Abstract
This study investigated whether commercially available compression garments (COMP) exerting a moderate level of pressure and/or neuromuscular electrical stimulation (NMES) accelerate recovery following a cross-country sprint skiing competition compared with a control group (CON) consisting of active recovery only. Twenty-one senior (12 males, 9 females) and 11 junior (6 males, 5 females) Swedish national team skiers performed an outdoor sprint skiing competition involving four sprints lasting ∼3–4 min. Before the competition, skiers were matched by sex and skiing level (senior versus junior) and randomly assigned to COMP (n = 11), NMES (n = 11) or CON (n = 10). Creatine kinase (CK), urea, countermovement jump (CMJ) height, and perceived muscle pain were measured before and 8, 20, 44 and 68 h after competition. Neither COMP nor NMES promoted the recovery of blood biomarkers, CMJ or perceived pain post-competition compared with CON (all P > .05). When grouping all 32 participants, urea and perceived muscle pain increased from baseline, peaking at 8 h (standardised mean difference (SMD), [95% confidence intervals (CIs)]): 2.8 [2.3, 3.2]) and 44 h (odds ratio [95% CI]: 3.3 [2.1, 5.1]) post-competition, respectively. Additionally, CMJ was lower than baseline 44 and 68 h post-competition in both males and females (P < .05). CK increased from baseline in males, peaking at 44 h (SMD: 1.4 [−0.4, 0.9]), but was decreased in females at 20 h post-competition (SMD: −0.8 [−1.4, −0.2]). In conclusion, cross-country sprint skiing induced symptoms of exercise-induced muscle damage peaking 8–44 h post-competition. However, neither COMP nor NMES promoted physiological or perceptual recovery compared with CON.
Highlights
Methods to accelerate post-exercise recovery after a cross-country sprint skiing competition have not been investigated.
Sprint skiing induces symptoms of exercise induced muscle damage that peak 8-44 h post-competition.
Wearing compression garments or applying neuromuscular electrical stimulation during post-competition recovery does not accelerate physiological or perceptual recovery.
Introduction
Cross-country sprint skiing involves an initial time-trial qualification round (a prologue), followed by three head-to-head knockout rounds (quarter-finals, semi-finals and final). Therefore, a cross-country sprint skiing competition involves four consecutive races on the same day for the six successful finalists, completed over the same course, which is typically 1.2–1.6 km and lasts ∼3–4 min per race. Both aerobic and anaerobic energy systems are highly taxed during sprint skiing, with ∼18–26% of the required energy produced from anaerobic pathways, and the remainder derived from aerobic pathways (Andersson et al., Citation2010; Losnegard & Hallén, Citation2014). Whilst there are approximately two-to-three hours of recovery between the end of the prologue and the start of quarter-finals, skiers will typically have 15–30 min of recovery between their quarter-final, semi-final and final, which may not be sufficient time to completely clear metabolic products before a subsequent race (Gejl et al., Citation2017). Since most World Cup skiers compete in both sprint and distance events, which are often scheduled on consecutive days (International Ski Federation (FIS), Citation2017), the extent of recovery following one event is likely to affect performance in the subsequent event.
Maximal exercise performance involving high-velocity, eccentric muscle actions induce changes in physiological and perceptual biomarkers associated with exercise-induced muscle damage (EIMD), such as increases in creatine kinase (CK), urea, muscle swelling and pain perception and decreased joint range of motion (Debenham, Travers, Campbell, & Allison, Citation2016). Delayed onset muscle soreness secondary to EIMD develops within ∼24–72 h after exercise (Nosaka & Clarkson, Citation1995) and is associated with decreased maximal exercise performance during this period (Twist & Eston, Citation2009). Muscle soreness and CK levels have been shown to peak immediately and 24 h after a 50 km cross-country race, respectively (Takashima, Ishii, Takizawa, Yamaguchi, & Nosaka, Citation2007), but evidence of EIMD symptoms following a sprint skiing competition is lacking. Stöggl, Kampel, Mueller, and Lindinger (Citation2010) suggested that the presence and effectiveness of a stretch-shortening cycle, involving eccentric muscle action, might be elevated during sprint skiing at high velocities, especially for the G3 (V2) “double push” sub-technique. Therefore, EIMD may be likely to develop following a sprint skiing competition, possibly impairing performance in events scheduled one-to-two days afterwards. Whilst some studies have investigated strategies to enhance recovery between races within a sprint skiing competition (Losnegard, Andersen, Spencer, & Hallén, Citation2015; Sperlich, Born, Zinner, Hauser, & Holmberg, Citation2014), none have investigated strategies to facilitate recovery after a sprint skiing competition.
