ABSTRACT
The aim of this study was to examine lung function, bronchial hyperresponsiveness (BHR) and exercise-induced respiratory symptoms in elite athletes performing different sports. Norwegian national-team athletes (30 swimmers, 32 cross-country skiers, 16 speed-skaters, 11 rowers/paddlers, 17 handball players and 23 soccer players) completed a validated questionnaire, measured exhaled nitric oxide (FENO), spirometry, methacholine provocation (PD20met) and skin prick test. Three cut-off levels defined BHR; i.e. PD20met ≤2 µmol, ≤4 µmol and ≤8 µmol. Mean forced vital capacity (FVC) was highest in swimmers (Mean z-score[95%CI] = 1.16 [0.80, 1.51]), and close to or higher than reference values according to the Global Lung Initiative equation, across all sports. Mean forced expiratory volume in 1 s (FEV1) was higher than reference values in swimmers (0.48 [0.13, 0.84]), and ball game athletes (0.69 [0.41, 0.97]). Mean forced expiratory flow between 25 and 75% of FVC (FEF25-75), and/or FEV1/FVC were lower than reference values in all endurance groups. BHR defined by ≤2 and ≤8 µmol methacholine was observed in respectively 50%–87% of swimmers, 25%–47% of cross-country skiers, 20%–53% of speed-skaters, 18%–36% of rowers/paddlers, and 0%–17% of the ball game athletes. Exercise-induced symptoms were common in all groups, most frequent in cross-country skiers (88%), swimmers (83%) and speed-skaters (81%).
Highlights
Swimmers and ball game athletes had higher mean FVC and FEV1 when compared to the reference values predicted by the Global Lung Initiative (GLI) reference equation. Contrasting this, across all sports except ball game athletes, mean FEF25-75 and/or FEV1/FVC were lower than reference values.
The prevalence of bronchial hyperresponsiveness (BHR) was high among elite athletes competing in swimming, cross-country skiing, speed skating and rowing/paddling, with swimmers being most affected.
The majority of the elite athletes reported exercise-induced respiratory symptoms independent of lung function or BHR.
Introduction
Studies suggest that elite athletes on average have larger lung volumes and higher maximum airflow rates than healthy sedentary individuals (Doherty & Dimitriou, Citation1997; Rochat et al., Citation2021). The findings may vary between sports, and have been particularly prominent in studies of swimmers and athletes within other water-based activities (Doherty & Dimitriou, Citation1997; Durmic et al., Citation2015; Rochat et al., Citation2021; Rosser-Stanford et al., Citation2019). Causal mechanisms are largely unknown, and particularly we cannot rule out a selection bias linked to the high ventilatory volumes required for success in some of the endurance sports. Nevertheless, decades of high intensity training during childhood, adolescence and young adulthood may impact development of the respiratory system and lead to gradual changes in lung function, perhaps with swimming and/or water-based activities being particularly potent modulators (Armour et al., Citation1993; Clanton et al., Citation1987; Cordain & Stager, Citation1988; Durmic et al., Citation2015; Lazovic et al., Citation2015; Rochat et al., Citation2021). For example, studies that examine lung function across several sports have suggested higher lung volumes in endurance athletes (Lazovic et al., Citation2015) and water polo players (Durmic et al., Citation2015; Mazic et al., Citation2015). Childhood lung growth and developmental trajectories have lifelong implications (Agusti & Faner, Citation2019), and failure to reach the expected peak in early adulthood is linked to later chronic obstructive pulmonary disease (COPD) (Belgrave et al., Citation2018). Thus, a better understanding of the impact of sports on the development of lung function is important and will likely influence clinical practice in the future.
