ABSTRACT
The biarticular triceps brachii long head (TBLong) is lengthened more in the overhead than neutral arm position. We compared triceps brachii hypertrophy after elbow extension training performed in the overhead vs. neutral arm position. Using a cable machine, 21 adults conducted elbow extensions (90−0°) with one arm in the overhead (Overhead-Arm) and the other arm in the neutral (Neutral-Arm) position at 70% one-repetition maximum (1RM), 10 reps/set, 5 sets/session, 2 sessions/week for 12 weeks. Training load was gradually increased (+5% 1RM/session) when the preceding session was completed without repetition failure. 1RM of the assigned condition and MRI-measured muscle volume of the TBLong, monoarticular lateral and medial heads (TBLat+Med), and whole triceps brachii (Whole-TB) were assessed pre- and post-training. Training load and 1RM increased in both arms similarly (+62−71% at post, P = 0.285), while their absolute values/weights were always lower in Overhead-Arm (-34−39%, P < 0.001). Changes in muscle volume in Overhead-Arm compared to Neutral-Arm were 1.5-fold greater for the TBLong (+28.5% vs. +19.6%, Cohen's d = 0.61, P < 0.001), 1.4-fold greater for the TBLat+Med (+14.6% vs. +10.5%, d = 0.39, P = 0.002), and 1.4-fold greater for the Whole-TB (+19.9% vs. +13.9%, d = 0.54, P < 0.001). In conclusion, triceps brachii hypertrophy was substantially greater after elbow extension training performed in the overhead versus neutral arm position, even with lower absolute loads used during the training.
Highlights
Growing evidence suggests that resistance training at long muscle lengths promotes muscle hypertrophy, but its practical applications are yet to be explored.
Triceps brachii muscle hypertrophy was substantially greater after cable elbow extension training performed in the overhead than neutral arm position, particularly in the biarticular triceps brachii long head, even with lower absolute loads lifted (i.e. lower mechanical stress to muscles/joints).
Cable elbow extension training should be performed in the overhead rather than neutral arm position if one aims to maximise muscle hypertrophy of the triceps brachii or to prevent atrophy of this muscle.
Introduction
Resistance training is widely recommended for athletic, general, and clinical populations due to its well-known benefits on physical performance and health (ACSM, Citation2009; Westcott, Citation2012). Increasing muscle size is one of the main goals of resistance training, and several evidence-based hypertrophy-oriented training guidelines have been established and updated in the past three decades for various training variables such as load/intensity, volume, and inter-set rest (Evans, Citation1999; Kassiano et al., Citation2022; Kraemer & Ratamess, Citation2004; Morton, Colenso-Semple, & Phillips, Citation2019; Schoenfeld & Grgic, Citation2019). On the other hand, only relatively recently have studies begun to examine the influence of muscle length/joint angle during exercise on muscle hypertrophy (Kubo et al., Citation2006; Alegre, Ferri-Morales, Rodriguez-Casares, & Aguado, Citation2014; McMahon, Morse, Burden, Winwood, & Onambele, Citation2014; Noorkoiv, Nosaka, & Blazevich, Citation2014; Stasinaki et al., Citation2018; Akagi, Hinks, & Power, Citation2020; Maeo et al., Citation2021; Pedrosa et al., Citation2021). Nevertheless, a growing body of evidence suggests that muscle hypertrophy is promoted when resistance training is conducted at long vs. short muscle lengths (see Oranchuk, Storey, Nelson, & Cronin, Citation2019 for a recent review), likely attributable, at least in part, to greater metabolic stress (Kooistra, de Ruiter, & de Haan, Citation2008) and IGF-1 expression (McMahon et al., Citation2014) associated with exercise at long muscle lengths.
Of the previous studies that compared muscle hypertrophy after training at long vs. short muscle lengths, almost all adopted isometric (Kubo et al., Citation2006; Alegre et al., Citation2014; Noorkoiv et al., Citation2014; Akagi et al., Citation2020) or partial range of motion (ROM) (McMahon et al., Citation2014; Stasinaki et al., Citation2018; Pedrosa et al., Citation2021) training at different joint angles about the same joint (e.g. knee extensors trained at knee flexed vs. extended positions). However, resistance training guidelines generally recommend dynamic exercises with a full ROM when possible (Evans, Citation1999; Kraemer & Ratamess, Citation2004; Morton et al., Citation2019). Thus, it is of great practical importance to compare hypertrophic effects of full ROM exercises that place the same target muscle(s) under long vs. short conditions. Based on the characteristics of biarticular muscles that their lengths are influenced by two joints the muscles cross, we recently compared hamstrings muscle hypertrophy induced by seated (hip-flexed: long) vs. prone (hip-extended: short) leg curl training performed with a full ROM (Maeo et al., Citation2021). The results clearly indicated that hypertrophy was greater for the seated than prone condition in the whole and three biarticular hamstrings, but equivalent in the monoarticular one, providing strong evidence that muscle length during exercise influences muscle hypertrophy. Such a finding can be readily utilised in the fitness and rehabilitation fields, giving a simple message that seated rather than prone leg curl is the choice for those aiming to increase hamstrings muscle size. The same approach can be tested in other major biarticular muscles in the limbs, the results of which will greatly contribute to efficient exercise programming for large populations and future research as well.