Compression garments (COMP) (Hill, Howatson, van Someren, Leeder, & Pedlar, Citation2014) and neuromuscular electrical stimulation (NMES) (Malone, Blake, & Caulfield, Citation2014) are two modalities used to promote post-exercise recovery. Mechanistically, COMP is purported to enhance recovery by creating an external pressure gradient that reduces the space available for muscle swelling (Ko, Lerner, Klose, & Cosimi, Citation1998) and increase blood flow to skeletal muscle via a vasoactive pump mechanism, in turn promoting blood metabolite clearance (Tucker et al., Citation2010). In comparison, NMES emits a sub-tetanic electrical pulse to the skeletal muscle that enhances local blood flow, which may also assist blood metabolite clearance, hypothetically accelerating post-exercise recovery kinetics (Malone et al., Citation2014). Whilst COMP has been reported to benefit (Hill et al., Citation2014; Jakeman, Byrne, & Eston, Citation2010), or have no effect on (Sperlich et al., Citation2010) the recovery kinetics of several physiological, perceptual and performance parameters, there is currently limited evidence to support using NMES to promote post-exercise recovery (Babault, Cometti, Maffiuletti, & Deley, Citation2011; Maffiuletti, Citation2010; Malone et al., Citation2014).
No studies to date have investigated the effectiveness of COMP and/or NMES on post-exercise recovery following a cross-country sprint skiing competition in a field-based context. The current study therefore aimed to determine whether or not COMP and NMES accelerated the recovery of blood biomarkers of muscle damage (CK and urea), countermovement jump (CMJ) height and perceived muscle pain before and 8, 20, 44 and 68 h after a cross-country sprint skiing competition in a cohort of elite senior and junior cross-country skiers. It was hypothesised that COMP and NMES would speed up the recovery of both physiological and perceptual parameters compared with CON.
Material and methods
Participants
Thirty-two cross-country skiers gave their written informed consent to participate in this study. Three participants were aged <18 years thus written consent was provided by their parents. All participants were members of the Swedish national senior (12 males, 9 females) or junior (6 males, 5 females) teams for the 2016–2017 season (). Ethical approval was granted for the study by the regional ethics board in Umeå, Sweden (2016-443-31M) and study protocols were conducted in accordance with the 1964 Declaration of Helsinki.
Table 1. Mean ± standard deviation descriptive characteristics of the senior and junior female and male cross-country skiers.
Study overview
The study followed a randomised, parallel groups design with repeated measures. Blood parameters (CK and urea), CMJ and perceived muscle pain were measured at baseline in the morning one day before the competition. Skiers were then randomised into one of three recovery intervention groups, matched for sex and experience level (i.e. senior versus junior): COMP (n = 11), NMES (n = 11) or a control group (CON, n = 10). All skiers performed an active recovery immediately after the sprint skiing competition, followed by either COMP or NMES. Skiers in the CON received no recovery intervention following the active recovery and all skiers were instructed to perform no additional, self-selected recovery methods. The recovery parameters were reassessed 8, 20, 44 and 68 h after the sprint skiing competition. Throughout the study, skiers maintained their normal dietary habits to replicate the practices they would employ during an international standard, sprint skiing competition. The sprint competition was performed in conjunction with a pre-season training camp and typical training plans during the data collection period, as set by the national team coaches, are available as supplementary material.
Cross-country sprint skiing competition
The cross-country sprint skiing competition was organised by the Swedish Ski Federation at an international-standard ski stadium situated at an altitude of 700 m. The competition was designed to simulate World Cup competition conditions and was performed on snow using the freestyle technique. The ski track was prepared in the morning before the event by a team of experts to optimise racing conditions. The temperature of the snow was 0°C and the ambient temperature was ∼1°C. All skiers used their own racing skis, which were selected for prevailing snow conditions and prepared by a team of professional ski technicians, using a similar stone grind and glide-wax (Briko Maplus Med Base).
The race distance was 1570 m for both males and females and consisted of a prologue conducted as an individual time-trial, followed by quarter-finals, semi-finals and finals. Skiers were instructed to warm up and compete exactly as they would during a regular sprint skiing competition. Unlike real-world sprint racing, all skiers completed all four races of the competition (i.e. the prologue, quarter-final, semi-final and final), regardless of where they placed in each race. However, rest periods between races and the organisation of progression through the heats simulated real-world racing conditions. Heart rate (HR) was recorded continuously from the start of the warm-up to the end of the cool-down. Finger-prick capillary blood samples were collected into 60 µL glass microcapillary tubes within 3 min following each sprint race and peak lactate concentration in whole blood (B[La−]peak) was analysed within 60 min of sampling using an automated blood lactate analyser (Biosen, EFK Diagnostics, Germany). As per real-world competition, where access to ski tracks may be limited, skiers immediately performed a 15-min active cool down consisting of jogging at 60–65% of maximal heart rate (HRmax) after completing their final ski race.