Even though studies indicate high lung volumes and high peak ventilatory capacity in elite athletes, several studies also suggest a high prevalence of exercise-induced respiratory symptoms, exercise-induced bronchoconstriction (EIB) and bronchial hyperresponsiveness (BHR) (Bougault et al., Citation2010; Fitch, Citation2012; Stang et al., Citation2016). Bronchial hyperresponsiveness (BHR) has particularly been observed in endurance athletes practicing winter- and water sports (Atchley & Smith, Citation2020; Mäki-Heikkilä et al., Citation2020; Stang et al., Citation2016), possibly linked to heavy exposures to cold dry winter-air or to the chemical disinfectants used in swimming pools (Atchley & Smith, Citation2020; Couto et al., Citation2018). Couto et al. (Citation2015) have therefore suggested two phenotypes of asthma among elite athletes; a “sports asthma” defined by the presence of exercise-induced respiratory symptoms and BHR, and a more regular “atopic asthma” characterised by allergy, eosinophilic inflammation and increased expired nitric oxide (FENO). The etiology of these two phenotypes probably differs, and to provide optimal treatment it is recommended that athletes’ respiratory health and atopy are systematically tested (Couto et al., Citation2015, Citation2018).
Although a large number of studies have emphasised high prevalence of respiratory disorders among athletes (Atchley & Smith, Citation2020; Fitch, Citation2012), the prevalence rates vary widely between different sports, and are probably also influenced by the criteria used for diagnosis (Bonini & Silvers, Citation2018). Furthermore, a recent systematic review suggests that asthma may be underdiagnosed among cross-country skiers, as many skiers without previously diagnosed asthma fulfilled the diagnostic criterion following a bronchial provocation test (Mäki-Heikkilä et al., Citation2020). Underestimation of asthma may be due to symptoms being misinterpreted as a normal physiological response to high-intensity exercise, and some athletes may thus be unaware of their respiratory disorder (Atchley & Smith, Citation2020; Bougault et al., Citation2010). Alternatively, some athletes may also report respiratory symptoms without clinically having a respiratory disorder (Bougault et al., Citation2010; Boulet & O'Byrne, Citation2015). Hence, we need more studies that measure lung function and BHR objectively in athletes both with and without previously reported asthma or symptoms, and across a range of different sports, using the same methodologies.
The primary objective of the present study was therefore to examine lung function, BHR and exercise-induced respiratory symptoms in athletes at the Norwegian National teams in swimming, cross-country skiing, speed skating, rowing, paddling, handball and soccer.
Methods
Subjects and design
This cross-sectional study is based on data from 25 elite National team swimmers, tested in 2009–2010 and a subsample from a multi-centre study including nine participating countries with data collection performed between 2007 and 2010; the “Asthma and Allergy in Olympians” study. The Olympic study was conducted within the framework of the Global Allergy and Asthma European Network (GA2LEN). The Norwegian National team athletes aiming to qualify for participation in the Olympic games in Beijing 2008 or Vancouver 2010 in swimming, cross-country skiing, speed skating, rowing/paddling, handball and soccer were invited, and all agreed to participate. The sample consisted of 30 swimmers, 32 cross-country skiers, 16 speed skaters, 11 rowers/paddlers, 17 handball players and 23 soccer players. We combined the soccer and handball players into one ball game group. All sport-groups included both male and female athletes, except the ball game group with only female participants. Due to the risk of athletes being identified we cannot report number of males/females and age-range in each sport-group. The athletes were recruited at the National Olympic Centre in Oslo, Norway or via the national sport federation for the corresponding sport.
The Regional Committee for Medical and Health Research Ethics (REC) approved the retrieval of results from medical records of the 25 swimmers (REC number: South-east C-175900). The Olympic study was approved by the REC (REC number: S-07468a). Both studies were carried out according to the principles stated in the Declaration of Helsinki. Signed informed consent was obtained from each subject and in addition from a parent if the subject was younger than 18 years.
Measurements
The athletes attended one visit at the respiratory laboratory at the Norwegian School of Sport Sciences (NSSS), Oslo, Norway, for assessment of respiratory symptoms via a questionnaire, and clinical examinations of fractional exhaled nitric oxide (FENO), spirometry, BHR to methacholine (PD20met) and a skin prick test (SPT) for allergy assessment. Asthma medication was withheld according to current guidelines given by European Respiratory Society (ERS) (Miller et al., Citation2005). Thus, inhaled short-acting beta2 agonists and sodium cromoglycate were withheld for 8 h prior to testing, inhaled long-acting beta2 agonists, theophylline and leukotriene antagonists for the last 72 h, anti-histaminic for the last 7 days, and orally administered glucocorticosteroids for the last 3 months. Inhaled glucocorticosteroids were not allowed to use at the test day. All athletes refrained from exercise and any food or drink containing nitrate on the same day of testing.