The triceps brachii acts as the primary elbow extensor and is considered to play crucial roles in various sports and daily life activities such as baseball throws, (Fleisig & Escamilla, Citation1996) tennis serves, (Buckley & Kerwin, Citation1988) and shot puts, (Terzis, Georgiadis, Vassiliadou, & Manta, Citation2003) as well as injury prevention from falls (Dietz, Noth, & Schmidtbleicher, Citation1981). Of its three constituent muscles, the biarticular triceps brachii long head (TBLong) crosses not only the elbow but also the shoulder joint, and is lengthened more in the overhead (shoulder-flexed) than neutral arm position () (Delp et al., Citation2007). Both overhead (Krings et al., Citation2016; Ode L et al., Citation2012) and neutral (Hussain, Sundaraj, Subramaniam, & Lam, Citation2020; Jurimae, Abernethy, Blake, & McEniery, Citation1996) position elbow extensions are commonly implemented in the field and literature (often called “overhead triceps extension” and “triceps pushdown”, respectively), but their difference has rarely been discussed, particularly for their hypertrophic effects. To the authors’ knowledge, only one study (Stasinaki et al., Citation2018) examined the effects of overhead vs. neutral position elbow extension training on triceps brachii hypertrophy, which did not find a significant difference between the two training conditions. However, some methodological issues should be considered when interpreting their results. First and most importantly, their training period (6 weeks) and sample size (n = 9/condition) were likely insufficient, which appears to be the main reason for not finding a statistical difference even though the overhead condition had a 1.5-fold greater increase in a muscle size measure. Second, the training was conducted with a partial ROM of the elbow joint in combination with different shoulder joint angles, which itself is not a problem but contradicts with the general training guidelines as mentioned above. These aspects motivated us to reexamine the hypertrophic effects of overhead vs. neutral position elbow extension training with a more methodologically robust and practical approach, although we commend the previous study (Stasinaki et al., Citation2018) for its novelty.
Figure 1. Postures of the shoulder and elbow joints (A and B) and operating ranges of each triceps brachii muscle on the normalised force–length curve (C–E) during the elbow extensions performed in the overhead and neutral arm positions. These were obtained using the OpenSim Arm26 model, (Delp et al., Citation2007) with the shoulder joint flexed at 180° and 0° for the overhead and neutral conditions, respectively, and the elbow joint angle ranging 90−0° for both conditions as conducted in this study. It can be clearly seen that the TBLong operates at longer muscle lengths in the overhead than neutral condition, while there is no difference between the conditions in the TBLat and TBMed. TBLong = triceps brachii long head; TBLat and TBMed triceps brachii lateral and medial heads, respectively.
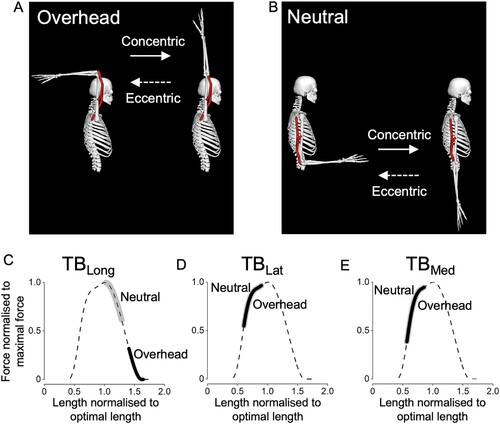
The purpose of this study was therefore to examine the effects of elbow extension training performed in the overhead vs. neutral arm position on triceps brachii muscle hypertrophy. To this end, we designed a 12-week training intervention study using a within-participant comparison model, a powerful approach for comparing hypertrophic responses induced by two types of interventions (MacInnis, McGlory, Gibala, & Phillips, Citation2017). A sample size of 21 (i.e. n = 21 arms per condition) was deemed well sufficient based on our recent study (Maeo et al., Citation2021) using the same training intervention model on the hamstrings (n = 20 legs per condition). We hypothesised that 1) hypertrophy of the TBLong and consequently the whole triceps brachii (Whole-TB) would be greater, but 2) that of the monoarticular lateral and medial heads (TBLat+Med) would be equivalent, when elbow extension training is performed in the overhead vs. neutral arm position.
Methods
Participants and study overview
Twenty-one healthy young adults (14 males, age: 23.0 ± 1.4 y, height: 1.73 ± 0.07 m, body mass: 70.0 ± 11.1 kg; 7 females, age: 24.3 ± 1.6 y, height: 1.61 ± 0.07 m, body mass: 53.6 ± 6.3 kg) participated in this study, which was approved by the Ethics Committee of Ritsumeikan University (BKC-IRB-2018-087). Written informed consent was obtained from each participant. The participants were all healthy, but none had been involved in any type of systematic resistance training programme in the past 12 months. They conducted 12-week unilateral elbow extension training with one arm in the overhead position (Overhead-Arm) and the other arm in the neutral position (Neutral-Arm). All participants attended three measurement sessions; two sessions before the training period (Pre 1 and Pre 2) separated by 2−7 days, and one session after the training period (Post) 2−4 days after the final training session. Participants were instructed to avoid any intensive and unfamiliar physical activities for the arms within 2 days before Pre 1 and throughout the experimental period. Each measurement session involved MRI scans and one-repetition maximum (1RM) measurements. Training and measurements were conducted as follows.
