ABSTRACT
The aim of the study is to evaluate whether exercise interventions are associated with improved glycaemic control in children and adolescents with type 1 diabetes mellitus (T1DM), and to examine its relationship with the characteristics of the intervention (i.e. type, intensity, length, and duration of the sessions). Eligible criteria were randomised controlled trials of youth aged 6–18 years with T1DM, participating in an exercise-based intervention where glycaemic control is measured (i.e. glycated haemoglobin [HbA1c]). Pooled effect sizes (Hedges’g) were calculated using random-effects inverse-variance analyses. Fourteen studies enrolling 509 patients were analysed. Effect size was expressed as Hedges’ g to correct for possible small sample bias. Overall, HbA1c levels in the exercise group (g = –0.38 95% confidence interval [CI], –0.66 to –0.11; mean difference [MD] = –0.62%) were reduced compared with the control group. Concurrent training (g = –0.63 95%CI, –1.05 to –0.21), high-intensity exercise (g = –0.43 95%CI, –0.83 to –0.03), interventions ≥24 weeks (g = –0.92 95%CI, –1.44 to –0.40), and sessions ≥60 minutes (g = –0.71 95%CI, –1.05 to –0.08) showed larger changes (MD = –0.66% to 1.30%). In conclusion, our study suggests that programmes longer than 24 weeks with at least 60 min/session of high-intensity concurrent exercise may serve as a supportive therapy to metabolic control in youth with T1DM.
Highlights
Exercise training has a moderate effect on the reduction of glycated haemoglobin (HbA1c) and insulin dose per day in youths with type 1 diabetes.
Exercise training moderately increases cardiorespiratory fitness youths with type 1 diabetes.
Reductions in HbA1c are stronger with high-intensity and concurrent training (i.e. aerobic and strength) interventions, and longer programmes.
1. Introduction
Type 1 diabetes mellitus (T1DM) is a chronic inflammatory autoimmune disease that destroys the insulin-producing β-cells of the pancreas, leading to absolute insulin deficiency (Atkinson & Eisenbarth, Citation2001). It mainly affects the paediatric age group, and its incidence is increasing worldwide. Poorly controlled T1DM elevates the risk of both acute and chronic complications because of the lifetime exposure to fluctuating blood glucose levels (McCarthy, Funk, & Grey, Citation2016), resulting in approximately 7 years of lower life expectancy (Soedamah-Muthu et al., Citation2006).
Poor glycaemic control increases the risk of having acute complications such as episodes of hypoglycaemia and ketoacidosis, and chronic complications including microvascular events (nephropathy, retinopathy, and neuropathy), and cardiometabolic disorders (Association, Citation2020), causing damage to the growth and development of children and adolescents. The American Diabetes Association (ADA) recommends the use of glycated haemoglobin (HbA1c) for the evaluation of glycaemic control in T1DM, with a target of <7% for all paediatric age groups, aiming at the prevention of future complications (Association, Citation2021; DiMeglio et al., Citation2018). Time in range (within target range, below range, above range) is also appropriate and a useful clinical target that complements HbA1c (Battelino et al., Citation2019).
Physical activity and exercise are associated with better glycaemic control in youth with T1DM (Colberg et al., Citation2016). Physical activity can play a role in managing blood glucose levels in this setting, as it positively affects insulin sensitivity, with the additional benefit of being a key component of health maintenance and optimal physical/psychological development (Piercy et al., Citation2018). Accordingly, several international societies such as The International Society for Paediatric and Adolescent Diabetes (ISPAD) (Adolfsson et al., Citation2018) and the ADA recommend that children and youth perform at least 60 min of moderate- to high-intensity aerobic activity daily, with vigorous muscle-strengthening and bone-strengthening activities at least 3 days per week (C-level evidence: supportive evidence from poorly controlled or uncontrolled studies) (Association, Citation2020); however, the majority of youth do not meet current physical activity guidelines (Liese, Ma, Maahs, & Trilk, Citation2013), which represents a considerable challenge for this population. This is especially relevant since physical inactivity and prolonged periods of sitting increase gradually with age and are linked to high HbA1c concentrations in youth with T1DM (Galler, Lindau, Ernert, Thalemann, & Raile, Citation2011).
However, several studies have reported inconsistent findings on the clinical benefits of exercise on physiological and biochemical outcomes in children and adolescents with T1DM. This might be because many factors influence an individual’s glycaemic response to exercise, including the type, intensity, and duration of the activity (Tonoli et al., Citation2012), the dose of insulin and the person’s stress/anxiety levels (Association, Citation2019). For example, a systematic review and meta-analysis published by Quirk et al. (Quirk, Blake, Tennyson, Randell, & Glazebrook, Citation2014) suggested that exercise programmes have the potential to decrease the risk for cardiovascular diseases and improve glycaemic control in children and adolescents with T1DM. By contrast, the systematic reviews and meta-analyses of Ostman et al. (Ostman, Jewiss, King, & Smart, Citation2018) and Kennedy et al. (Kennedy et al., Citation2013) provided evidence for no glycaemic benefits from exercise interventions in youth with T1DM. The methodological approach taken in these three meta-analyses introduced a potential risk of bias because non-randomised controlled trials were included, and we must be cautious with their findings. Also, all of the above-mentioned meta-analyses used the traditional random-effects model of Dersimonian and Laird, while the recent inverse variance heterogeneity and quality effects models of IntHout et al. (Inthout, Ioannidis, & Borm, Citation2014) have been shown to be more robust with respect to coverage probabilities.
There also remains uncertainty whether the effects of different types of training (Tonoli et al., Citation2012) and intensity have different outcomes for chronic glycaemic control in paediatric populations with T1DM (Riddell et al., Citation2017). In view of the above, the objective of the present study was to evaluate whether exercise interventions are associated with improved glycaemic control in children and adolescents with T1DM, and to examine whether this was related to the characteristics of the intervention (i.e. type, intensity, length, and duration of the sessions).
2. Materials and methods
2.1. Protocol and registration
The study was conducted and reported according to PRISMA guidelines and recommendations (Liberati et al., Citation2009). The protocol was registered in the PROSPERO International Prospective Register of Systematic Reviews (ID: CRD42020219366). The entire process from literature selection to data extraction was performed independently by two authors (AG-H & YE). Any disagreements were resolved through consultation with a third researcher (RR-V).