Whole-body compression garments
Skiers in the whole-body compression group wore commercially available, moderate-pressure upper- and lower-body COMP (Craft SportswearTM, Borås, Sweden) matched for body size for ∼17 h following the post-competition active recovery. Upper-body COMP consisted of a long-sleeved shirt applying pressure to the trunk and arms. Lower-body COMP covered the thigh and leg up to the ankle. The compressive force applied by the garments was measured in duplicate by the manufacturer using a medical stocking testing device (MST MK V, Swisslastic AG, St. Gallen, Switzerland) on a sample garment at seven segments along the lower limb: B (ankle): 14.6 ± 0.1 mmHg, B1 (achilles tendon): 13.6 ± 1.4 mmHg, C (calf): 13.7 ± 1.3 mmHg, D (tibial tuberosity): 7.6 ± 1.1 mmHg, E (patella) 8.3 ± 2.3 mmHg, F (mid-thigh): 5.3 ± 1.1 mmHg and G (5 cm below crotch): 4.2 ± 2.8 mmHg. These pressures are similar to those measured by Hill et al. (Citation2017) at the calf (14.8 ± 2.1 mmHg) and thigh (8.1 ± 1.3 mmHg). The garments were worn from ∼12:00 following the competition until ∼08:00 the next morning, only being removed during the evening training session on the day of the competition (∼16:00–18:00). Two participants (one male and one female) removed their garments during the night, after wearing them for ∼12–14 h, due to overheating.
Neuromuscular electrical stimulation
For skiers in the NMES group, trained researchers applied NMES to the thigh muscles using a commercially available device (Compex Sport, CA, USA) within 3–4 h after the sprint skiing competition in accordance with the manufacturers’ instructions. Specifically, three electrode patches were placed at the centre of the muscle belly on the rectus femoris, vastus medialis and vastus lateralis. Subsequently, the device was set to “recovery massage” mode, and a standardised electrical stimulation protocol was applied for 20 min, comprising 7 min at 9 Hz, 7 min at 7 Hz and 6 min at 5 Hz. The pulse frequency was strong enough to elicit muscle contraction but not to elicit pain or discomfort. No participants reported any pain or discomfort during or following treatment with NMES and the recovery protocol was completed before the commencement of the skiers’ second daily training session.
Recovery parameters
Participants rated their perceived muscle pain upon arrival at the indoor test location using a 10-point pain scale (0 = no pain at all, 10 = unbearable pain) following a full-depth, body-weight squat. Finger-prick blood samples were then collected into 60 µL lithium heparin microcuvettes and whole blood was analysed immediately after each test session for CK and urea concentrations using an automated bench-top analyser (Reflotron Sprint, Roche Diagnostics, USA). The coefficient of variation for the repeatability (within-series imprecision) and reproducibility (day-to-day imprecision) of the CK assay was 3.5% and 3.7%, respectively, and 2.3% and 3.5% for the urea assay, respectively.
To measure neuromuscular fatigue of the lower limbs, participants performed two CMJs with at least 30 s of passive recovery between each jump. To isolate the lower limbs, skiers stood with their hands on their hips before squatting to a depth of 90°, then jumped as high as possible immediately following the squat with the hands remaining on the hips throughout the jump (i.e. no arm swing was permitted). An experienced researcher demonstrated the jumping protocol for each individual and skiers were re-tested if they were unable to correctly perform the jumping protocol. An automated infrared contact mat was used to measure jump height (Muscle Lab Jump, Ergotest, Norway).
Statistical analysis
Statistical analyses were performed using the nlme package (Pinheiro, Bates, DebRoy, & Sarkar, Citation2014) of the R statistical programming language (R Core Team, Citation2017). The changes in CK, urea and CMJ following the sprint skiing competition were analysed using linear mixed models with fixed effects for time (five levels: pre-competition and 8, 20, 44 and 68 h post-competition) and condition (three levels: CON, COMP, NMES) and a random effect for athlete ID. CK and urea were log-transformed before analysis, and back-transformed to allow the results to be expressed as a percentage change from the reference levels (time: pre-competition; condition: CON). Where necessary, heteroscedasticity was corrected by modelling an appropriate residual variance structure.