Training programme
Each arm was assigned to Overhead-Arm or Neutral-Arm, with the dominant and non-dominant arms counterbalanced using a computer-generated list. Both arms were trained unilaterally with the assigned training condition in a standing position by using a cable machine (Signature Series Dual Adjustable Pulley, Life Fitness, Chicago, IL) with the shoulder joint fixed at 180° flexion for Overhead-Arm and 0° for Neutral-Arm (0° = anatomical position) (). The elbow joint ROM was 90−0° for both conditions, which we defined as the full ROM, with the wrist kept supinated (to help keep the shoulder adducted). Each training session commenced with 5−10 warm-up repetitions at 50% of the load prescribed for that session (detailed below) with the assigned training condition. Participants then performed the elbow extensions 10 repetitions/set for 5 sets, taking 2 s for each of the concentric (elbow extending) and eccentric (elbow flexing) phases without a pausing phase with the guide of a metronome (60 bpm). 2-min rest intervals were taken in between sets. After training one arm (5 sets), the other arm was trained. The preceding arm was counterbalanced in the first training session among participants, and it was switched every session for each participant. Training was conducted twice per week on nonconsecutive days. Training load was gradually increased at the first, second, and third sessions from 50, 60, and 70% of 1RM measured pre-training (detailed below), respectively, and 70% of 1RM was used thereafter. This protocol was the same as for our previous study (Maeo et al., Citation2021) targeting the hamstrings, and similar to those of previous studies (Stasinaki et al., Citation2018; Kawakami, Abe, Kuno, & Fukunaga, Citation1995; Sugisaki et al., Citation2015; Wakahara, Fukutani, Kawakami, & Yanai, Citation2013) targeting the triceps brachii, which all found typical hypertrophy for the targeted muscles (see Discussion). At least one examiner always supervised the training sessions and provided verbal encouragement, and corrected the joint positions and/or movement speed of the exercise when necessary. The examiners also assisted (spotted) the participants in executing the exercise when they could no longer repeat the repetitions up to 10 in each set. This was done in such a way that the participants could complete the task in a controlled manner with their continuous (∼maximum) efforts. If the participants could complete all the prescribed protocol without examiners’ assist at the third session and thereafter, +5% of 1RM was added at the subsequent sessions.
1RM
1RM was measured in each arm with the assigned training condition using the same cable machine as for the training. At Pre 1, participants were familiarised with the exercise by performing 3−5 repetitions with a light load, which was gradually increased (3−5 stages) with a short rest period (10−20 s) until the participants felt it somewhat heavy. Thereafter, only 1 repetition was performed at each increasing load by increments of 1.25 kg, with ≥2 min rest in between trials. 1RM was defined as the maximum load lifted with the proper joint positions, which was checked by the examiner(s). At Pre 2, participants performed 5, 3, and 1 warm-up repetitions at 50%, 75%, and 90% of 1RM of Pre 1, respectively, with 30 s rest, and tried the same 1RM load of Pre 1 after ≥2 min rest. 1RM was determined by increasing or decreasing the load thereafter. At Post, the warm-up and 1RM assessment were similarly conducted but based on the 1RM of Pre 2. The training load and its increments in the training sessions described above were also based on the 1RM of Pre 2. The mean within-participant coefficient of variation (CV) between the two pre-training sessions were 8.0% and 10.5% for the Overhead-Arm and Neutral-Arm 1RM, respectively. Data from Pre 2 was used for further analysis as the Pre value.
MRI
Preceding the 1RM measurement, longitudinal relaxation time-weighted cross-sectional MRIs of the upper arm were obtained for each arm using 18-channel body array and spine coils (Body 18 and CP Spine Array Coil, Siemens Healthineers, Germany) with the following parameters (modified based on previous studies Kawakami et al., Citation1995; Wakahara et al., Citation2013 [e.g. slice thickness and gap were reduced from 10 mm to 2.5 mm for a more precise analysis]): field of view, 250*250 mm; matrix, 448*336; pixel size, 0.56*0.74 mm; in-plane resolution, 0.42 mm; TR, 700 ms; TE, 9.4 ms; flip angle, 120°; gap, 2.5 mm; slice thickness, 2.5 mm; number of slices, 32*2 blocks (). Participants lay supine with their arms extended and muscles relaxed in a 3T magnet bore (MAGNETOM Skyra, Siemens Healthineers, Germany), and 2 series of blocks were taken with the most proximal slice set at the widest part of the humerus head to cover the whole triceps brachii for each arm.
Figure 2. Example images of the right arm MRIs at ∼50% of the whole length of the triceps brachii taken at Pre (A) and Post (B). Anatomical cross-sectional areas of the Long, Lat, and Med heads of the triceps brachii are drawn. Lat = lateral; Long = long; Med = medial; Post = post-training; Pre = pre-training.
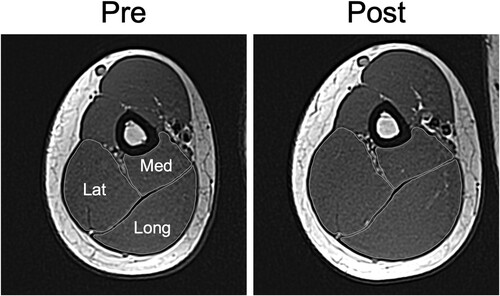
Images were analysed by using image analysis software (Horos, Horos Project), with the MRI data anonymised and investigators blinded to the training conditions. Anatomical cross-sectional areas (ACSAs) of the individual triceps brachii muscles were manually outlined in every other image from the most proximal to the most distal image in which the muscle was visible. Care was taken to exclude visible adipose and connective tissue incursions. ACSAs for the skipped images and gaps were estimated based on linear interpolation between the images in which ACSAs were outlined (Maeo, Shan, Otsuka, Kanehisa, & Kawakami, Citation2018). The volume of individual muscle was determined by summing all ACSAs for that muscle multiplied by the slice thickness. Due to difficulty in separating the monoarticular lateral and medial heads in the distal regions in some participants, the muscle volumes of these muscles are reported as the sum of these muscles (i.e. TBLat+Med). The Whole-TB volume was calculated by summing the volumes of TBLong and TBLat+Med. The mean within-participant CVs between the two pre-training sessions for the muscle volume were as follows: TBLong, 2.5%; TBLat+Med, 1.6%; Whole-TB, 1.5%. Data from the two pre-training sessions were averaged and used for further analysis as the Pre value.