Questionnaire
Prior diagnoses of asthma, allergy, use of asthma medication the last year, and current symptoms of cough, phlegm, wheeze, and dyspnoea during or after exercise as well as training volume were recorded with the modified AQUA-questionnaire (Bonini et al., Citation2009).
Lung function
Exhaled nitric oxide (FENO)
Measurement of FENO was performed before spirometry by Eco Medics CLD 88 sp Exhalyzer (Eco Medics AG, 8635 Duernten, Switzerland). Subjects inhaled NO-free air to total lung capacity and exhaled with a standardised flow of 50 ml·s−1 according to ATS/ERS recommendations (American Thoracic Society; European Respiratory Society, Citation2005). Mean values were used after measurements were performed in triplicates.
Spirometry
Lung function was measured by maximum expiratory flow-volume loops using a MasterScreen Pneumo spirometer (Jaeger GmbH, Würzburg, Germany) according to Miller et al. (Citation2005). The following variables were recorded: Forced vital capacity (FVC), forced expiratory volume in the first second (FEV1), forced expiratory flow between 25 and 75% of FVC (FEF25-75), and FEV1/FVC. All maneuvres complied with the general acceptability criteria of the ERS (American Thoracic Society; European Respiratory Society, Citation2005). Raw data were normalised for age, sex, ethnicity, and height according to Global Lung Initiative (GLI) reference equation (Quanjer et al., Citation2012), and presented as z-scores with 95% confidence intervals (CI). The Lower limit of normal (LLN) is defined as a z-score ≤ −1.645 (Quanjer et al., Citation2012). Results presented as actual values and percent of predicted are provided in Supplementary Table 1.
Methacholine provocation test
Methacholine bronchial challenge was performed with an inspiration-triggered nebuliser (Aerosol Provocation System, Jaeger, Würzburg, Germany). The nebuliser output was controlled and calibrated before start of the study and weekly during the study period. Lung function was measured at baseline and after inhaling nebulised isotonic saline (9 mg/ml). Then, methacholine chloride, 32 mg·mL−1, was inhaled in doubling doses from a starting dose of 0.51 µmol (0.1 mg). Lung function measurements were performed one minute after each delivered dose until FEV1 had decreased ≥20% from the measurement after inhaled saline, or the maximum cumulative dose of 24.48 µmol (4.8 mg) was reached. The methacholine dose causing a ≥20% decrease in FEV1 (PD20met) was calculated by linear interpolation on the dose–response curve. Clinically significant BHR was defined using three different criteria: severe PD20met ≤2 µmol, moderate PD20met ≤4 µmol and mild PD20met ≤8 µmol, based on a previous study in elite athletes (Stang et al., Citation2016). After the methacholine provocation, all athletes inhaled salbutamol; 5 mg·mL−1 (0.1 mL·10 kg body mass−1) by nebulisation to reverse bronchial obstruction.
Skin prick test (SPT)
Skin prick tests (SPT) were performed according to the Nordic guidelines (Aas & Belin, Citation1973) with the following locally prevalent ambient allergens: moulds (Cladosporium herbarum), house dust mites (Dermatophagoides pteronyssinus), dog dander, cat dander, birch pollen, grass pollen (timothy), mug worth pollen, milk, shrimp and hen’s egg white (Soluprick, ALK, Copenhagen, Denmark). To be considered a positive response, the weal should measure at least 1/2 of the reaction to histamine 10 mg · mL−1, or the maximum + minimum diameter ([mm]) /2 should exceed 3 mm.
Statistical analyses
Descriptive data are provided as means with standard deviation (SD) for continuous variables and counts with percentages (%) for categorical variables. All continuous variables were normally distributed. Differences in background data across sports were analysed by analysis of variance (ANOVA) for continuous variables, and Fisher's exact test for categorical variables and the corresponding p-values are provided in . Post hoc analyses were performed by Tukey’s test for continuous variables and the significant results from this test are provided in the text. Due to unequal variance in the weight variable, the difference in weight across sports was examined using Welch’s ANOVA, with the corresponding Games-Howell multiple comparisons test.