Separate models were fit to examine the time × condition interaction effect for CK and CMJ in males and females, respectively, owing to the known sex difference for these variables. When there was no significant interaction effect(s), higher order effects were averaged over levels of the remaining factor(s). The change in perceived muscle pain for each recovery condition was analysed using an ordinal regression, with the results expressed as odds ratios (ORs). The standardised mean difference (SMD) was calculated by dividing the difference in means by the within-subject standard deviation (SD) derived from the random effects and interpreted using the following qualitative descriptors: trivial (<0.2), small (0.2–0.6), moderate (0.6–1.2), large (1.2–2.0), very large (2.0–4.0) and extremely large (>4.0) (Hopkins, Citation2014). The imprecision of model parameter estimates and effect sizes is expressed by 95% confidence intervals (CIs).
Results
Performance, HR and blood lactate responses
Descriptive statistics of race times, HR and B[La−]peak responses for each race of the sprint competition are presented in for the senior and junior female and male cross-country skiers.
Table 2. Mean ± standard deviation race time, heart rate and post-race peak blood lactate concentration in senior and junior female and male skiers.
Recovery parameters
Responses for each recovery parameter at baseline, 20, 44 and 68 h post-competition are presented in . No time × condition effect was observed for urea or perceived muscle pain, or in males or females for CK or CMJ (all P > .05), meaning that all treatment groups showed a similar pattern of recovery over time. As such, the interaction term was removed and separate models were fitted to investigate the change in each recovery parameter following the sprint skiing competition.
Table 3. Mean ± standard deviation responses for recovery parameters in the control (CON, n = 10), compression (COMP, n = 11) and neuromuscular electrical stimulation (NMES, n = 11) group at baseline, 8, 20, 44 and 68 h after a cross-country sprint skiing competition.
The changes in recovery parameters after the sprint skiing competition are presented in for all three recovery groups combined (CON, COMP, NMES).
Figure 1. Creatine kinase (A), urea (B), countermovement jump (C) and perceived muscle pain (D) values for the three recovery groups combined (CON, COMP and NMES) at baseline, and 8, 20, 44 and 68 h after a cross-country sprint skiing competition. Time points included within the shaded regions are significantly different from baseline (P < .05). Significantly different from baseline in males (a, b) and females (c) (P < .05).
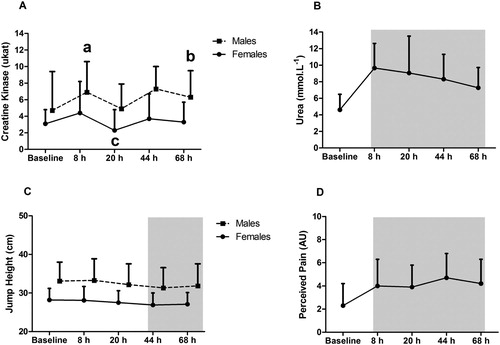
In males, CK showed a moderate to large increase from baseline levels at 8 h (122% [15, 329], SMD: 1.0 [0.2, 1.7]), 44 (40% [−29, 177]; SMD: 1.4 [−0.4, 0.9]) and 68 h (120% [22, 295]; SMD: 1.0 [0.3, 1.5]) post-competition, respectively. There was a moderate decrease in CMJ from baseline levels at 44 h (SMD: −0.8 [−2.0, −0.2]) and 68 h (SMD: −0.6 [−1.8, 0.0]) post-competition.
In females, CK levels moderately decreased from baseline levels at 20 h post-competition (−46% [−67, −12]; SMD: −0.8 [−1.4, −0.2]), whereas a large decrease in CMJ from baseline levels occurred at 44 h (SMD: −1.7 [−2.6, −0.2]) and 68 h (SMD: −1.5 [−2.3, −0.2]) post-competition.
Overall, urea levels were higher than baseline at all post-competition time points (all P < .05), peaking at 8 h (SMD: 2.8 [2.3, 3.2], very large). Skiers were 2.3 [1.4, 3.9], 2.3 [1.4, 3.7], 3.3 [2.1, 5.1]) and 2.6 [1.6, 4.4] times more likely to rate perceived muscle pain higher at 8, 20, 44 and 68 h post-competition, respectively.
Discussion
Contrary to the study hypothesis, COMP and NMES applied following a cross-country sprint skiing competition did not accelerate post-competition recovery kinetics compared with CON. Furthermore, the sprint skiing competition induced symptoms consistent with EIMD, such as increased blood urea and CK concentrations, perceived muscle pain and a decreased CMJ. Moreover, there were clear sex differences in the magnitude of the post-competition CK and CMJ responses, respectively.
