behaviour by altering the activity of a specific brain region and examining the effects on behaviour. For example, Mevorach et al. (Citation2010) combined fMRI and TMS techniques to investigate the role of different brain regions in attention control during a task that required the suppression of a response to stimuli. They found that TMS applied to the early visual areas led to reduced responses to distractors, which provided evidence for the functional role of these regions in attending or suppressing responses to salient stimuli. Similarly, the effects of QED may be modulated by attention control regions, which can be determined by implementing these techniques.
The combination of TMS and fMRI techniques has been shown to be robust in examining functional mechanisms and neural networks involved in behaviour compared to the less robust ERP measures obtained by electroencephalography (EEG). As such, caution needs to be taken when inferring brain activation of specific areas using EEG methods given the poor spatial resolution; however, EEG is well suited to test the timing of neural activity in a given task. With new technology emerging, it is now possible to measure electrophysiological responses during a golf-putting task (e.g. see Mann et al., Citation2011), although regional information may not be robust using this method. Thus, combining these techniques (e.g. fMRI with EEG and/or TMS) may be of great benefit when trying to explain spatially and timing-dependent networks involved in QE.
The relative contributions of the neural networks for attention control, programming and online control (functions presumably involved in QE) may depend on specific task-goal demands. For example, golf putting requires more dynamic online control functions compared to shooting a target, or shooting a moving target may require a balance between anticipation and inhibition mechanisms afforded by increased attention control. Thus, generalisations of the functional mechanisms involved during the QE and how they influence performance should be characterised based on task demands.
A key issue is that the definition of the QE period is restricted by the sensitivity of the measurement systems that have been employed, which in turn impacts the interpretation of the results. Future research should address the arbitrarily defined threshold for determining QED and systematically evaluate whether performance and skill differences still hold at varying thresholds (e.g. 1 versus 2 or 2 versus 3 degrees of visual angle), thus examining the importance of the amplitude of eye movement during the QE. Researchers should determine whether these eye movement differences are task- or skill-related (i.e. experts may have better oculomotor control) and whether alternate gaze strategies other than fixations may come into play. Thus, caution should be taken when relying on fixation-attention mechanisms to explain the QE phenomenon, since other gaze strategies may be taking place within the QE, and distinct cognitive processes may be encompassed by a single QE definition or threshold. For example, even though there is a great functional overlap between fixation and pursuit; there are also clear distinct neural networks for each system (for a review, see Krauzlis, Citation2005).
High-resolution eye trackers (under 0.1 degrees spatial resolution and sampling ≥500 Hz) have limited use in sport settings, but may be used in laboratory-based tasks in which QED effects on performance are obtained during an aiming or interceptive task. These eye tracking systems better identify differences in oculomotor control and the amount and/or type (pursuit, saccades, microsaccades) of eye movement related to attention/inhibition mechanisms. For example: observed high frequency of microsaccades versus a higher rate of larger saccades would indicate an enhanced fixation ability in the former compared to the latter, which reveals disruptions in visuospatial attention. The use of these high-resolution eye trackers may also address discrepancies between different gaze measuring and analyses techniques for QE. QEDs have been reported using mobile video-based gaze trackers of different resolutions (e.g. 30–60 Hz), video cameras, and electro-oculography (Mann et al., Citation2011). The sensitivity of one technique over another may indicate that some aspects of QE are overlooked; for instance, high variability between participants may hinder the effects of QED on hit or miss trials or QED differences between expert and novice groups. Different techniques may also underestimate or overestimate the duration of the QE, which has implications when attempting to examine the mechanisms involved, particularly when breaking the QED down into its temporal components (onset and offset).
In this review, we also highlighted the need to further examine the links between QE and attention and address whether it is simply gaze control or gaze control coupled with specific allocation of attention that matters in QE. The type of approach reported by Klostermann et al. (Citation2014), in which gaze is not influenced by instructions but QE is manipulated may probe into these attention allocation mechanisms.
Conclusions
The QE appears to be an important measure of perceptual-cognitive expertise. We have highlighted current research that has focused on the functional mechanisms of QE involving direct manipulations and probes of the information programming theory. However, there are strong links between eye movement, attention and programming networks, which may prove to be important during QE and thus, the relative contributions of these still need to be addressed. The importance of understanding the mechanisms of the QE will provide further knowledge of the behavioural and neural mechanisms of performance-enhancing strategies used by expert performers. It will also facilitate the formulation of more efficient training protocols to improve goal-directed movements not only in sports but in other fields, such as surgery and amongst clinical populations such as children with developmental coordination disorders and stroke survivors. This field of research continues to offer a plethora of research opportunities for those interested in vision, cognition and action in sport and other domains of activity.