2.2. Eligibility criteria
To be eligible for inclusion in the present review, randomised controlled trials (RCT) needed to meet the following criteria: (i) children and adolescents diagnosed with T1DM aged 6–18 years (mean age); (ii) RCT that included a comparison group (i.e. control group) also diagnosed with T1DM but without a structured exercise plan or calorie restriction; (iii) supervised exercise interventions (e.g. aerobic, strength/resistance, or a combination of both [concurrent training]); and (iv) primary outcome of interest was glycaemic control estimated using the evaluation of HbA1c, but also other secondary outcomes such as insulin dosage per day, hypoglycaemic episodes and cardiorespiratory fitness estimated as maximum oxygen consumption (VO2max or peak). In the case of duplicate studies, the latest version was included.
2.3. Information sources and search
The strategy was designed around the PICOS question format – Do children and adolescents diagnosed with T1DM (Population), who participate in an exercise-based programme (Intervention) compared to those who do not (Comparator), differ in glycaemic control (HbA1c levels, Outcome) in RCTs (Study design)?
Ovid, MEDLINE, EMBASE, Cochrane Controlled Trials Registry, and SPORTDiscus electronic databases were searched for studies from inception to March 10, 2021. In addition, a grey literature search of abstracts and conference proceedings from the Meeting of the International Society for Pediatric and Adolescent Diabetes, ADA, the European Diabetes Congress, the World Congress on Diabetes, Endocrinology & Metabolism, and the International Diabetes Federation Congress in the last five years was also carried out. The MeSH terms used were: [“diabetes mellitus, type 1” OR “type I” OR “type 1 diabetes” OR “juvenile diabetes” OR “insulin-dependent diabetes”] AND [“physical activity” OR “exercise” OR “exercises” OR “sport” OR “sports” OR “fitness” OR “training” OR “exercise therapy”]. Also, searches were limited to youth aged 5–18 years and RCTs. Cross-referencing was also used by examining the reference lists of articles that met the inclusion criteria. A librarian was consulted to supervise the quality of the search. The search strategy is described in detail in Supplementary data 1.
2.4. Study selection and data collection process
Two authors independently extracted the following data from the identified RCTs: characteristics of the studies (i.e. authors name, sample size, country, duration of intervention and publication year); characteristics of participants, age and sex; (iv) characteristics of the exercise intervention (i.e. frequency, duration, adherence and type [i.e. aerobic, strength/resistance or (concurrent)]); and (v) results from primary (HbA1c) and secondary outcomes (insulin dosage per day, hypoglycaemic episodes, and cardiorespiratory fitness). Missing data from the selected studies were requested from the authors of the original manuscripts.
2.5. Risk of bias in individual studies
Methodological quality was appraised using the Physiotherapy Evidence Database (PEDro) criteria (www.pedro.org.au) (Maher, Sherrington, Herbert, Moseley, & Elkins, Citation2003). This tool is an 11-item scale designed for rating the methodological quality of RCT. Two authors scored each study independently, with disagreements being resolved by consensus with a third investigator.
2.6. Synthesis of results
All analyses were carried out with the STATA software (v16.1; StataCorp, College Station, TX, USA) and conducted using the random-effects inverse-variance model with the Hartung-Knapp-Sidik-Jonkman adjustment. Changes in primary and secondary outcomes for RCT were calculated by subtracting change differences between the exercise and control groups, using the pooled standard deviation (SD) of change in both groups. If change scores SD were not available, they were calculated from 95% confidence intervals (CI) for either change outcome or exercise training effect differences as well as pre-SD and post-SD values (Follmann, Elliott, Suh, & Cutler, Citation1992). When the data were shown only by figures, webplotdigitizer 4.3 software was used to extract them. Effect size was expressed as Hedges’ g to correct for possible small sample bias (org JH http://www.cochrane handbook (Citation2008)). However, we also determined mean differences whenever parameters in all studies were expressed through the same unit of measurement (e.g. HbA1c as percentage or cardiorespiratory fitness as ml·kg·min−1).
Heterogeneity across RCT was calculated using the inconsistency index (I2), derived from the Cochran Q statistic (Higgins, Thompson, Deeks, & Bmj, Citation2003). Larger values for I2 suggest a larger degree of inconsistency. Small-study effects and publication biases were examined using the Doi plot and Luis Furuya-Kanamori (LFK) index. Both test have been shown to be superior to the traditional funnel plot and Egger’s regression intercept test (Furuya-Kanamori, Barendregt, & Doi, Citation2018). No asymmetry, minor asymmetry or major asymmetry was considered with values of –1, between –1 and –2, and >–2, respectively (Furuya-Kanamori et al., Citation2018).
Random-effects meta-regression analysis using the Hartung-Knapp-Sidik-Jonkman adjustment was performed to evaluate whether results were different by duration of the interventions (months). The association between changes in HbA1c and insulin dosage per day was also tested.
Finally, a subgroup analysis was conducted according to duration of the intervention (<20 weeks or ≥20 weeks), duration of session (<60 minutes or ≥60 minutes), type of exercise training (i.e. aerobic, strength, and concurrent) and intensity of training using the categories proposed by the American College of Sports Medicine (light, moderate, moderate-to-high, and high intensity) (Riebe, Ehrman, Liguori, Magal, & Medicine, Citation2018).
3. Results
3.1. Study selection
A total of 14 RCT were included in the meta-analysis (Campaigne, Gilliam, Spencer, Lampman, & Schork, Citation1984; Campaigne, Landt, & Mellies, Citation1985; D’hooge et al., Citation2011; Gusso et al., Citation2017; Heyman et al., Citation2007; Huttunen et al., Citation1989; Landt, Campaigne, James, & Sperling, Citation1985; Nazari, Shabani, & Dalili, Citation2020; Petschnig, Wagner, Robubi, & Baron, Citation2020; Roberts, Jones, & Fournier, Citation2002; Salem, Aboelasrar, Elbarbary, Elhilaly, & Refaat, Citation2010; Stratton, Wilson, Endres, & Goldstein, Citation1987; Tomar, Hamdan, & Al-Qahtani, Citation2014; Tunar et al., Citation2012). The PRISMA flow diagram is shown in . Excluded studies and reasons for exclusion are provided in Supplementary data 2.