Table 1. Descriptive statistics of study participants across the different sports. Results are provided as mean with standard deviation (SD) or numbers (n) with percent of total in parentheses.
Results are expressed as mean values with 95% confidence intervals (CI) or counts (%), as appropriate. The association between type of sport and the lung function measurements was tested using linear regression analyses, providing the unstandardised regression coefficients (B) with 95%CI. The ball game athletes were considered the reference category, since ball game was considered the sport that differ the most from the other sports concerning endurance-pattern.
The distribution across the sports regarding the cumulative dose of inhaled methacholine that caused ≥ 20% reduction in FEV1 (PD20met) is illustrated by Kaplan Meier curves. We performed a log-rank test for equality to examine whether the curves differed across the sports.
Although we were able to perform the above-mentioned statistical test to demonstrate possible differences in lung function and responses to methacholine across the different sports, the small sample size prevented us from doing one-to-one comparisons between each sport. Comparisons between the different sports are hence not included in the main aim of this study.
Statistical analyses were performed in Stata SE/16.0 (StataCorp., 2013. Stata Statistical Software: TX: StataCorp LP). P-values ≤ 0.05 was considered statistically significant.
Results
Subject characteristics
provides the characteristics of the participating athletes. The swimmers were significantly younger than the other groups except for the speed skaters, and the speed skaters were younger than the rowers/paddlers. The ball game athletes (all females) had significantly lower weight, height and body mass index (BMI) than the speed skaters and lower height and BMI than the rowing/paddling athletes (both males and females). The use of inhaled corticosteroids (ICS) and ipratropium bromide (IB) was most prevalent in cross-country skiers (71.9% and 62.5%, respectively), whereas the use of antihistamines was most prevalent in speed skaters (37.5%). Short-acting (SABA) and long-acting (LABA) beta2 agonists were most often used by athletes involved in cross-country skiing and rowing/paddling. None of the participants smoked or consumed any other tobacco products.
Lung function
Mean FVC and FEV1 z-scores were higher (mean z-score and 95% CI higher than zero) or close to the estimated reference values in all sports. Mean FEF25-75 and/or FEV1/FVC were lower (mean z-score and 95% CI lower than zero) than the estimated reference values in all sports except in the ball game athletes. The unstandardised regression coefficient suggested that being a swimmer was associated with a 0.53 higher FVC z-score compared to being a ball game athlete. The coefficients further suggest that competing in cross country skiing, speed skating and rowing/paddling was associated with a lower FEV1, whereas all athltes had lower FEF25-75 and FEV1/FVC compared to the ball game athletes (). None of the atheletes had FVC z-scores below LLN, two cross-country skiers had FEV1 z-scores below LLN, whereas there were athletes within all sports who had FEF25-75 and FEV1/FVC z-scores below LLN.
Table 2. Lung function of elite athletes participating in different sports. Values are presented in z-scores and Lower Limit of Normal (LLN) according to Quanjer et al. (Citation2012) (Lazovic-Popovic et al., Citation2016).
Bronchial hyperresponsiveness, allergy and respiratory symptoms
The distribution of athletes responding with a reduction in FEV1 ≥20%, after inhaling cumulative doses of methacholine, varied across the sports (). The highest rate of BHR was observed in swimmers and the lowest in ball game athletes, irrespective of cut-off level ( and ).
Figure 1. The distribution of the elite athletes’ responses to increasing cumulative doses of methacholine across the different sports, with a reduction in FEV1 ≥20% as the event of interest. The vertical line shows the diagnostic criteria for severe BHR (PD20met ≤2 µmol), moderate BHR (PD20met ≤4 µmol) and mild BHR (PD20met ≤8 µmol). The Kaplan-Meier curves differ depending on sport (p < 0.001), with the highest proportion of responding athletes in the swimmers.