Disclosure statement
No potential conflict of interest was reported by the authors.
Additional information
Funding
References
- Baker, J., Fischer, L., Strauss, B., & Schorer, J. (2012). Field of vision influences sensory-motor control of skilled and less-skilled dart players. Journal of Sports Science & Medicine, 11(3), 542–550.
- Balslev, D., Miall, R. C., & Cole, J. (2007). Proprioceptive deafferentation slows down the processing of visual hand feedback. Journal of Vision, 7(5), 12.1–7. doi:10.1167/7.5.12
- Battaglia, P. W., & Schrater, P. R. (2007). Humans trade off viewing time and movement duration to improve visuomotor accuracy in a fast reaching task. The Journal of Neuroscience: The Official Journal of the Society for Neuroscience, 27(26), 6984–6994. doi:10.1523/JNEUROSCI.1309-07.2007
- Behan, M., & Wilson, M. (2008). State anxiety and visual attention: The role of the quiet eye period in aiming to a far target. Journal of Sports Sciences, 26(2), 207–215. doi:10.1080/02640410701446919
- Benn, Y., Webb, T. L., Chang, B. P. I., Sun, Y.-H., Wilkinson, I. D., & Farrow, T. F. D. (2014). The neural basis of monitoring goal progress. Frontiers in Human Neuroscience, 8. doi:10.3389/fnhum.2014.00688
- Causer, J., Bennett, S. J., Holmes, P. S., Janelle, C. M., & Williams, A. M. (2010). Quiet eye duration and gun motion in elite shotgun shooting. Medicine and Science in Sports and Exercise, 42(8), 1599–1608. doi:10.1249/MSS.0b013e3181d1b059
- Causer, J., Harvey, A., Snelgrove, R., Arsenault, G., & Vickers, J. N. (2014). Quiet eye training improves surgical knot tying more than traditional technical training: A randomized controlled study. American Journal of Surgery, 208(2), 171–177. doi:10.1016/j.amjsurg.2013.12.042
- Causer, J., Holmes, P. S., & Williams, A. M. (2011). Quiet eye training in a visuomotor control task. Medicine and Science in Sports and Exercise, 43(6), 1042–1049. doi:10.1249/MSS.0b013e3182035de6
- Collewijn, H., & Kowler, E. (2008). The significance of microsaccades for vision and oculomotor control. Journal of Vision, 8(14), 20.1–21. doi:10.1167/8.14.20
- Corbetta, M., Akbudak, E., Conturo, T. E., Snyder, A. Z., Ollinger, J. M., Drury, H. A., … Shulman, G. L. (1998). A common network of functional areas for attention and eye movements. Neuron, 21(4), 761–773. doi: 10.1016/S0896-6273(00)80593-0
- Corbetta, M., & Shulman, G. L. (2002). Control of goal-directed and stimulus-driven attention in the brain. Nature Reviews Neuroscience, 3(3), 215–229. doi:10.1038/nrn755
- De Oliveira, R. F., Huys, R., Oudejans, R. R. D., van de Langenberg, R., & Beek, P. J. (2007). Basketball jump shooting is controlled online by vision. Experimental Psychology, 54(3), 180–186. doi: 10.1027/1618-3169.54.3.180
- DeSouza, J. F. X., Menon, R. S., & Everling, S. (2003). Preparatory set associated with pro-saccades and anti-saccades in humans investigated with event-related FMRI. Journal of Neurophysiology, 89(2), 1016–1023. doi:10.1152/jn.00562.2002
- Forstmann, B. U., van den Wildenberg, W. P. M., & Ridderinkhof, K. R. (2008). Neural mechanisms, temporal dynamics, and individual differences in interference control. Journal of Cognitive Neuroscience, 20(10), 1854–1865. doi:10.1162/jocn.2008.20122
- Goldberg, M. E., Bushnell, M. C., & Bruce, C. J. (1986). The effect of attentive fixation on eye movements evoked by electrical stimulation of the frontal eye fields. Experimental Brain Research, 61(3), 579–584. doi: 10.1007/BF00237584
- Gowen, E., Abadi, R. V., Poliakoff, E., Hansen, P. C., & Miall, R. C. (2007). Modulation of saccadic intrusions by exogenous and endogenous attention. Brain Research, 1141, 154–167. doi:10.1016/j.brainres.2007.01.047
- Gowen, E., & Miall, R. C. (2006). Eye-hand interactions in tracing and drawing tasks. Human Movement Science, 25(4–5), 568–585. doi:10.1016/j.humov.2006.06.005
- Halsband, U., & Lange, R. K. (2006). Motor learning in man: A review of functional and clinical studies. Journal of Physiology-Paris, 99(4–6), 414–424. doi:10.1016/j.jphysparis.2006.03.007