3.2. Study characteristics
A summary of the 14 studies included in this study is listed in . All children and adolescents were recruited in hospitals or in diabetes clinics. The included RCT involved a total of 509 participants (exercise groups n = 318), ranging from 14 (Campaigne et al., Citation1984; Landt et al., Citation1985) to 196 (Salem et al., Citation2010) participants per trial. All studies included boys and girls, with the exception of one study that included only girls (Heyman et al., Citation2007), and one study only of boys (Tomar et al., Citation2014). Duration of T1DM was of at least 24 weeks in all participants.
Table 1. Summary of included studies.
Studies included aerobic (n = 6), strength/resistance (n = 1), concurrent training (n = 6), and Pilates alone (n = 1), with an average duration of 12 weeks, ranging from eight (Stratton et al., Citation1987) to 32 (Petschnig et al., Citation2020) weeks. Seven RCT included the adherence to the training programmes (>62%). Exercise intensity of the exercise training was mainly high (Campaigne et al., Citation1984; Gusso et al., Citation2017; Heyman et al., Citation2007; Landt et al., Citation1985; Roberts et al., Citation2002; Salem et al., Citation2010; Stratton et al., Citation1987).
Regarding daily insulin doses, three studies reported units per day (Campaigne et al., Citation1984; Gusso et al., Citation2017; Tomar et al., Citation2014) and three reported units per kilograms per day (D’hooge et al., Citation2011; Salem et al., Citation2010; Tunar et al., Citation2012). For this reason, we did not calculate the mean differences for this parameter.
Finally, all RCT used maximal tests on a cycle ergometer (Campaigne et al., Citation1984; Gusso et al., Citation2017; Huttunen et al., Citation1989; Landt et al., Citation1985) or a treadmill (Campaigne et al., Citation1985) with spirometry to determine oxygen consumption by VO2max or VO2peak (i.e. cardiorespiratory fitness), which was reported as mL·kg−1·min−1.
3.3. Risk of bias within studies
All 14 studies fulfilled item 2 (proper randomisation), item 10 (between-group analysis) and item 11 (point estimates and variability) of the PEDro criteria. By contrast, given the nature of the exercise-based interventions, blinding of patients and therapists was not possible, so no study fulfilled items 5 and 6, and only two studies evaluated outcomes with blinded assessors (item 7) (D’hooge et al., Citation2011; Gusso et al., Citation2017). Overall, the mean score was 4.8/10 (Supplementary Table 1).
3.4. Synthesis of results
Glycated haemoglobin (HbA1c). Compared with the control group, structured exercise interventions were associated with significant moderate reductions in HbA1c (Hedge’s g = –0.38 95% CI, –0.66 to –0.11, p = 0.009; I2 = 55.41%) (), corresponding to a mean difference of –0.62% (Supplementary Figure 1). Cochran’s Q statistic for statistical heterogeneity was 31.40 (p = 0.005). Asymmetry suggestive of small-study effects was observed for HbA1c (LFK index = 2.56) (Supplementary Figure 2).
Figure 2. Forest plot showing estimates of the size of change in HbA1c, insulin dosage, and cardiorespiratory fitness after a physical exercise intervention.
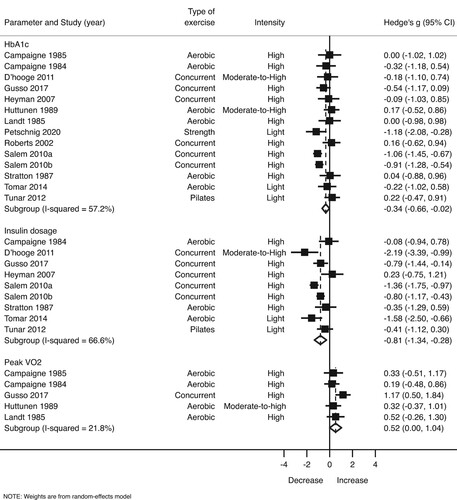
Subgroup analysis reveals that concurrent training (Hedge’s g = –0.63 95% CI, –1.05 to –0.21, p = 0.011; Q = 12.18; I2 = 50.73%; mean difference = –0.74%), high-intensity exercise (Hedge’s g = –0.43 95% CI, –0.83 to –0.02, p = 0.037; Q = 17.62; I2 = 54.6%; mean difference = –0.66%), interventions ≥24 weeks (g = –0.92 95% CI, –1.44 to –0.40; Q = 10.36; I2 = 80.7%; mean difference = –1.30%), and sessions ≥60 minutes (g = –0.71 95% CI, –1.05 to –0.08; Q = 8.60; I2 = 30.2%; mean difference = –0.86%) led greater reductions in HbA1c ().
Table 2. Exercise effects on HbA1c, insulin dosage and cardiorespiratory fitness according to training programme characteristics.
Meta-regression analyses revealed significant effects of duration of the intervention (β = –0.067; p < 0.001) and sessions (β = –0.032; p = 0.012) on the effect size estimate, but not for frequency per week (β = 0.047; p = 0.762) (Supplementary Figure 3). Sensitivity analyses indicated no modifications in the results for after removing one study at a time, ranging from –0.44 (95% CI, –0.71 to –0.17) to –0.31 (95% CI, –0.58 to –0.05).
Insulin dosage per day. Exercise training resulted in higher decreases of insulin dosage per day (Hedgés g = –0.81 95% CI, –1.34 to –0.28, p = 0.008; I2 = 66.6%) (). Cochran’s Q statistic for statistical heterogeneity was 23.99 (p = 0.002). No asymmetry suggestive of small-study effects was observed (LKF index = 0.35) (Supplementary Figure 4).
In the same manner as described above, high-intensity exercise programmes (Hedge’s g = –0.65 95% CI, –1.25 to –0.06, p = 0.037; Q = 15.42; I2 = 67.6%), mainly combined training (Hedge’s g = –0.96 95% CI, –1.88 to –0.04, p = 0.045; Q = 14.61; I2 = 72.6%), and sessions of at least 60 minutes (Hedge’s g = –1.04 95% CI, –1.78 to –0.29, p = 0.016; Q = 16.05; I2 = 68.9%) resulted in a stronger reduction in insulin dosage ().