Statistical analysis
All data were analysed using SPSS software (version 25, IBM Corp, USA) unless otherwise noted. Statistical significance was set at P < 0.05. Residuals were checked for normality and homoscedasticity by Shapiro–Wilk's test and Levene's test, respectively, and all data set were confirmed by the tests except for relative increase in 1RM and relative training load (explained below). Changes in muscle volumes from Pre to Post were compared between arms by an ANCOVA with the Pre values as covariates and the Post values as the dependent variables. A linear mixed-effects model was used with a subject as a random effect and an arm (training condition) as a fixed effect. Effect sizes of between-condition differences were calculated as Cohen's d values based on absolute change values, and were interpreted as trivial < 0.2; small 0.2–0.49; moderate 0.5-0.79; and large ≥ 0.8 (Cohen, Citation1988). To improve statistical inference, mean difference from baseline with their bootstrap 95% confidence interval (CI) was calculated for each condition by using estimation statistics (Ho, Tumkaya, Aryal, Choi, & Claridge-Chang, Citation2019).
We also explored if there were any differences in 1RM and training load between the arms (training conditions). Absolute 1RM at Pre and Post and absolute training load at each training session were compared between arms by a paired t-test at each time point. Relative increase in 1RM at Post and relative training load at each session, both expressed as % 1RM of Pre, were compared between arms by the Wilcoxon signed-rank test because most of the data at the early training sessions were not normally distributed due to prescribing the training loads based on % 1RM values. The main statistical results were the same when the relative data were compared by paired t-tests, or when absolute data were z-scored and compared by paired t-tests. Descriptive data are presented as means ± SDs.
Results
Training load
Training load gradually increased in both Overhead-Arm and Neutral-Arm (). In the relative values, there were significant but only minor differences between arms at some of the first half sessions (P = 0.025−0.044 at sessions 4, 7, 9, 10, 12), and no significant differences existed at the second half sessions (P = 0.195−0.876). On the other hand, absolute training load was always lower for Overhead-Arm than Neutral-Arm at all sessions (P < 0.001), as can be clearly seen from .
Figure 3. Relative (A) and absolute (B) training load used in the training sessions. Training load was gradually increased at the first, second, and third sessions from 50%, 60%, and 70% 1RM measured pre-training, respectively, and 70% of 1RM was used thereafter. If the participants could complete all the prescribed protocol at the third session and thereafter without repetition failure, +5% 1RM was added at the subsequent sessions. *P < 0.05, between conditions. Neutral-Arm = the arm that trained in the neutral position; Overhead-Arm = the arm that trained in the overhead position.
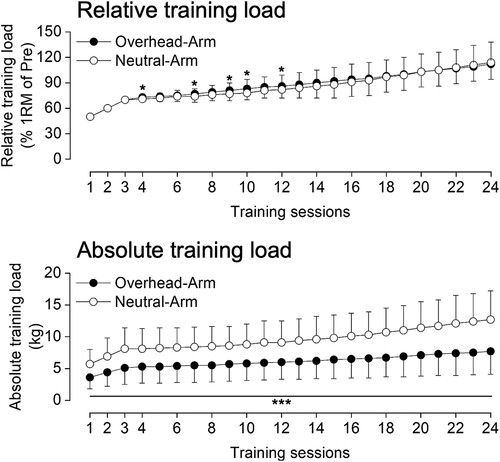
1RM
Absolute 1RM was lower for Overhead-Arm than Neutral-Arm at both Pre (7.2 ± 3.7 vs. 11.5 ± 4.7 kg, P < 0.001) and Post (12.0 ± 5.8 vs. 18.3 ± 6.9 kg, P < 0.001). Relative increases in 1RM did not significantly differ between the arms (71.4 ± 46.8% vs. 62.3 ± 29.2%, P = 0.285).
Muscle volume
There were significant differences in mean muscle volume changes between the arms in each of the TBLong, TBLat+Med, and Whole-TB (; see Table for details, Supplementary data 1). The increases in muscle volume were greater for Overhead-Arm than Neutral-Arm in the TBLong (ANCOVA-adjusted mean change: +28.5% vs. +19.6%, 1.5-fold difference, P < 0.001, Cohen's d = 0.61 [medium] based on absolute changes of 50.6 ± 30.6 vs 34.5 ± 21.2 cm3), TBLat+Med (+14.6% vs. +10.5%, 1.4-fold difference, P = 0.002, d = 0.39 [small] based on absolute changes of 42.1 ± 33.4 vs 30.4 ± 26.9 cm3), and Whole-TB (+19.9% vs. +13.9%, 1.4-fold difference, P < 0.001, d = 0.54 [medium] based on absolute changes of 92.8 ± 58.1 vs 64.9 ± 45.2 cm3).