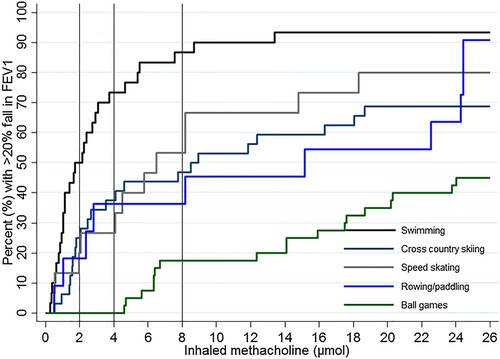
Table 3. Allergy, bronchial hyperresponsiveness (BHR), fractional exhaled nitric oxide (FENO), self-reported exercise-induced respiratory symptoms and doctor diagnosed asthma in elite athletes participating in different sports
Mean FENO levels ranged from 14.4–22.6 ppb across the different sports and was within normal reference values in all groups (). The number of athletes with one or more positive skin prick tests ranged from 26% to 54% across the different sports (). A large proportion of the athletes reported exercise-induced respiratory symptoms, with highest prevalence in swimmers, cross-country skiers and speed skaters (). Cough was the most prevalent reported respiratory symptom in cross-country skiers, speed skaters and the ball game athletes, whereas both cough and chest tightness were the most common reported respiratory symptoms in swimmers and rowers/paddlers.
Discussion
In this study of elite athletes, we found high mean FVC across all sports, but particularly in swimmers, and that swimmers and ball game athletes had higher mean FEV1 compared to reference values and the other sports groups. Bronchial hyperresponsiveness (BHR) was common in all sports, except in the ball game athletes, and especially prominent among swimmers where 50% had severe BHR. Most of the swimmers, cross-country skiers and speed skaters had BHR and/or reported exercise-induced respiratory complaints.
Mean FVC and FEV1 were close to or in the upper range of values predicted by the applied reference equation in all sports groups, whereas FEF25-75 and FEV1/FVC were in the lower range, particularly for athletes engaged in swimming, cross-country skiing and rowing/paddling. In several athletes within these three endurance sports, FEF25-75 and FEV1/FVC were below LLN. A consistent tendency of high lung volumes and high airflow values in swimmers is in line with several previous studies (Armour et al., Citation1993; Doherty & Dimitriou, Citation1997; Lazovic-Popovic et al., Citation2016; Rosser-Stanford et al., Citation2019; Silvestri et al., Citation2013). Our results also suggest that ball game athletes have higher FVC and FEV1 than the reference values, and similar FEV1 values as swimmers. These findings are in agreement with the study by Rosser-Stanford et al. (Citation2019), whereas some other studies do not support a higher FVC and FEV1 in ball game athletes (Durmic et al., Citation2015; Lazovic-Popovic et al., Citation2016). Lazovic et al. (Citation2015) examined lung function in male athletes across a wide range of sports, and their results suggest better than average lung function in several groups of athletes. The endurance athletes – including rowing and canoeing, swimming, long–distance running, marathon, cycling, triathlon and pentathlon – had the highest FVC. Mazic et al. (Citation2015) also studied lung function in athletes competing at an international level across several sports, and their results suggested enhanced lung function, especially in water polo players.
We need more studies to confirm and further examine the observation that a relatively large proportion of the endurance sports athletes demonstrate low FEF25-75 and FEV1/FVC. Despite their high to normal FEV1, this could signal ongoing pathophysiology in small airways, as suggested in studies of athletes (Sue-Chu et al., Citation1999) and normal populations (Qin et al., Citation2021). Conceivably, a high FVC could also contribute to low FEF25-75 and FEV1/FVC (Sherter et al., Citation1978), although ball game athletes had both high FVC and normal FEF25-75 and FEV1/FVC.