Meta-regression analyses revealed no significant effects of duration of the intervention (β = –0.023; p = 0.570), session number (β = –0.035; p = 0.062), or frequency per week (β = 0.014; p = 0.963) on the effect size estimate (Supplementary Figure 5).
With each study deleted from the model once, nonoverlapping 95% CIs were observed across all deletions, ranging from –0.90 (95% CI, –1.12 to –0.69) to –1.01 (95% CI, –1.27 to –0.76).
Cardiorespiratory fitness. We also observed a moderate increase of cardiorespiratory fitness among youth in the exercise groups (Hedge’s g = 0.52 95% CI, 0.00–1.04, p = 0.049; I2 = 21.8%) () corresponding to a mean difference of 3.94 mL·kg−1·min−1 (Supplementary Figure 6). Cochran’s Q statistic for statistical heterogeneity was 5.12 (p = 0.275). No asymmetry suggestive of small-study effects was observed (LKF index = 1.13) (Supplementary Figure 7).
Meta-regression analyses revealed significant effects of duration of the session (β = 0.033; p = 0.037) on the effect size estimate, but not for duration of the intervention (β = 0.094; p = 0.076) or frequency (β = 0.224; p = 0.260) (Supplementary Figure 8).
Finally, with each study deleted from the model once, nonoverlapping 95% CIs were observed across all deletions, ranging from 0.51 (95% CI, 0.05–0.98) to 0.61 (95% CI, 0.20–1.03). However, significant changes disappeared after deletion of the results of Gusso et al. (Gusso et al., Citation2017).
Hypoglycaemic episodes. Seven studies did not report this information. Three studies reported no hypoglycaemic episodes during exercise among their participants (Campaigne et al., Citation1985; Landt et al., Citation1985; Petschnig et al., Citation2020). Campaigne et al. (Campaigne et al., Citation1984) reported that one child experienced hypoglycaemia during an exercise session. D’hooge et al. (D’hooge et al., Citation2011) reported a median number of hypoglycaemic levels of three in the training group. Salem et al. (Salem et al., Citation2010) showed that after exercise programmes, the frequency of hypoglycaemic events was not statistically different between the two study arms.
4. Discussion
We evaluated studies from the past 40 years investigating the long-term effect of exercise on several glycaemic control parameters in youth with T1DM. The major findings of this meta-analysis are that structured exercise training has a moderate effect on the reduction of HbA1c (%) levels and insulin dose per day, and moderately increases of cardiorespiratory fitness in children and adolescents with T1DM. Other important findings are that these reductions are stronger with high-intensity and concurrent training interventions. Finally, the exercise programme duration (i.e. minutes of session longer than 60 and length of the intervention longer than 24 weeks) has a moderating role on the reduction of HbA1c levels.
It is a well-known fact the role of exercise in the metabolic control of children and adolescents with T1DM (Riddell et al., Citation2017). A previously published literature review reported benefits of exercise on glycaemic control in youth with T1DM, but highlighted inconsistencies across studies (Quirk et al., Citation2014). Indeed, several meta-analyses found no improvement in glycaemic control in this population after exercise training (Kennedy et al., Citation2013; Ostman et al., Citation2018; Tonoli et al., Citation2012). By contrast, our meta-analytic design, which used strict inclusion criteria and a more robust statistical approach (Doi, Furuya-Kanamori, Thalib, & Barendregt, Citation2017), reveals a significant reduction in HbA1c levels (–0.62%), which can be considered clinically relevant for young people with T1DM (Rosenbauer et al., Citation2012). Our findings reinforce the importance of exercise in the clinical management of diabetes to delay or reduce the risk of microvascular complications and cardiovascular disease (Maahs et al., Citation2014; McCarthy et al., Citation2016). A 1% decrease in HbA1c reflects a 14% reduction in the risk of acute myocardial infarction, a 37% reduction in the risk of microvascular complications, and a 21% reduction in the risk of T1DM-related deaths (Evans, Citation1998). However, the inconsistent findings between meta-analyses could reflect the heterogeneity of studies used for analyses, at the level of both inclusion criteria and study design, and also the lack of measurement and/or reporting of changes in insulin dosage and diet in included studies (Quirk et al., Citation2014).
In accordance with our present findings, studies on T1DM consistently demonstrate that exercise is associated with reduced insulin requirements (Chimen et al., Citation2012), which may reduce patient burden (Rosenbauer et al., Citation2012). Kennedy et al. (Kennedy et al., Citation2013) also noted in their systematic review and meta-analysis that a longer duration of exercise intervention showed a trend towards a reduction in HbA1c levels. Similarly, Chimen et al. (Chimen et al., Citation2012) reported that it is possible that the reductions in insulin dosage per day could mask any glycaemic improvement, as measured by HbA1c; however, our results showed no associations between both changes (Supplementary Figure 9, β = 0.184; p = 0.550). Several RCTs included in the present study (D’hooge et al., Citation2011; Salem et al., Citation2010; Tomar et al., Citation2014) suggest that children and adolescents might have reduced their insulin doses or ingested more carbohydrates than usual to avoid hypoglycaemia. It has been suggested that HbA1c may not be a sensitive indicator of glycaemic control (Kennedy et al., Citation2013), and that improvement in glycaemic variability may not be reflected in this parameter. In this line, continuous or intermittent monitoring of glucose in interstitial fluid could help individuals to learn their own response to different types of exercise programmes, and it might be a more appropriate marker than HbA1c to explore the exercise-induced beneficial effect (Chetty et al., Citation2019). However it is well known that exercise improves insulin sensitivity in trained muscles (Kristiansen, Gade, Wojtaszewski, Kiens, & Richter, Citation2000), such that the insulin needs may be lowered. Thus, the results of investigated studies are open to debate.