- Heinen, S. J., Rowland, J., Lee, B.-T., & Wade, A. R. (2006). An oculomotor decision process revealed by functional magnetic resonance imaging. The Journal of Neuroscience: The Official Journal of the Society for Neuroscience, 26(52), 13515–13522. doi:10.1523/JNEUROSCI.4243-06.2006
- Horn, R. R., Okumura, M. S., Alexander, M. G. F., Gardin, F. A., & Sylvester, C. T. (2012). Quiet eye duration is responsive to variability of practice and to the axis of target changes. Research Quarterly for Exercise and Sport, 83(2), 204–211. doi:10.1080/02701367.2012.10599851
- Klostermann, A., Kredel, R., & Hossner, E. J. (2013). The “quiet eye” and motor performance: Task demands matter!. Journal of Experimental Psychology: Human Perception and Performance, 39(5), 1270–1278. doi:10.1037/a0031499
- Klostermann, A., Kredel, R., & Hossner, E. J. (2014). On the interaction of attentional focus and gaze: The quiet eye inhibits focus-related performance decrements. Journal of Sport & Exercise Psychology, 36(4), 392–400. doi:10.1123/jsep.2013-0273
- Krauzlis, R. J. (2005). The control of voluntary eye movements: New perspectives. The Neuroscientist, 11(2), 124–137. doi:10.1177/1073858404271196
- Mann, D. T. Y., Coombes, S. A., Mousseau, M. B., & Janelle, C. M. (2011). Quiet eye and the Bereitschaftspotential: Visuomotor mechanisms of expert motor performance. Cognitive Processing, 12(3), 223–234. doi:10.1007/s10339-011-0398-8
- Mars, R. B., Piekema, C., Coles, M. G. H., Hulstijn, W., & Toni, I. (2007). On the programming and reprogramming of actions. Cerebral Cortex, 17(12), 2972–2979. doi:10.1093/cercor/bhm022
- McCamy, M. B., Otero-Millan, J., Macknik, S. L., Yang, Y., Troncoso, X. G., Baer, S. M., … Martinez-Conde, S. (2012). Microsaccadic efficacy and contribution to foveal and peripheral vision. The Journal of Neuroscience: The Official Journal of the Society for Neuroscience, 32(27), 9194–9204. doi:10.1523/JNEUROSCI.0515-12.2012
- Mevorach, C., Hodsoll, J., Allen, H., Shalev, L., & Humphreys, G. (2010). Ignoring the elephant in the room: A neural circuit to downregulate salience. The Journal of Neuroscience: The Official Journal of the Society for Neuroscience, 30(17), 6072–6079. doi:10.1523/JNEUROSCI.0241-10.2010
- Mevorach, C., Humphreys, G. W., & Shalev, L. (2006). Opposite biases in salience-based selection for the left and right posterior parietal cortex. Nature Neuroscience, 9(6), 740–742. doi:10.1038/nn1709
- Miall, R. C., & King, D. (2008). State estimation in the cerebellum. The Cerebellum, 7(4), 572–576. doi:10.1007/s12311-008-0072-6
- Miles, C. A. L., Wood, G., Vine, S. J., Vickers, J. N., & Wilson, M. R. (2015). Quiet eye training facilitates visuomotor coordination in children with developmental coordination disorder. Research in Developmental Disabilities, 40, 31–41. doi:10.1016/j.ridd.2015.01.005
- Moore, L. J., Vine, S. J., Cooke, A., Ring, C., & Wilson, M. R. (2012). Quiet eye training expedites motor learning and aids performance under heightened anxiety: The roles of response programming and external attention. Psychophysiology, 49(7), 1005–1015. doi:10.1111/j.1469-8986.2012.01379.x
- Moore, T., & Fallah, M. (2001). Control of eye movements and spatial attention. Proceedings of the National Academy of Sciences of the United States of America, 98(3), 1273–1276. doi:10.1073/pnas.021549498
- Moore, T., & Fallah, M. (2004). Microstimulation of the frontal eye field and its effects on covert spatial attention. Journal of Neurophysiology, 91(1), 152–162. doi:10.1152/jn.00741.2002
- Piras, A., & Vickers, J. N. (2011). The effect of fixation transitions on quiet eye duration and performance in the soccer penalty kick: Instep versus inside kicks. Cognitive Processing, 12(3), 245–255. doi:10.1007/s10339-011-0406-z
- Rienhoff, R., Hopwood, M. J., Fischer, L., Strauss, B., Baker, J., & Schorer, J. (2013). Transfer of motor and perceptual skills from basketball to darts. Frontiers in Psychology, 4, 593. doi:10.3389/fpsyg.2013.00593