Figure 4. Changes in muscle volume of the TBLong (A), TBLat+Med (B), and Whole-TB (C) after the training. In each subfigure/muscle(s), the raw data is plotted on the upper axes for Overhead-Arm (left) and Neutral-Arm (right); each paired set of observations at Pre and Post is connected by a line. On the lower axes, each paired mean difference is plotted as a bootstrap sampling distribution. Mean differences are depicted as dots with horizontal dashed lines; 95% confidence intervals are indicated by the ends of the vertical error bars. ***P < 0.001 and **P < 0.01, difference between arms (conditions) based on a baseline-adjusted ANCOVA. d values indicate Cohen's d effect sizes of between-group differences based on absolute change values. The bar graphs in the summary figure (D) are based on the ANCOVA-adjusted mean changes for each muscle(s). TBLong = triceps brachii long head; TBLat+Med = triceps brachii lateral and medial heads; Neutral-Arm = the arm that trained in the neutral position; Overhead-Arm = the arm that trained in the overhead position; Whole-TB = whole triceps brachii.
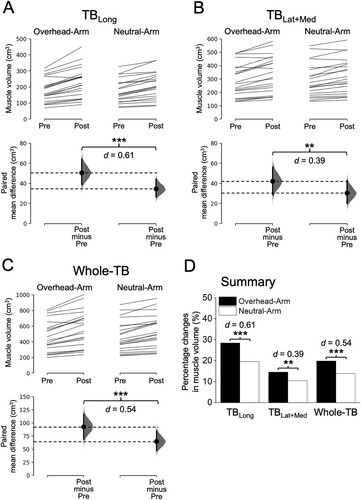
Discussion
The primary finding of this study was that hypertrophy of the triceps brachii was greater after elbow extension training performed in the overhead vs. neutral arm position, not only in the TBLong and Whole-TB but also in the TBLat+Med. This supported our first hypothesis on the TBLong and Whole-TB, but refuted the second hypothesis on the TBLat+Med for which we had presumed equivalent hypertrophic effects of the overhead and neutral conditions. Nevertheless, the observed clearly greater hypertrophy of the overall triceps brachii after overhead vs. neutral position elbow extension training would be highly useful in exercise prescription and designing future studies.
Muscle hypertrophy
Changes in the Whole-TB volume induced by the 12-week twice-weekly training intervention of this study were +19.9% for the Overhead-Arm and +13.5% for the Neutral-Arm, which correspond to +0.83% and +0.58% per session, respectively. These are in the range of the reported gains (+0.55–1.19% per session) in the Whole-TB volume or ACSA after traditional isotonic resistance training for the triceps brachii performed 2−3 times per week for 6−16 weeks (Stasinaki et al., Citation2018; Kawakami et al., Citation1995; Sugisaki et al., Citation2015; Wakahara et al., Citation2013). Thus, it appears that typical triceps brachii hypertrophy was induced in this study as well, although a direct comparison is difficult given different methodology used by this study vs. previous ones such as training frequency and period to name a few. It may be worth mentioning that these studies adopted various triceps brachii exercises such as standing (Kawakami et al., Citation1995) or lying (Sugisaki et al., Citation2015) elbow extension using a dumbbell, and lying dumbbell press (Wakahara et al., Citation2013). It is beyond the scope of this study to discuss which or any combination of these is more effective than others in promoting triceps brachii muscle hypertrophy. Nevertheless, given that the hypertrophy pattern was the same for both Overhead-Arm and Neutral-Arm in a sense that both had greater hypertrophy in the TBlong than TBLat+Med (), there may be no need to combine these to optimise Whole-TB hypertrophy (i.e. only the overhead condition is fine). On the other hand, there is some evidence that single-joint (Maeo, Shan, Otsuka, Kanehisa, & Kawakami, Citation2018) and multi-joint (Kubo, Ikebukuro, & Yata, Citation2019) exercises preferentially train biarticular and monoarticular muscles, respectively, the former of which is supported by this study. Thus, a combination of overhead elbow extension (single-joint exercise) and lying dumbbell press (multi-joint exercise) may further promote hypertrophy of the Whole-TB. Further research is needed to directly explore this issue, and the approach and results of this study would be an informative guide for developing such work.
Based on our recent finding (Maeo et al., Citation2021) that muscle hypertrophy was greater after seated than prone leg curl training exclusively in the biarticular hamstrings (i.e. equivalent in the monoarticular one), we hypothesised greater hypertrophy of the overhead than neutral condition to occur in the TBLong (and Whole-TB) but not in the TBLat+Med. However, the overhead condition induced greater hypertrophy than the neutral condition not only in the TBLong but also in the TBLat+Med (). This suggests that factors other the muscle length difference during exercise influenced the degree of hypertrophy of the monoarticular muscles, in contrast to our previous study on the hamstrings (Maeo et al., Citation2021). A potential reason may be related to the muscle length of the TBLong and its associated force contribution to the elbow extension task. More specifically, as can be seen from the simulation data in , the TBLong is lengthened in the overhead condition, operating at around the edge of the descending limb of the force-length curve, to the point where it can only produce a small force. This would result in proportionally greater force contribution from the TBLat+Med to the elbow extension task in the overhead vs. neutral condition due to reduced contribution from the TBLong, which is supported by a recent simulation study (Kholinne et al., Citation2018). This was likely not the case for the seated vs. prone leg curl training, in which the biarticular muscles operated at much wider regions (i.e. not around the edge) of the descending limb in the seated condition (see of Maeo et al., Citation2021) compared to the case for this study, with other synergists such as the sartorius and gracilis also collaboratively contributing to the knee flexion task (Maeo et al., Citation2021).