The mechanisms explaining high lung volumes in swimmers are still unclear, but it has been discussed whether it is related to the characteristic nature of breathing a successful swimmer must adopt; i.e. a short time to inhale followed by a relatively slow exhalation against a positive pressure of 5–10 cm H2O. This breathing pattern requirement will of course favour those who are able to cope, and thus we cannot exclude a bias related to genetic factors that foster the necessary features and characteristics (Armour et al., Citation1993; Clanton et al., Citation1987; Cordain & Stager, Citation1988; Lazovic-Popovic et al., Citation2016; Rochat et al., Citation2021). In order to disentangle these important questions, we need prospective studies that focus on the development of lung function from childhood to adulthood, scrutinising the influence of training load (volume and intensity) and the type of sport. Only then can we understand if there is a causal relationship between swim training – or other types of training – and improved lung function. We know that lung function normally increases through childhood and adolescence, reach a peak in the mid-twenties, and then gradually declines with age (Agusti & Faner, Citation2019). This trajectory is modulated by genetic mechanisms, antenatal factors, and multiple early-life events and exposures (Bush, Citation2016). For most people, the adult decline proceeds unrecognised as symptoms only appear if lung function reaches very low levels (approximately at 75% of reference FEV1) (Jakeways et al., Citation2003). However, failure to reach the expected peak in early adulthood is linked to development of chronic obstructive pulmonary disease (COPD) (Belgrave et al., Citation2018). If early life lung function growth can be modulated by sports activities during childhood and/or adolescence, this might represent a therapeutic opportunity for those at risk of early onset of COPD, for example individuals exposed to antenatal or early life adverse events or premature birth (Belgrave et al., Citation2018; Bush, Citation2016).
The distribution of the athletes’ response to methacholine suggested large differences across sports. Bronchial hyperresponsiveness (BHR) was clearly most prominent in the swimmers, with prevalence rates ranging from 50% to 87% depending on which cut-off level was used. Nevertheless, we also observed a high prevalence in cross-country skiers (25–47%), speed skaters (20–53%) and rowers/paddlers (18–36%). Langdeau et al. (Citation2009) observed that BHR, assessed with methacholine challenge, was significantly higher in female athletes compared to male athletes. Despite the apparent female disadvantage, the female ball game athletes were the least affected in this present study, with only 17.5% responders at a cumulative dose of 8 µmol. Notably, despite low numbers with BHR among ball game athletes, more than half reported exercise-induced respiratory symptoms. The high prevalence of BHR in swimmers is consistent with most previous studies (Bougault et al., Citation2010; Stang et al., Citation2016). Three studies examining athletes competing in the Olympic Games or the World Championships reported a high prevalence of documented BHR and asthma – defined by the proportion applying for a therapeutic use exemption (TUE) for SABA (Fitch, Citation2012; Mountjoy et al., Citation2015) or a doctor’s diagnosed asthma (Burns et al., Citation2015) – in both summer (Burns et al., Citation2015; Fitch, Citation2012; Mountjoy et al., Citation2015) and winter athletes (Fitch, Citation2012) participating in prolonged endurance sports. Also in these studies, the highest prevalence of BHR was observed in athletes engaged in endurance sports combined with water sports, e.g. swimmers, artistic swimmers, and triathletes (Burns et al., Citation2015; Fitch, Citation2012; Mountjoy et al., Citation2015). High minute ventilation achieved during repeated high intensity endurance exercise is believed to contribute to the development of BHR in endurance athletes, as this causes significant mechanical, thermic and osmotic stress to the airway epithelium (Haahtela et al., Citation2008). This effect may be aggravated by unfavorable environmental exposures, such as chlorine subproducts in indoor swimming pools, or cold and dry air in outdoor sports. Increased sputum eosinophils and bronchial epithelial cells have been found in swimmers, suggesting airway epithelial damage, airway inflammatory and remodeling processes. In addition, sputum neutrophil counts are shown to correlate with training hours in both swimmers and cold air athletes (Bougault et al., Citation2009).
In agreement with previous studies (Bougault et al., Citation2010; Stang et al., Citation2016), our results also support that a high proportion of athletes report exercise-induced respiratory symptoms. The high prevalence of BHR and exercise-induced respiratory symptoms, combined with mean FENO within normal values (Dweik et al., Citation2011), suggest that most of the athletes in the present study may have developed the “sports asthma” phenotype, as discussed by Couto et al. (Citation2015). However, the confidence intervals of the mean FENO-measurements were wide among the cross-country skiers, speed skaters and rowers/paddlers. Moreover, a slightly higher prevalence of allergy in the speed skaters and rowers/paddlers compared to the average Norwegian adult population (about 40%) (NAAF, Citation2021), suggests that some of these athletes may have the phenotype “atopic asthma”. Inevitably, there will of course be individuals also among these athletes who would have developed asthma, BHR and respiratory symptoms irrespective of a career as athletes. We need long-term prospective studies starting from young adolescence to disentangle causal mechanisms behind the development of BHR and exercise-induced respiratory symptoms across different sports; e.g. the role of atopy, training environment, training intensity, and type of activity.