Symptoms of EIMD
To our knowledge, this is the first study to investigate the influence of a sprint skiing competition on the symptoms of EIMD in elite cross-country skiers. Commonly reported symptoms of EIMD include elevated blood CK and urea levels, peaking 24–72 h post-exercise, increased skeletal muscle pain perception (Peake, Neubauer, Della Gatta, & Nosaka, Citation2017) and reduced CMJ performance (Byrne & Eston, Citation2002). In the current study, increases in CK (in males), urea and perceived muscle pain were observed, in conjunction with a decrease in CMJ, with some symptoms persisting for 44–68 h after competition. The severity of the muscle damage symptoms observed in the current study is lower than those reported in untrained (Jakeman et al., Citation2010) and well-trained (Byrne & Eston, Citation2002) individuals undertaking different exercise modalities. For example, the peak rise in CK observed in the present study was ∼70% above baseline and occurred 20 h post-competition, which is substantially lower than the 300–700% post-exercise increase in CK reported for untrained individuals (Byrne & Eston, Citation2002). According to the repeated bout effect, the symptoms of EIMD are less severe in athletes who are accustomed to an exercise task or movement pattern (Hyldahl, Chen, & Nosaka, Citation2017). The high volumes of aerobic exercise, combined with consistent high-intensity interval and strength training performed by elite cross-country skiers (Sandbakk & Holmberg, Citation2017), may have therefore protected the skiers in the current study against muscle-fibre damage and the subsequent leakage of CK into the blood following the sprint skiing competition. Furthermore, cross-country skiers may be less susceptible to EIMD since a sprint skiing competition likely involves less eccentric muscle actions performed at high mechanical loads compared with other exercise modalities such as running or weightlifting, in turn leading to less severe myofibril damage. However, further research is necessary to elucidate the exact relationship between eccentric muscle actions and the severity of EIMD following a sprint skiing competition. Practically, complete recovery from EIMD following a sprint skiing race may not be achieved if skiers are required to compete again within 48 h. Since the physiological consequences of EIMD include reduced skeletal muscle force production, impaired glycogen resynthesis and increased pain perception (Clarkson & Hubal, Citation2002), which may impair maximal exercise performance, these findings highlight an important challenge for elite cross-country skiers who typically compete in multiple races within a 48-h period (International Ski Federation (FIS), Citation2017).
Compression garments
Contrary to the study hypothesis, COMP worn after the sprint skiing competition did not facilitate improved recovery of the blood biomarkers, perceived muscle pain or CMJ compared with CON. These findings are consistent with experimental studies reporting that COMP does not reduce blood CK levels during the post-exercise recovery period, although these earlier studies did lead to reduced perceived muscle pain (Duffield et al., Citation2008; Duffield, Cannon, & King, Citation2010). Furthermore, a meta-analysis by Hill et al. (Citation2014) concluded that wearing COMP after exercise showed moderate effect sizes for reducing blood CK levels, perceived muscle soreness and restoring muscle power compared with a control group. The external pressure applied by the commercial COMP used in the current study (i.e. 13.6 mmHg at the calf and 14.6 mmHg at the ankle for ∼15–24 h post-exercise) may have been insufficient to alter hemodynamics and thus promote the removal of metabolites after exercise. Furthermore, whilst COMP is thought to enhance limb skeletal muscle blood flow and venous return, Sperlich, Born, Kaskinoro, Kalliokoski, and Laaksonen (Citation2013) observed that COMP applied to the thigh (37 mmHg external pressure, which is far greater than the ∼5 mmHg applied in the current study) instead decreased blood flow to the rectus femoris and bicep femoris muscles following submaximal cycling exercise. In a later study, Sperlich et al. (Citation2014) noted that upper-body COMP did not alter cardiovascular parameters such as stroke volume, venous return or oxygen uptake after 3 × 3 min double-poling sprints on a ski ergometer. Collectively, these studies suggest that commercially available COMP may have a limited ability to alter skeletal muscle blood flow and may explain why the garments did not speed the recovery kinetics of blood biomarkers compared with CON after the cross-country sprint skiing competition in the current study.