Another explanation for greater hypertrophy found not only in the TBLong but also in the TBLat+Med may be due to reduced muscle blood flow concomitant with maintaining the overhead arm position, (Tschakovsky & Hughson, Citation2000) which may have increased the metabolic stress within the muscle and promoted hypertrophy, (Pearson & Hussain, Citation2015) likely irrespective of bi- and monoarticular muscles. Again, this was perhaps not the case for the seated vs. prone leg curl training (Maeo et al., Citation2021), in which the legs were approximately in a horizontal position when extended in both conditions. Unfortunately, it is unknown how much of these potential mechanisms alone or in combination influenced the degree of muscle hypertrophy, so we cannot rule out the possibility that training at long muscle length in the overhead condition per se had no influence on the hypertrophy of the TBLong. However, it is worth noting that the difference between the conditions (overhead > neutral) in the degree of hypertrophy was slightly more pronounced in the TBLong vs. TBLat+Med (mean change difference: 1.5-fold vs. 1.4-fold, Cohen's d: 0.61 [medium] vs. 0.39 [small], overlaps in the 95% CI: smaller vs. larger, ). Thus, we consider that the hypertrophy of the TBLong was at least partly promoted by being more lengthened in the overhead than neutral condition during the exercise, while either or both mechanisms mentioned above may also have played some roles in promoting hypertrophy of the TBLat+Med.
Training load and 1RM
Here, we discuss the training loads used and their shifts as well as changes in maximum strength, together with the hypertrophy results, to provide practical information and a novel aspect of associations among them found in this study. Relative training load (% 1RM of Pre) increased similarly for both training conditions although there were some minor albeit significant differences between conditions in the first half sessions (A). After the intervention, relative increases in 1RM did not significantly differ between the overhead vs. neutral conditions. These indicate that relative training load and improvement in muscle strength were equivalent between the two training conditions. Given that the hypertrophy was greater for the overhead than neutral condition as discussed above, the similarity of strength improvement might seem inconsistent. However, it is well documented that strength improvement induced by a relatively short (e.g. ≤ 12 weeks) resistance training intervention is mainly attributable to neural factors such as increased neural drive although increases in muscle size could also contribute to the strength gain (Maeo et al., Citation2018). This study did not measure any neurophysiological parameters to assess neural changes induced by the training since the focus of this study was to compare changes in muscle size. Nevertheless, we recommend future studies measure both neural and muscle size parameters to better understand how each of these contributes to training-induced strength gains, ideally by multiple measurements over the time course of long-term intervention (e.g. > 6 months).
Absolute training load (kg) was always lower for the overhead than neutral condition (B) because absolute 1RM was lower for the overhead condition at both Pre and Post. This is likely at least partly attributable to the TBLong operating at around the edge of the descending limb of the force-length curve (i.e. lower force-generating capacity) in the overhead condition (). Taken together with the hypertrophy results, this indicates that absolute training load is not a determinant of training-mediated hypertrophic gains. Although such a finding is already reported by previous studies (Mitchell et al., Citation2012; Schoenfeld, Peterson, Ogborn, Contreras, & Sonmez, Citation2015) that showed similar hypertrophy after high vs. low load resistance training performed to repetition failure, they used the same exercise and body position for both conditions, indicating that both absolute and relative load were different between the conditions. It is important to note that low (relative) load, compared to traditional high load, resistance training performed to failure requires substantially greater number of repetitions and is more time-consuming, which may limit its practical use. In contrast, relative training load in this study was equivalent between conditions (or we intentionally matched them, at least at the initial sessions, A), and the number of repetitions and therefore total time were the same for both conditions throughout the interventions. Thus, this study supports the previous studies (Mitchell et al., Citation2012; Schoenfeld et al., Citation2015) and adds that the environment within the muscle such as muscle length during exercise, rather than absolute or even relative training load, can be a strong determinant of training-induced muscle hypertrophy. This aspect would be particularly relevant in rehabilitation and periodisation since lower absolute load indicates lower mechanical stress on muscles and joints.
Limitations and future perspectives
This study provided further evidence with a practical model that training at long vs. short muscle lengths promotes muscle hypertrophy (Maeo et al., Citation2021), which may be partly attributable to greater metabolic stress (Kooistra et al., Citation2008) and IGF-1 expression (McMahon et al., Citation2014) associated with exercise at long muscle lengths. However, it was also found that other factors such as body position-related changes in muscle blood flow may also have influenced the degree of muscle hypertrophy, likely irrespective of bi- and monoarticular muscles. Thus, future studies comparing hypertrophic effects of resistance training at different joint angles/muscle lengths, especially when manipulating an angle of one of two joints biarticular muscles cross (as conducted in this study), should consider/examine the influence of body position-related changes in muscle blood flow. It is also necessary to explore the mechanisms underpinning greater hypertrophy after training at long vs. short muscle lengths as well as its dose–response relationship using both basic science and practical approaches. Specifically, it is currently unknown whether muscles should be lengthened as much as possible during exercise, or there is an optimal lengthened state, to achieve maximum hypertrophy. Clarifying these will largely contribute to refining effective and safe exercise prescription.