Strengths and limitations
Major strengths of the present study were the objective measures used to assess lung function and BHR in a total population of athletes performing at the highest national level in their respective sports, and the inclusion of both summer and winter sports, as well as water and non-water sports. Furthermore, the same trained test-personnel performed all measurements in all athletes across the different sports, which is likely to increase the reliability of the results. Nevertheless, the results should be interpreted keeping some important considerations in mind. The ball game athletes were only females, which limits the generalizability of the results to males.
In our study, BHR was assessed using a methacholine provocation test. However, eucapnic voluntary hyperpnoea (EVH) is now recommended by the International Olympic Committee -Medical Commission (IOC-MC) and is considered the most specific and sensitive provocation test for BHR in athletes. EVH acts indirectly through the release of inflammatory contractile mediators, and thus suggested to better mimic the trigger factors for EIB in athletes (Hull et al., Citation2016). Methacholine on the other hand, acts directly on muscarine receptors on the airway smooth muscle cells causing bronchoconstriction, and the test is considered to be similarly sensitive, but less specific than EVH (Boulet & O'Byrne, Citation2015). Even though EVH has a key role in diagnosing EIB in athletes, the test is not yet recommended as the “gold standard test” due to poor repeatability in mild asthmatic athletes (Hull et al., Citation2016). Further, as Boulet and O’Byrne state in their review (Boulet & O'Byrne, Citation2015), athletes may respond differently to different provocation tests, and they therefore suggest that more than one diagnostic test for asthma can be required.
Furthermore, the cross-sectional design of this study limits our possibility to infer causality, and we emphasise the importance and need for prospective studies to examine the impact of type of sports on lung function growth and development of respiratory disorders. Although withheld a day prior to testing, the effects from ICS were of course still present in those who used them, which may have influenced the results, especially in the cross-country skiers where the majority of the athletes (72%) used this medication. Exercise-induced respiratory symptoms were based on self-reports, which is a strategy prone to error (Atchley & Smith, Citation2020; Boulet & O'Byrne, Citation2015; Hull et al., Citation2016).
Conclusion
Mean FVC was higher or close to reference values in all sports, and elite swimmers and ball game athletes had higher mean FEV1 compared to reference values. Endurance athletes, but not ball game athletes, demonstrated low FEF25-75 and FEV1/FVC despite their high to normal FEV1. The prevalence of severe BHR was particularly high in swimmers (50%), but high also in cross-country skiers (25%), speed skaters (20%), and rowers/paddlers (18%), but not in ball game athletes (0%). More than half of the elite athletes in all sports reported exercise-induced respiratory symptoms, with highest prevalence in swimmers, cross-country skiers and speed skaters. Cough was the most frequent complaint. Respiratory health might be influenced by years of training; findings that raise speculations regarding pathophysiology as well as novel treatment avenues. The study substantiates a need for systematic follow-up of endurance athletes’ respiratory health, based on objective tests as well as self-reported symptoms.
Data sharing
The consent given by the participants does not open for sharing the full data set.
Ethical approval
The study was approved by the Regional Committee for Medical and Health Research Ethics (REC number: S-07468a and REC number: South-east C-175900).
Supplemental Material
Download MS Word (18.2 KB)Acknowledgements
The authors would like to pay a special tribute and thank you to the late Professor Kai-Haakon Carlsen for initiating and organising the important study “Asthma and allergy in Olympians” in 2007. Further, we are indeed grateful to all the athletes who took part in this study. Contributorship: Kai-Håkon Carlson and Trine Stensrud planned the study and collected the data. All authors conceptualised the specific research question and planned the analyses. Guro P. Bernhardsen analysed the data. Guro P. Bernhardsen and Trine Stensrud drafted the manuscript. Julie Stang and Thomas Halvorsen critically reviewed all versions of the manuscript and approved the final version.