Impairment in the ability to perform exercise in people with T1DM may be related to the level of glycaemic control. For example, a large study of healthy and adolescents/young adults shows that the responses to cardiopulmonary exercise testing are impaired in individuals with T1DM independent of HbA1c compared with control participants without T1DM (Mccarthy et al., Citation2020). Likewise, Poortmans et al. (Poortmans, Saerens, Edelman, Vertongen, & Dorchy, Citation1986) and Huttunen et al. (Huttunen et al., Citation1984) both reported that cardiorespiratory fitness is inversely related to the level of metabolic control, as measured by HbA1c. Consistent with the literature (Chimen et al., Citation2012), the present meta-analysis found that cardiorespiratory fitness, assessed with indirect calorimetry, increased about 3.94 mL·kg−1·min−1 with exercise programmes. Several of the analysed studies measured this parameter using the PWC170 (Physical Work Capacity) and the “Aerobic Power Index” submaximal test, and found positive significant effects in the exercise groups (D’hooge et al., Citation2011; Heyman et al., Citation2007; Roberts et al., Citation2002). Because children and adolescents with T1DM may present with early cardiovascular complications, especially those with poor glycaemic control (Maahs et al., Citation2014), and are less fit than matched individuals without diabetes (Wu et al., Citation2021) the importance of cardiorespiratory fitness (Garciá-Hermoso, Ramírez-Vélez, Garciá-Alonso, Alonso-Martínez, & Izquierdo, Citation2020) and the important role of physical activity (Piercy et al., Citation2018) from early years should be emphasised. It is unclear, however, whether the reduced work capacity in youth with T1DM is a result of poorer oxygenation of muscle (Ditzel & Standl, Citation1975) or lower muscle capillarisation (Kivelä et al., Citation2006), or whether poorer metabolic control is a function of lower amounts of habitual physical activity (Robertson, Riddell, Guinhouya, Adolfsson, & Hanas, Citation2014).
Long-term exercise-induced glycaemic benefits are based on the continuous effect of each successive bout of exercise. To date, no studies have provided guidance on the duration, type or intensity of exercise that will confer the greatest benefit in this population. In this respect, the published evidence indicates that exercise programmes must be sufficiently long enough to achieve a reduction in HbA1c (Ostman et al., Citation2018; Tonoli et al., Citation2012), findings confirmed by our meta-analysis. Specifically, our results show larger reductions on HbA1c with programmes of at least 24 weeks (−1.30%) and sessions larger than 60 minutes (−0.86%). Kennedy et al. (Kennedy et al., Citation2013) suggest that the benefits to HbA1c are associated with programmes longer than 12–24 weeks. Since the life span of the human red blood cell is about 127 days (Shemin & Rittenberg, Citation1946), this timeframe appears to be reasonable to bring at least partial change in HbA1c. In fact, a larger reduction in HbA1c was reported by Petschnig et al. (Petschnig et al., Citation2020) using isolated supervised progressive resistance training with a duration of 32 weeks in 21 children with T1DM.
A better understanding of the metabolic and neuroendocrine responses to the various modalities of exercise performed by youth with T1DM is critical for determining appropriate nutritional and insulin management strategies (Riddell et al., Citation2017). Another important finding of the present study in this respect was that concurrent training (i.e. a combination of aerobic and strength training) showed slightly higher reductions in HbA1c and insulin doses per day. These results are in accord with the position of the ISPAD Clinical Practice Consensus Guidelines 2018 Compendium, who recommend both aerobic and strength activities for most people living with T1DM (Adolfsson et al., Citation2018). Similar benefits on several health parameters have been reported in obese children in response to concurrent training (García-Hermoso, Ramírez-Vélez, Ramírez-Campillo, Peterson, & Martínez-Vizcaíno, Citation2018). A possible explanation for this is the combined effect of a greater use of glucose, caused by an increased muscle mass, and the decreased insulin resistance (Svensson & Eriksson, Citation2011).
It is also unclear what is the most effective intensity of exercise for improvement of glycaemic control in T1DM. Our study suggests that high-intensity exercise training favours greater reductions in HbA1c and insulin dosage per day. This finding is consistent with that of Chimen et al. (Chimen et al., Citation2012) who confirmed that the studies reporting a glycaemic benefit involved high-intensity exercise in contrast to the majority of other studies that did not report a benefit and employed a moderate exercise programme. In this respect, higher high-intensity physical activity seems to be negatively related to adiposity and cardiometabolic risk among children and adolescents later in life (García-Hermoso, Ezzatvar, Ramírez-Vélez, Olloquequi, & Izquierdo, Citation2021). Also, high-intensity interval training has been shown to be more effective than continuous aerobic training in improving cardiovascular fitness and various parameters related to glucose metabolism in adults with T1DM (Farinha et al., Citation2018). To this end, more extensive studies clarifying the physiological and molecular basis of exercise adaptive responses, and their possible alterations in diabetes, are needed.
Overall, the frequency of hypoglycaemia experienced by youths in the intervention groups was similar in comparison to the control groups (Campaigne et al., Citation1985; Landt et al., Citation1985; Petschnig et al., Citation2020; Salem et al., Citation2010), indicating that exercise in trial settings can be undertaken safely in children and adolescents with T1DM. A possible explanation for this might be that the exercise protocols were designed with a limited duration and with specific exercises and were performed under controlled conditions. Also, some studies considered pre-exercise glucose levels of the participants according to the ADA recommendations6. For example, Petschnig et al. (Petschnig et al., Citation2020) required pre-exercise glucose levels of 115-280 mg/dL. This will give the ability to children and adolescents for learning about their individual glucose response to exercise and eventually establish an individual strategy to prevent exercise-induced hypoglycaemia.
Interpretation of these results must be considered with caution since they are subject to several limitations. First, the main limitation of this review is the low quality of included studies. Second, few RCTs considered important confounding factors such as diet or adjustment of insulin dosage. Third, only few studies provided information on the use of an insulin pump, insulin injections or a continuous glucose measurement system. Fourth, not all RCTs reported attendance at exercise sessions and the adherence to achieving intensity targets, and this is essential to know whether individuals performed as intended and adhered to the planned programme. Fifth, not all studies reported key factors involved as predictors of glycaemic control, such as age at diagnosis, disease duration, diet, nutritional status, and others (i.e. parental schooling, sexual maturation, and/or family income). Finally, publication bias may have affected the findings in this review as the Doi plot and the LFK index showed asymmetry for HbA1c.