Conclusion
In summary, elbow extension training performed in the overhead vs. neutral position produced greater hypertrophy in the biarticular triceps brachii as expected, which appears to be at least partly attributable to the between-condition difference in its muscle length during exercise. On the other hand, greater hypertrophy of the overhead condition was also found in the monoarticular muscles, in which muscle lengths do not differ between the conditions. This may suggest involvement of muscle length-related proportionally greater force contribution from the monoarticular muscles due to reduced contribution from the biarticular one, and/or arm position-related reduced muscle blood flow, as additional hypertrophy-promoting factors in the overhead condition. In either case, hypertrophy of the overall triceps brachii was consequently much greater after overhead vs. neutral elbow extension training, even with lower absolute loads lifted (i.e. lower mechanical stress on muscles/joints). Thus, we recommend elbow extension training should be performed in the overhead rather than neutral arm position if one aims to maximise muscle hypertrophy of the triceps brachii or to prevent atrophy of this muscle.
Supplemental Material
Download MS Word (47.4 KB)Acknowledgments
This work was supported by a research grant from the Descente and Ishimoto Memorial Foundation of Sports Science to SM. We thank Professor Jonathan Folland at Loughborough University for his comments on the interpretation of the hypertrophy of the monoarticular muscles and Mr. Takahiro Tanaka at Ritsumeikan University for his support with the OpenSim simulation analysis.
Disclosure statement
No potential conflict of interest was reported by the author(s).
Additional information
Funding
References
- ACSM. (2009). Progression models in resistance training for healthy adults. Medicine & Science in Sports & Exercise, 41, 687–708.
- Akagi, R., Hinks, A., & Power, G. A. (2020). Differential changes in muscle architecture and neuromuscular fatigability induced by isometric resistance training at short and long muscle-tendon unit lengths. Journal of Applied Physiology, 129, 173–184.
- Alegre, L. M., Ferri-Morales, A., Rodriguez-Casares, R., & Aguado, X. (2014). Effects of isometric training on the knee extensor moment-angle relationship and vastus lateralis muscle architecture. European Journal of Applied Physiology, 114, 2437–2446.
- Buckley, J. P., & Kerwin, D. G. (1988). The role of the biceps and triceps brachii during tennis serving. Ergonomics, 31, 1621–1629.
- Cohen, J. (1988). Statistical power analysis for the behavioral sciences. Hillsdale, N.J.: L. Erlbaum Associates.
- Delp, S. L., Anderson, F. C., Arnold, A. S., Loan, P., Habib, A., John, C. T., … Thelen, D. G. (2007). <![CDATA[OpenSim: Open-source software to create and analyze dynamic simulations of movement]]>. IEEE Transactions on Biomedical Engineering, 54, 1940–1950.
- Dietz, V., Noth, J., & Schmidtbleicher, D. (1981). Interaction between pre-activity and stretch reflex in human triceps brachii during landing from forward falls. The Journal of Physiology, 311, 113–125.
- Evans, W. J. (1999). Exercise training guidelines for the elderly. Medicine & Science in Sports & Exercise, 31, 12–17.
- Fleisig, G. S., & Escamilla, R. F. (1996). Biomechanics of the elbow in the throwing athlete. Operative Techniques in Sports Medicine, 4, 62–68.
- Ho, J., Tumkaya, T., Aryal, S., Choi, H., & Claridge-Chang, A. (2019). Moving beyond p values: Data analysis with estimation graphics. Nature Methods, 16, 565–566.
- Hussain, J., Sundaraj, K., Subramaniam, I. D., & Lam, C. K. (2020). Muscle fatigue in the three heads of triceps brachii during intensity and speed variations of triceps push-down exercise. Frontiers in Physiology, 11, 112–124.
- Jurimae, J., Abernethy, P. J., Blake, K., & McEniery, M. T. (1996). Changes in the myosin heavy chain isoform profile of the triceps brachii muscle following 12 weeks of resistance training. European Journal of Applied Physiology and Occupational Physiology, 74, 287–292.
- Kassiano, W., Nunes, J. P., Costa, B., Ribeiro, A. S., Schoenfeld, B. J., & Cyrino, E. S. (2022). Does varying resistance exercises promote superior muscle hypertrophy and strength gains? A systematic review. Journal of Strength and Conditioning Research, 36, 1753–1762.
- Kawakami, Y., Abe, T., Kuno, S. Y., & Fukunaga, T. (1995). Training-induced changes in muscle architecture and specific tension. European Journal of Applied Physiology and Occupational Physiology, 72, 37–43.
- Kholinne, E., Zulkarnain, R. F., Sun, Y. C., Lim, S., Chun, J. M., & Jeon, I. H. (2018). The different role of each head of the triceps brachii muscle in elbow extension. Acta Orthopaedica et Traumatologica Turcica, 52, 201–205.
- Kooistra, R. D., de Ruiter, C. J., & de Haan, A. (2008). Knee angle-dependent oxygen consumption of human quadriceps muscles during maximal voluntary and electrically evoked contractions. European Journal of Applied Physiology, 102, 233–242.
- Kraemer, W. J., & Ratamess, N. A. (2004). Fundamentals of resistance training: Progression and exercise prescription. Medicine & Science in Sports & Exercise, 36, 674–688.
- Krings, B. M., Rountree, J. A., McAllister, M. J., Cummings, P. M., Peterson, T. J., Fountain, B. J., … Smith, J. W. (2016). Effects of acute carbohydrate ingestion on anaerobic exercise performance. Journal of the International Society of Sports Nutrition, 13, 40–50.