Neuromuscular electrical stimulation
The interest in investigating the efficacy of NMES as a recovery intervention in the current study stemmed from anecdotal reports from the medical support staff that skiers were using these devices to enhance their recovery from competitions. The findings showed that applying NMES to the quadriceps muscles did not accelerate physiological, perceptual or performance recovery kinetics compared with CON following the sprint skiing competition. These results support the conclusion that NMES does not promote metabolite recovery compared to a passive control group (Maffiuletti, Citation2010; Malone et al., Citation2014). Several factors are known to influence the effectiveness of NMES as a recovery method, such as the location of the electrode placement, inter-individual differences between motor and pain perception thresholds and limb anatomy (i.e. the muscle cross-sectional area and levels of subcutaneous adipose tissue) (Babault et al., Citation2011). For example, NMES applied to the gastrocnemius muscle belly for 15–20 min following a Yo-Yo intermittent recovery test has been shown to increase bicarbonate levels, improve blood lactate clearance and maintain blood pH more effectively than an active cool down involving 15 min of stationary cycling at 40% maximal oxygen consumption in female handball players (Bieuzen, Borne, Toussaint, & Hausswirth, Citation2014). However, although a similar NMES protocol applied to the gastrocnemius maintained performance in a subsequent 30-s all-out rowing ergometer test scheduled 24 h after an initial bout, it was no more effective than a passive recovery at maintaining CMJ performance or decreasing blood CK levels during post-exercise recovery (Bieuzen, Pournot, Roulland, & Hausswirth, Citation2012). Thus, stronger pulse-wave frequencies than those used in the current study may be required to increase local blood flow and augment the removal of blood-borne metabolites in elite cross-country skiers with large skeletal muscle masses.
Strengths and limitations
To preserve the ecological validity of this study, no attempt was made to control the skiers’ dietary habits. However, due to the importance of nutrition in the recovery process (Hausswirth & Le Meur, Citation2011) all athletes were supported by experienced sports nutritionists throughout the study, to facilitate optimal intakes of macro- and micronutrients. Regarding intervention durations for COMP and NMES, these were not matched in the present study for two reasons: firstly, applying NMES to skeletal muscles for long periods after exercise is potentially harmful (Nosaka & Clarkson, Citation1995), and secondly, in practice, athletes would likely use NMES for a shorter duration than they would use COMP. Thus, the dissimilar recovery durations between CON, COMP and NMES are justified from a practical perspective.
The sprint competition in the current study was organised in conjunction with a pre-season training camp for the national cross-country ski teams, whereby athletes followed training schedules devised by their coaches that focused on requirements for that phase of the season. This resulted in training sessions before and after the sprint competition potentially affecting recovery measures made throughout the study. There was a systematic attempt to reduce any group bias by matching the number of senior and juniors athletes in each intervention group (CON, COMP and NMES), since the different age groups followed different training schedules. Although speculative, it is possible that the higher training volumes undertaken by the senior groups during the post-competition phase could have slowed the recovery kinetics of CK and perceived pain, meaning that these parameters remained elevated above pre-competition values for longer in the senior, compared with junior, athletes.
Whilst low participant numbers are often stated as a limitation in applied sport studies, it is likely unrealistic to strive for more than 32 national team athletes in a well-controlled, field-based scientific experiment, as were recruited to this study. Therefore, it may be wise in future applied studies with elite athletes to limit the number of intervention groups to two, in order to maintain statistical power. The small sample size for each recovery group in the present study (i.e. 10–11 skiers per group) and large intra- and inter-individual variability in the blood biomarkers in response to the sprint skiing competition makes it difficult to conclude that neither recovery intervention promoted skiers’ recovery kinetics compared with CON. Indeed, given the variable individual responses to each recovery intervention, both COMP and NMES may benefit some individuals when applied in the correct context. Future studies could investigate the effects of different compressive forces in the compression garments, as well as different exposure durations and pulse-wave frequencies of the NMES. In addition, baseline and follow-up measures could include a ski-specific performance task, to provide a practically relevant indicator of recovery modality efficacy.
Practical applications
The results of the current study suggest that using moderate-pressure, commercially available COMP for ∼17 h post-exercise or NMES for 20 min post-exercise does not promote recovery following a sprint skiing competition. However, it remains unknown whether alternative COMP or NMES protocols, or combining these two recovery modalities, could improve post-competition recovery. A positive aspect of using both COMP and NMES is their ease of transport and application, which may provide a practical advantage over recovery methods such as massage and cold-water immersion. Finally, since sleep is currently considered the best form of recovery (Juliff, Halson, & Peiffer, Citation2015), further research is necessary to investigate the typical sleep hygiene practices of cross-country skiers during World Cup events.
Conclusion
A cross-country sprint skiing competition induced symptoms of EIMD such as elevated blood CK and urea levels and perceived muscle pain, peaking at 8–44 h post-competition. Furthermore, CMJ was decreased following the sprint skiing competition. However, neither COMP nor NMES accelerated the recovery kinetics of physiological, perceptual or performance parameters compared with CON. Further research is necessary to investigate the influence of individualised strategies to promote recovery following a sprint skiing competition and their influence on skiing performance during events held on consecutive days.
Supplementary_Material.docx
Download MS Word (19.7 KB)Acknowledgement
The authors would like to thank the skiers and coaches involved in this study and the Swedish Skiing Federation for their cooperation and enthusiasm during the project.