In conclusion, the present review suggests that exercise interventions can have a significant effect on the reduction of HbA1c and insulin dose per day and can increase cardiorespiratory fitness in children and adolescents with T1DM. The findings suggest that longer interventions (i.e. ≥ 24 weeks and sessions ≥ 60 minutes) and inclusion of concurrent and high-intensity exercise (e.g. 77–95% of maximum heart rate and ≥ 70-84% of one-repetition maximum) might be more effective at improving these parameters. Also, as no episode of severe hypoglycaemia was reported during exercise in any of the RCTs included in our meta-analysis, our findings suggest that exercise in trial settings can be undertaken safely in children and adolescents with T1DM. Our findings contribute in several ways to our understanding on how to prescribe exercise in terms of duration, mode, and intensity among the paediatric population with diabetes and provide a basis for future studies.
Disclosure statement
No potential conflict of interest was reported by the author(s).
Additional information
Funding
References
- Adolfsson, P., Riddell, M. C., Taplin, C. E., Davis, E. A., Fournier, P. A., Annan, F., … Hofer, S. E. (2018). ISPAD Clinical Practice Consensus Guidelines 2018: Exercise in children and adolescents with diabetes. Pediatric Diabetes, 19, 205–226. doi:10.1111/pedi.12755
- Association, A. D. (2019). 5. Lifestyle management: Standards of medical care in diabetesd2019. Diabetes Care, 42, S46–S60. doi:10.2337/dc19-S005
- Association, A. D. (2020). Children and adolescents: Standards of medical care in diabetes- 2020. Diabetes Care, 43, S163–S182. doi:10.2337/dc20-S013
- Association, A. D. (2021). Glycemic targets: Standards of medical care in diabetes−2021. Diabetes Care, 44, S73–S84. doi:10.2337/dc21-S006
- Atkinson, M. A., & Eisenbarth, G. S. (2001). Type 1 diabetes: New perspectives on disease pathogenesis and treatment. Lancet, 358, 221–229. doi:10.1016/S0140-6736(01)05415-0
- Battelino, T., Danne, T., Bergenstal, R. M., Amiel, S. A., Beck, R., Biester, T., … Phillip, M. (2019). Clinical targets for continuous glucose monitoring data interpretation: Recommendations from the international consensus on time in range. Diabetes Care, 42, 1593–1603. doi:10.2337/dci19-0028
- Campaigne, B. N., Gilliam, T. B., Spencer, M. L., Lampman, R. M., & Schork, M. A. (1984). Effects of a physical activity program on metabolic control and cardiovascular fitness in children with insulin-dependent diabetes mellitus. Diabetes Care, 7, 57–62. doi:10.2337/diacare.7.1.57
- Campaigne, B. N., Landt, K. W., & Mellies, M. J. (1985). The effects of physical training on blood lipid profiles in adolescents with insulin-dependent diabetes mellitus. Physician and Sportsmedicine, 13, 83–89. doi:10.1080/00913847.1985.11708949
- Chetty, T., Shetty, V., Fournier, P. A., Adolfsson, P., Jones, T. W., & Davis, E. A. (2019). Exercise management for young people with type 1 diabetes: A structured approach to the exercise consultation. Frontiers in Endocrinology, 10, doi:10.3389/fendo.2019.00326
- Chimen, M., Kennedy, A., Nirantharakumar, K., Pang, T. T., Andrews, R., & Narendran, P. (2012). What are the health benefits of physical activity in type 1 diabetes mellitus? A literature review. Diabetologia, 55, 542–551. doi:10.1007/s00125-011-2403-2
- Colberg, S. R., Sigal, R. J., Yardley, J. E., Riddell, M. C., Dunstan, D. W., Dempsey, P. C., … Tate, D. F. (2016). Physical activity/exercise and diabetes: A position statement of the American Diabetes Association. Diabetes Care, 39, 2065–2079. doi:10.2337/dc16-1728
- D’hooge, R., Hellinckx, T., Van, L. C., Stegen, S., De, S. J., Van, A. S., … Calders, P. (2011). Influence of combined aerobic and resistance training on metabolic control, cardiovascular fitness and quality of life in adolescents with type 1 diabetes: A randomized controlled trial. Clinical Rehabilitation, 25, 349–359. doi:10.1177/0269215510386254
- DiMeglio, L. A., Acerini, C. L., Codner, E., Craig, M. E., Hofer, S. E., Pillay, K., & Maahs, D. M. (2018). ISPAD Clinical Practice Consensus Guidelines 2018: Glycemic control targets and glucose monitoring for children, adolescents, and young adults with diabetes. Pediatric Diabetes, 19, 105–114. doi:10.1111/pedi.12737
- Ditzel, J., & Standl, E. (1975). THE PROBLEM OF TISSUE OXYGENATION IN DIABETES MELLITUS: Evidence of disordered oxygen release from the erythrocytes of diabetics in various conditions of metabolic control. Acta Medica Scandinavica, 197, 59–68. doi:10.1111/j.0954-6820.1975.tb06503.x
- Doi, S. A. R., Furuya-Kanamori, L., Thalib, L., & Barendregt, J. J. (2017). Meta-analysis in evidence-based healthcare: A paradigm shift away from random effects is overdue. International Journal of Evidence-Based Healthcare, 15, 152–160. doi:10.1097/XEB.0000000000000125
- Evans, M. (1998). The UK Prospective Diabetes Study. Lancet, 352, 1932–1933. doi:10.1016/s0140-6736(05)60422-9
- Farinha, J. B., Ramis, T. R., Vieira, A. F., Macedo, R. C. O., Rodrigues-Krause, J., Boeno, F. P., … Reischak-Oliveira, A. (2018). Glycemic, inflammatory and oxidative stress responses to different high-intensity training protocols in type 1 diabetes: A randomized clinical trial. Journal of Diabetes and its Complications, 32, 1124–1132. doi:10.1016/j.jdiacomp.2018.09.008