- Kubo, K., Ikebukuro, T., & Yata, H. (2019). Effects of squat training with different depths on lower limb muscle volumes. European Journal of Applied Physiology, 119, 1933–1942.
- Kubo, K., Ohgo, K., Takeishi, R., Yoshinaga, K., Tsunoda, N., Kanehisa, H., & Fukunaga, T. (2006). Effects of isometric training at different knee angles on the muscle-tendon complex in vivo. Scandinavian Journal of Medicine and Science in Sports, 16, 159–167.
- MacInnis, M. J., McGlory, C., Gibala, M. J., & Phillips, S. M. (2017). Investigating human skeletal muscle physiology with unilateral exercise models: When one limb is more powerful than two. Applied Physiology, Nutrition, and Metabolism, 42, 563–570.
- Maeo, S., Huang, M., Wu, Y., Sakurai, H., Kusagawa, Y., Sugiyama, T., … Isaka, T. (2021). Greater hamstrings muscle hypertrophy but similar damage protection after training at long versus short muscle lengths. Medicine & Science in Sports & Exercise, 53, 825–837.
- Maeo, S., Shan, X., Otsuka, S., Kanehisa, H., & Kawakami, Y. (2018a). Neuromuscular adaptations to work-matched maximal eccentric versus concentric training. Medicine & Science in Sports & Exercise, 50, 1629–1640.
- Maeo, S., Shan, X., Otsuka, S., Kanehisa, H., & Kawakami, Y. (2018b). Single-joint eccentric knee extension training preferentially trains the rectus femoris within the quadriceps muscles. Translational Sports Medicine, 1, 212–220.
- McMahon, G., Morse, C. I., Burden, A., Winwood, K., & Onambele, G. L. (2014). Muscular adaptations and insulin-like growth factor-1 responses to resistance training are stretch-mediated. Muscle & Nerve, 49, 108–119.
- Mitchell, C. J., Churchward-Venne, T. A., West, D. W., Burd, N. A., Breen, L., Baker, S. K., & Phillips, S. M. (2012). Resistance exercise load does not determine training-mediated hypertrophic gains in young men. Journal of Applied Physiology, 113, 71–77.
- Morton, R. W., Colenso-Semple, L., & Phillips, S. M. (2019). Training for strength and hypertrophy: An evidence-based approach. Current Opinion in Physiology, 10, 90–95.
- Noorkoiv, M., Nosaka, K., & Blazevich, A. J. (2014). Neuromuscular adaptations associated with knee joint angle-specific force change. Medicine & Science in Sports & Exercise, 46, 1525–1537.
- Ode L, L.-F., Robertson, R. J., Farah, B. Q., Rodrigues, S. L., Cyrino, E. S., & Ritti-Dias, R. M. (2012). Effects of exercise intensity on rating of perceived exertion during a multiple-set resistance exercise session. Journal of Strength and Conditioning Research, 26, 466–472.
- Oranchuk, D. J., Storey, A. G., Nelson, A. R., & Cronin, J. B. (2019). Isometric training and long-term adaptations: Effects of muscle length, intensity, and intent: A systematic review. Scandinavian Journal of Medicine & Science in Sports, 29, 484–503.
- Pearson, S. J., & Hussain, S. R. (2015). A review on the mechanisms of blood-flow restriction resistance training-induced muscle hypertrophy. Sports Medicine, 45, 187–200.
- Pedrosa, G. F., Lima, F. V., Schoenfeld, B. J., Lacerda, L. T., Simões, M. G., Pereira, M. R., … Chagas, M. H. (2021). Partial range of motion training elicits favorable improvements in muscular adaptations when carried out at long muscle lengths. European Journal of Sport Science, 1–11.
- Schoenfeld, B. J., & Grgic, J. (2019). Does training to failure maximize muscle hypertrophy? Strength & Conditioning Journal, 41, 108–113.
- Schoenfeld, B. J., Peterson, M. D., Ogborn, D., Contreras, B., & Sonmez, G. T. (2015). Effects of Low- vs. High-load resistance training on muscle strength and hypertrophy in well-trained Men. Journal of Strength and Conditioning Research, 29, 2954–2963.
- Stasinaki, A. N., Zaras, N., Methenitis, S., Tsitkanou, S., Krase, A., Kavvoura, A., & Terzis, G. (2018). Triceps brachii muscle strength and architectural adaptations with resistance training exercises at short or long fascicle length. Journal of Functional Morphology and Kinesiology, 3, 28–39.
- Sugisaki, N., Wakahara, T., Murata, K., Miyamoto, N., Kawakami, Y., Kanehisa, H., & Fukunaga, T. (2015). Influence of muscle hypertrophy on the moment arm of the triceps brachii muscle. Journal of Applied Biomechanics, 31, 111–116.
- Terzis, G., Georgiadis, G., Vassiliadou, E., & Manta, P. (2003). Relationship between shot put performance and triceps brachii fiber type composition and power production. European Journal of Applied Physiology, 90, 10–15.
- Tschakovsky, M. E., & Hughson, R. L. (2000). Venous emptying mediates a transient vasodilation in the human forearm. American Journal of Physiology-Heart and Circulatory Physiology, 279, H1007–H1014.
- Wakahara, T., Fukutani, A., Kawakami, Y., & Yanai, T. (2013). Nonuniform muscle hypertrophy. Medicine & Science in Sports & Exercise, 45, 2158–2165.
- Westcott, W. L. (2012). Resistance training is medicine. Current Sports Medicine Reports, 11, 209–216.