- Rizzolatti, G., Riggio, L., Dascola, I., & Umiltá, C. (1987). Reorienting attention across the horizontal and vertical meridians: Evidence in favor of a premotor theory of attention. Neuropsychologia, 25(1A), 31–40. doi: 10.1016/0028-3932(87)90041-8
- Rodrigues, S. T., Vickers, J. N., & Williams, A. M. (2002). Head, eye and arm coordination in table tennis. Journal of Sports Sciences, 20(3), 187–200. doi:10.1080/026404102317284754
- Rolls, E. T. (2014). Emotion and decision-making explained: A précis. Cortex; A Journal Devoted to the Study of the Nervous System and Behavior, 59, 185–193. doi:10.1016/j.cortex.2014.01.020
- Rushworth, M. F. S., Johansen-Berg, H., Göbel, S. M., & Devlin, J. T. (2003). The left parietal and premotor cortices: Motor attention and selection. NeuroImage, 20(Suppl 1), S89–S100. doi: 10.1016/j.neuroimage.2003.09.011
- Thayer, J. F., Åhs, F., Fredrikson, M., Sollers, J. J., & Wager, T. D. (2012). A meta-analysis of heart rate variability and neuroimaging studies: Implications for heart rate variability as a marker of stress and health. Neuroscience & Biobehavioral Reviews, 36(2), 747–756. doi:10.1016/j.neubiorev.2011.11.009
- Van Donkelaar, P., & Staub, J. (2000). Eye-hand coordination to visual versus remembered targets. Experimental Brain Research, 133(3), 414–418. doi: 10.1007/s002210000422
- Vickers, J. N. (1992). Gaze control in putting. Perception, 21(1), 117–132. doi: 10.1068/p210117
- Vickers, J. N. (1996). Visual control when aiming at a far target. Journal of Experimental Psychology. Human Perception and Performance, 22(2), 342–354. doi: 10.1037/0096-1523.22.2.342
- Vickers, J. N. (2009). Advances in coupling perception and action: The quiet eye as a bidirectional link between gaze, attention, and action. Progress in Brain Research, 174, 279–288. doi:10.1016/S0079-6123(09)01322-3
- Vickers, J. N. (2011). Mind over muscle: The role of gaze control, spatial cognition, and the quiet eye in motor expertise. Cognitive Processing, 12(3), 219–222. doi:10.1007/s10339-011-0411-2
- Vickers, J. N., & Williams, A. M. (2007). Performing under pressure: The effects of physiological arousal, cognitive anxiety, and gaze control in biathlon. Journal of Motor Behavior, 39(5), 381–394. doi:10.3200/JMBR.39.5.381-394
- Vine, S. J., Lee, D., Moore, L. J., & Wilson, M. R. (2013). Quiet eye and choking: Online control breaks down at the point of performance failure. Medicine and Science in Sports and Exercise, 45(10), 1988–1994. doi:10.1249/MSS.0b013e31829406c7
- Vine, S. J., Moore, L. J., & Wilson, M. R. (2014). Quiet eye training: The acquisition, refinement and resilient performance of targeting skills. European Journal of Sport Science, 14(Suppl 1), S235–S242. doi:10.1080/17461391.2012.683815
- Vine, S. J., & Wilson, M. R. (2011). The influence of quiet eye training and pressure on attention and visuo-motor control. Acta Psychologica, 136(3), 340–346. doi:10.1016/j.actpsy.2010.12.008
- Williams, A. M., Singer, R. N., & Frehlich, S. G. (2002). Quiet eye duration, expertise, and task complexity in near and far aiming tasks. Journal of Motor Behavior, 34(2), 197–207. doi:10.1080/00222890209601941
- Wolpert, D. M., Ghahramani, Z., & Jordan, M. I. (1995). An internal model for sensorimotor integration. Science, 269(5232), 1880–1882. doi: 10.1126/science.7569931
- Wright, M. J., Bishop, D. T., Jackson, R. C., & Abernethy, B. (2011). Cortical fMRI activation to opponents’ body kinematics in sport-related anticipation: Expert-novice differences with normal and point-light video. Neuroscience Letters, 500(3), 216–221. doi:10.1016/j.neulet.2011.06.045
- Wulf, G. (2013). Attentional focus and motor learning: A review of 15 years. International Review of Sport and Exercise Psychology, 6(1), 77–104. doi:10.1080/1750984X.2012.723728
- Wulf, G., Shea, C., & Lewthwaite, R. (2010). Motor skill learning and performance: A review of influential factors. Medical Education, 44(1), 75–84. doi:10.1111/j.1365-2923.2009.03421.x