- Follmann, D., Elliott, P., Suh, I., & Cutler, J. (1992). Variance imputation for overviews of clinical trials with continuous response. Journal of Clinical Epidemiology, 45, 769–773. doi:10.1016/0895-4356(92)90054-Q
- Furuya-Kanamori, L., Barendregt, J. J., & Doi, S. A. R. (2018). A new improved graphical and quantitative method for detecting bias in meta-analysis. International Journal of Evidence-Based Healthcare, 16, 195–203. doi:10.1097/XEB.0000000000000141
- Galler, A., Lindau, M., Ernert, A., Thalemann, R., & Raile, K. (2011). Associations between media consumption habits, physical activity, socioeconomic status, and glycemic control in children, adolescents, and young adults with type 1 diabetes. Diabetes Care, 34, 2356–2359. doi:10.2337/dc11-0838
- García-Hermoso, A., Ramírez-Vélez, R., Garciá-Alonso, Y., Alonso-Martínez, A. M., & Izquierdo, M. (2020). Association of Cardiorespiratory Fitness Levels during Youth with Health Risk Later in Life: A Systematic Review and Meta-analysis. JAMA Pediatrics, 174, 952–960. doi:10.1001/jamapediatrics.2020.2400
- García-Hermoso, A., Ezzatvar, Y., Ramírez-Vélez, R., Olloquequi, J., & Izquierdo, M. (2021). Is device-measured vigorous-intensity physical activity associated with health-related outcomes in children and adolescents? A systematic review and meta-analysis. Journal of Sport and Health Science, 10(3), 296–307. doi:10.1016/j.jshs.2020.12.001
- García-Hermoso, A., Ramírez-Vélez, R., Ramírez-Campillo, R., Peterson, M. D., & Martínez-Vizcaíno, V. (2018). Concurrent aerobic plus resistance exercise versus aerobic exercise alone to improve health outcomes in paediatric obesity: A systematic review and meta-Analysis. British Journal of Sports Medicine, 52, 161–166. doi:10.1136/bjsports-2016-096605
- Gusso, S., Pinto, T., Baldi, J. C., Derraik, J. G. B., Cutfield, W. S., Hornung, T., & Hofman, P. L. (2017). Exercise training improves but does not normalize left ventricular systolic and diastolic function in adolescents with type 1 diabetes. Diabetes Care, 40, 1264–1272. doi:10.2337/dc16-2347
- Heyman, E., Toutain, C., Delamarche, P., Berthon, P., Briard, D., Youssef, H., … Gratas-Delamarche, A. (2007). Exercise training and cardiovascular risk factors in Type 1 diabetic adolescent girls. Pediatric Exercise Science, 19, 408–419. doi:10.1123/pes.19.4.408
- Higgins, J., Thompson, S., & Deeks, J. (2003). Measuring inconsistency in meta–analyses. BMJ, 327(7414), 557–560.
- Higgins, J. P. T. (2008). Cochrane handbook for systematic reviews of interventions version 5.0. 1. The Cochrane
- Huttunen, N. P., Käär, M. L., Knip, M., Mustonen, A., Puukka, R., & Akerblom, H. K. (1984). Physical fitness of children and adolescents with insulin-dependent diabetes mellitus. Annals of clinical research, 16(1), 1–5.
- Huttunen, N. P., Lankela, S. L., Knip, M., Lautala, P., Kaar, M. L., Laasonen, K., & Puukka, R. (1989). Effect of once-a-week training program on physical fitness and metabolic control in children with IDDM. Diabetes Care, 12, 737–740. doi:10.2337/diacare.12.10.737
- Inthout, J., Ioannidis, J. P., & Borm, G. F. (2014). The Hartung-Knapp-Sidik-Jonkman method for random effects meta-analysis is straightforward and considerably outperforms the standard DerSimonian-Laird method. BMC Medical Research Methodology, 14, doi:10.1186/1471-2288-14-25
- Kennedy, A., Nirantharakumar, K., Chimen, M., Pang, T. T., Hemming, K., Andrews, R. C., & Narendran, P. (2013). Does Exercise Improve Glycaemic Control in Type 1 Diabetes? A Systematic Review and Meta-Analysis. PLoS ONE, doi:10.1371/journal.pone.0058861
- Kivelä, R., Silvennoinen, M., Touvra, A. M., Maarit Lehti, T., Kainulainen, H., Vihko, V., … Vihko, V. (2006). Effects of experimental type 1 diabetes and exercise training on angiogenic gene expression and capillarization in skeletal muscle. The FASEB Journal, 20, 1570–1572. doi:10.1096/fj.05-4780fje
- Kristiansen, S., Gade, J., Wojtaszewski, J. F. P., Kiens, B., & Richter, E. A. (2000). Glucose uptake is increased in trained vs. untrained muscle during heavy exercise. Journal of Applied Physiology, 89, 1151–1158. doi:10.1152/jappl.2000.89.3.1151
- Landt, K. W., Campaigne, B. N., James, F. W., & Sperling, M. A. (1985). Effects of exercise training on insulin sensitivity in adolescents with type I diabetes. Diabetes Care, 8, 461–465. doi:10.2337/diacare.8.5.461
- Liberati, A., Altman, D. G., Tetzlaff, J., Mulrow, C., Gøtzsche, P. C., Ioannidis, J. P. A., … Moher, D. (2009). The PRISMA Statement for Reporting Systematic Reviews and Meta-Analyses of Studies That Evaluate Health Care Interventions: Explanation and Elaboration. PLoS Medicine, 6, e1000100. doi:10.1371/journal.pmed.1000100
- Liese, A. D., Ma, X., Maahs, D. M., & Trilk, J. L. (2013). Physical activity, sedentary behaviors, physical fitness, and their relation to health outcomes in youth with type 1 and type 2 diabetes: A review of the epidemiologic literature. Journal of Sport and Health Science, 2, 21–38. doi:10.1016/j.jshs.2012.10.005
- Maahs, D. M., Daniels, S. R., De, F. S., Dichek, H. L., Flynn, J., Goldstein, B. I., … Urbina, E. (2014). Cardiovascular disease risk factors in youth with diabetes mellitus: A scientific statement from the American heart association. Circulation, 130, 1532–1558. doi:10.1161/CIR.0000000000000094
- Maher, C. G., Sherrington, C., Herbert, R. D., Moseley, A. M., & Elkins, M. (2003). Reliability of the PEDro scale for rating quality of randomized controlled trials. Physical therapy, 83(8), 713–721.