Disclosure statement
No potential conflict of interest was reported by the authors.
References
- Andersson, E., Supej, M., Sandbakk, Ø, Sperlich, B., Stöggl, T., & Holmberg, H.-C. (2010). Analysis of sprint cross-country skiing using a differential global navigation satellite system. European Journal of Applied Physiology, 110(3), 585–595. doi: 10.1007/s00421-010-1535-2
- Babault, N., Cometti, C., Maffiuletti, N. A., & Deley, G. (2011). Does electrical stimulation enhance post-exercise performance recovery? European Journal of Applied Physiology, 111(10), 2501–2507. doi: 10.1007/s00421-011-2117-7
- Bieuzen, F., Borne, R., Toussaint, J.-F., & Hausswirth, C. (2014). Positive effect of specific low-frequency electrical stimulation during short-term recovery on subsequent high-intensity exercise. Applied Physiology, Nutrition, and Metabolism, 39(2), 202–210. doi: 10.1139/apnm-2013-0185
- Bieuzen, F., Pournot, H., Roulland, R., & Hausswirth, C. (2012). Recovery after high-intensity intermittent exercise in elite soccer players using VEINOPLUS sport technology for blood-flow stimulation. Journal of Athletic Training, 47(5), 498–506. doi: 10.4085/1062-6050-47.4.02
- Byrne, C., & Eston, R. (2002). The effect of exercise-induced muscle damage on isometric and dynamic knee extensor strength and vertical jump performance. Journal of Sports Sciences, 20(5), 417–425. doi: 10.1080/026404102317366672
- Clarkson, P. M., & Hubal, M. J. (2002). Exercise-induced muscle damage in humans. American Journal of Physical Medicine & Rehabilitation, 81(11), S52–S69. doi: 10.1097/00002060-200211001-00007
- Debenham, J., Travers, M., Campbell, A., & Allison, G. (2016). Eccentric fatigue modulates stretch-shortening cycle effectiveness – a possible role in lower limb overuse injuries. International Journal of Sports Medicine, 37, 50–55.
- Duffield, R., Cannon, J., & King, M. (2010). The effects of compression garments on recovery of muscle performance following high-intensity sprint and plyometric exercise. Journal of Science and Medicine in Sport, 13(1), 136–140. doi: 10.1016/j.jsams.2008.10.006
- Duffield, R., Edge, J., Merrells, R., Hawke, E., Barnes, M., Simcock, D., & Gill, N. (2008). The effects of compression garments on intermittent exercise performance and recovery on consecutive days. International Journal of Sports Physiology and Performance, 3(4), 454–468. doi: 10.1123/ijspp.3.4.454
- Gejl, K. D., Ørtenblad, N., Andersson, E., Plomgaard, P., Holmberg, H. C., & Nielsen, J. (2017). Local depletion of glycogen with supramaximal exercise in human skeletal muscle fibres. The Journal of Physiology, 595(9), 2809–2821. doi: 10.1113/JP273109
- Hausswirth, C., & Le Meur, Y. (2011). Physiological and nutritional aspects of post-exercise recovery. Sports Medicine, 41(10), 861–882. doi: 10.2165/11593180-000000000-00000
- Hill, J., Howatson, G., van Someren, K., Gaze, D., Legg, H., Lineham, J., & Pedlar, C. (2017). The effects of compression-garment pressure on recovery after strenuous exercise. International Journal of Sports Physiology and Performance, 12(8), 1078–1084. doi: 10.1123/ijspp.2016-0380
- Hill, J., Howatson, G., van Someren, K. A., Leeder, J., & Pedlar, C. (2014). Compression garments and recovery from exercise-induced muscle damage: A meta-analysis. British Journal of Sports Medicine, 48(18), 1340–1346. doi: 10.1136/bjsports-2013-092456
- Hopkins, W. (2014). A scale of magnitudes for effect statistics. Retrieved from http://sportsci.org/resource/stats/effectmag.html.
- Hyldahl, R. D., Chen, T. C., & Nosaka, K. (2017). Mechanisms and mediators of the skeletal muscle repeated bout effect. Exercise and Sport Sciences Reviews, 45(1), 24–33. doi: 10.1249/JES.0000000000000095
- International Ski Federation (FIS). (2017). World cup rules and results. Retrieved from http://www.fis-ski.com.