- McCarthy, M. M., Funk, M., & Grey, M. (2016). Cardiovascular health in adults with type 1 diabetes. Preventive Medicine, 91, 138–143. doi:10.1016/j.ypmed.2016.08.019
- Mccarthy, O., West, D. J., Yardley, J. E., Bally, L., Zueger, T., Stettler, C., … Reischak-oliveira, A. (2020). Differences in Physiological Responses to Cardiopulmonary Exercise Testing in Adults With and Without Type 1 Diabetes : A Pooled Analysis. Diabetes Care, 44, 240–247. doi:10.2337/dc20-1496
- Nazari, M., Shabani, R., & Dalili, S. (2020). The effect of concurrent resistance-aerobic training on serum cortisol level, anxiety, and quality of life in pediatric type 1 diabetes. Journal of Pediatric Endocrinology and Metabolism, 33, 599–604. doi:10.1515/jpem-2019-0526
- Ostman, C., Jewiss, D., King, N., & Smart, N. A. (2018). Clinical outcomes to exercise training in type 1 diabetes: A systematic review and meta-analysis. Diabetes Research and Clinical Practice, 139, 380–391. doi:10.1016/j.diabres.2017.11.036
- Petschnig, R., Wagner, T., Robubi, A., & Baron, R. (2020). Effect of Strength Training on Glycemic Control and Adiponectin in Diabetic Children. Medicine and science in sports and exercise, 52, 2172–2178. doi:10.1249/MSS.0000000000002356
- Piercy, K. L., Troiano, R. P., Ballard, R. M., Carlson, S. A., Fulton, J. E., Galuska, D. A., … Olson, R. D. (2018). The Physical Activity Guidelines for Americans. JAMA, 320, 2020–2028. doi:10.1001/jama.2018.14854
- Poortmans, J. R., Saerens, P., Edelman, R., Vertongen, F., & Dorchy, H. (1986). Influence of the degree of metabolic control on physical fitness in type 1 diabetic adolescents. International Journal of Sports Medicine, 7, 232–235. doi:10.1055/s-2008-1025765
- Quirk, H., Blake, H., Tennyson, R., Randell, T. L., & Glazebrook, C. (2014). Physical activity interventions in children and young people with Type 1 diabetes mellitus: A systematic review with meta-analysis. Diabetic Medicine, 31, 1163–1173. doi:10.1111/dme.12531
- Riddell, M. C., Gallen, I. W., Smart, C. E., Taplin, C. E., Adolfsson, P., Lumb, A. N., … Laffel, L. M. (2017). Exercise management in type 1 diabetes: a consensus statement. The Lancet Diabetes and Endocrinology, 5, 377–390. doi:10.1016/S2213-8587(17)30014-1
- Riebe, D., Ehrman, J. K., Liguori, G., Magal, M., & Medicine, A. o. S. (2018) ACSM’s guidelines for exercise testing and prescription. Wolters Kl.
- Roberts, L., Jones, T. W., & Fournier, P. A. (2002). Exercise training and glycemic control in adolescents with poorly controlled type 1 diabetes mellitus. Journal of Pediatric Endocrinology and Metabolism, 15, 621–627. doi:10.1515/JPEM.2002.15.5.621
- Robertson, K., Riddell, M. C., Guinhouya, B. C., Adolfsson, P., & Hanas, R. (2014). Exercise in children and adolescents with diabetes. Pediatric Diabetes, 15, 203–223. doi:10.1111/pedi.12176
- Rosenbauer, J., Dost, A., Karges, B., Hungele, A., Stahl, A., Bächle, C., … Holl, R. W. (2012). Improved metabolic control in children and adolescents with type 1 diabetes: A trend analysis using prospective multicenter data from Germany and Austria. Diabetes Care, 35, 80–86. doi:10.2337/dc11-0993
- Salem, M. A., Aboelasrar, M. A., Elbarbary, N. S., Elhilaly, R. A., & Refaat, Y. M. (2010). Is exercise a therapeutic tool for improvement of cardiovascular risk factors in adolescents with type 1 diabetes mellitus? A randomised controlled trial. Diabetology and Metabolic Syndrome, 2, doi:10.1186/1758-5996-2-47
- Shemin, D., & Rittenberg, D. (1946). The life span of the human red blood cell - PubMed. Journal of Biological Chemistry, 166, 627–636.
- Soedamah-Muthu, S. S., Fuller, J. H., Mulnier, H. E., Raleigh, V. S., Lawrenson, R. A., & Colhoun, H. M. (2006). High risk of cardiovascular disease in patients with type 1 diabetes in the U.K.: A cohort study using the General Practice Research Database. Diabetes Care, 29, 798–804. doi:10.2337/diacare.29.04.06.dc05-1433
- Stratton, R., Wilson, D. P., Endres, R. K., & Goldstein, D. E. (1987). Improved glycemic control after supervised 8-wk exercise program in insulin-dependent diabetic adolescents. Diabetes Care, 10, 589–593. doi:10.2337/diacare.10.5.589
- Svensson, M. K., & Eriksson, J. W. (2011). Change in the amount of body fat and IL-6 levels is related to altered insulin sensitivity in type 1 diabetes patients with or without diabetic nephropathy. Hormone and Metabolic Research, 43, 209–215. doi:10.1055/s-0031-1271622
- Tomar, R., Hamdan, M., & Al-Qahtani, M. H. (2014). Effect of low to moderate intensity walking and cycling on glycaemic and metabolic control in type 1 diabetes mellitus adolescent males: A randomized controlled trial. Isokinetics and Exercise Science, 22, 237–243. doi:10.3233/IES-140544
- Tonoli, C., Heyman, E., Roelands, B., Buyse, L., Cheung, S. S., Berthoin, S., & Meeusen, R. (2012). Effects of Different Types of Acute and Chronic (Training) Exercise on Glycaemic Control in Type 1 Diabetes Mellitus. Sports Medicine, 42, 1059–1080. doi:10.1007/bf03262312
- Tunar, M., Ozen, S., Goksen, D., Asar, G., Bediz, C. S., & Darcan, S. (2012). The effects of pilates on metabolic control and physical performance in adolescents with type 1 diabetes mellitus. Journal of Diabetes and its Complications, 26, 348–351. doi:10.1016/j.jdiacomp.2012.04.006
- Wu, N., Bredin, S. S. D., Jamnik, V. K., Koehle, M. S., Guan, Y., Shellington, E. M., … Warburton, D. E. R. (2021). Association between physical activity level and cardiovascular risk factors in adolescents living with type 1 diabetes mellitus: a cross-sectional study. Cardiovascular Diabetology, 20, doi:10.1186/s12933-021-01255-0