- Jakeman, J. R., Byrne, C., & Eston, R. G. (2010). Lower limb compression garment improves recovery from exercise-induced muscle damage in young, active females. European Journal of Applied Physiology, 109(6), 1137–1144. doi: 10.1007/s00421-010-1464-0
- Juliff, L. E., Halson, S. L., & Peiffer, J. J. (2015). Understanding sleep disturbance in athletes prior to important competitions. Journal of Science and Medicine in Sport, 18(1), 13–18. doi: 10.1016/j.jsams.2014.02.007
- Ko, D. S., Lerner, R., Klose, G., & Cosimi, A. B. (1998). Effective treatment of lymphedema of the extremities. Archives of Surgery, 133(4), 452–458. doi: 10.1001/archsurg.133.4.452
- Losnegard, T., Andersen, M., Spencer, M., & Hallén, J. (2015). Effects of active versus passive recovery in sprint cross-country skiing. International Journal of Sports Physiology and Performance, 10(5), 630–635. doi: 10.1123/ijspp.2014-0218
- Losnegard, T., & Hallén, J. (2014). Physiological differences between sprint-and distance-specialized cross-country skiers. International Journal of Sports Physiology and Performance, 9(1), 25–31. doi: 10.1123/ijspp.2013-0066
- Maffiuletti, N. A. (2010). Physiological and methodological considerations for the use of neuromuscular electrical stimulation. European Journal of Applied Physiology, 110(2), 223–234. doi: 10.1007/s00421-010-1502-y
- Malone, J. K., Blake, C., & Caulfield, B. M. (2014). Neuromuscular electrical stimulation during recovery from exercise: A systematic review. Journal of Strength & Conditioning Research, 28(9), 2478–2506. doi: 10.1519/JSC.0000000000000426
- Nosaka, K., & Clarkson, P. M. (1995). Muscle damage following repeated bouts of high force eccentric exercise. Medicine & Science in Sports & Exercise, 27(9), 1263–1269. doi: 10.1249/00005768-199509000-00005
- Peake, J. M., Neubauer, O., Della Gatta, P. A., & Nosaka, K. (2017). Muscle damage and inflammation during recovery from exercise. Journal of Applied Physiology, 122(3), 559–570. doi: 10.1152/japplphysiol.00971.2016
- Pinheiro, J., Bates, D., DebRoy, S., & Sarkar, D. (2014). R Core Team (2014) nlme: linear and nonlinear mixed effects models. R package version 3.1-117. Retrieved from http://CRAN.R-project.org/package=nlme.
- R Core Team. (2017). R: A language and environment for statistical computing. R Foundation for Statistical Computing, 55.
- Sandbakk, Ø, & Holmberg, H.-C. (2017). Physiological capacity and training routines of elite cross-country skiers: Approaching the upper limits of human endurance. International Journal of Sports Physiology and Performance, 12(8), 1003–1011. doi: 10.1123/ijspp.2016-0749
- Sperlich, B., Born, D.-P., Kaskinoro, K., Kalliokoski, K. K., & Laaksonen, M. S. (2013). Squeezing the muscle: Compression clothing and muscle metabolism during recovery from high intensity exercise. PLoS One, 8(4), e60923. doi: 10.1371/journal.pone.0060923
- Sperlich, B., Born, D.-P., Zinner, C., Hauser, A., & Holmberg, H.-C. (2014). Does upper-body compression improve 3×3-min double-poling sprint performance? International Journal of Sports Physiology and Performance, 9(1), 48–57. doi: 10.1123/ijspp.2013-0137
- Sperlich, B., Haegele, M., Achtzehn, S., Linville, J., Holmberg, H.-C., & Mester, J. (2010). Different types of compression clothing do not increase sub-maximal and maximal endurance performance in well-trained athletes. Journal of Sports Sciences, 28(6), 609–614. doi: 10.1080/02640410903582768
- Stöggl, T., Kampel, W., Mueller, E., & Lindinger, S. (2010). Double-push skating versus V2 and V1 skating on uphill terrain in cross-country skiing. Medicine & Science in Sports & Exercise, 42(1), 187–196. doi: 10.1249/MSS.0b013e3181ac9748
- Takashima, W., Ishii, K., Takizawa, K., Yamaguchi, T., & Nosaka, K. (2007). Muscle damage and soreness following a 50-km cross-country ski race. European Journal of Sport Science, 7(1), 27–33. doi: 10.1080/17461390701197833
- Tucker, A., Maass, A., Bain, D., Chen, L., Azzam, M., Dawson, H., & Johnston, A. (2010). Augmentation of venous, arterial and microvascular blood supply in the leg by isometric neuromuscular stimulation via the peroneal nerve. International Journal of Angiology, 19(01), e31–e37. doi: 10.1055/s-0031-1278361
- Twist, C., & Eston, R. G. (2009). The effect of exercise-induced muscle damage on perceived exertion and cycling endurance performance. European Journal of Applied Physiology, 105(4), 559–567. doi: 10.1007/s00421-008-0935-z