Disclosure statement
No potential conflict of interest was reported by the author(s).
Additional information
Funding
References
- Aas, K., & Belin, L. (1973). Standardization of diagnostic work in allergy. International Archives of Allergy and Immunology, 45(1), 57–60. https://doi.org/10.1159/000231002
- Agusti, A., & Faner, R. (2019). Lung function trajectories in health and disease. The Lancet Respiratory Medicine, 7(4), 358–364. https://doi.org/10.1016/S2213-2600(18)30529-0
- American Thoracic Society; European Respiratory Society. (2005). ATS/ERS recommendations for standardized procedures for the online and offline measurement of exhaled lower respiratory nitric oxide and nasal nitric oxide, 2005. American Journal of Respiratory and Critical Care Medicine, 171(8), 912–930. https://doi.org/10.1164/rccm.200406-710ST
- Armour, J., Donnelly, P. M., & Bye, P. T. (1993). The large lungs of elite swimmers: An increased alveolar number? European Respiratory Journal, 6(2), 237–247.
- Atchley, T. J., & Smith, D. M. (2020). Exercise-induced bronchoconstriction in elite or endurance athletes. Annals of Allergy, Asthma & Immunology, 125(1), 47–54. https://doi.org/10.1016/j.anai.2020.01.023
- Belgrave, D. C. M., Granell, R., Turner, S. W., Curtin, J. A., Buchan, I. E., Le Souef, P. N., Simpson, A., Henderson, A. J., & Custovic, A. (2018). Lung function trajectories from pre-school age to adulthood and their associations with early life factors: A retrospective analysis of three population-based birth cohort studies. The Lancet Respiratory Medicine, 6(7), 526–534. https://doi.org/10.1016/S2213-2600(18)30099-7
- Bonini, M., Braido, F., Baiardini, I., Del Giacco, S., Gramiccioni, C., Manara, M., Tagliapietra, G., Scardigno, A., Sargentini, V., Brozzi, M., Rasi, G., & Bonini, S. (2009). AQUA©: allergy questionnaire for athletes. Development and validation. Medicine & Science in Sports & Exercise, 41(5), 1034–1041. https://doi.org/10.1249/MSS.0b013e318193c663
- Bonini, M., & Silvers, W. (2018). Exercise-induced bronchoconstriction: Background, prevalence, and sport considerations. Immunology and Allergy Clinics of North America, 38(2), 205–214. https://doi.org/10.1016/j.iac.2018.01.007
- Bougault, V., Turmel, J., & Boulet, L. P. (2010). Bronchial challenges and respiratory symptoms in elite swimmers and winter sport athletes: Airway hyperresponsiveness in asthma: Its measurement and clinical significance. Chest, 138(2 Suppl), 31S–37S. https://doi.org/10.1378/chest.09-1689
- Bougault, V., Turmel, J., St-Laurent, J., Bertrand, M., & Boulet, L. P. (2009). Asthma, airway inflammation and epithelial damage in swimmers and cold-air athletes. European Respiratory Journal, 33(4), 740–746. https://doi.org/10.1183/09031936.00117708
- Boulet, L. P., & O'Byrne, P. M. (2015). Asthma and exercise-induced bronchoconstriction in athletes. New England Journal of Medicine, 372(7), 641–648. https://doi.org/10.1056/NEJMra1407552
- Burns, J., Mason, C., Mueller, N., Ohlander, J., Zock, J.-P., Drobnic, F., Wolfarth, B., Heinrich, J., Omenaas, E., Stensrud, T., Nowak, D., & Radon, K. (2015). Asthma prevalence in Olympic summer athletes and the general population: An analysis of three European countries. Respiratory Medicine, 109(7), 813–820. https://doi.org/10.1016/j.rmed.2015.05.002
- Bush, A. (2016). Lung development and aging. Annals of the American Thoracic Society, 13(Suppl 5), S438–Ss46. https://doi.org/10.1513/AnnalsATS.201602-112AW
- Clanton, T. L., Dixon, G. F., Drake, J., & Gadek, J. E. (1987). Effects of swim training on lung volumes and inspiratory muscle conditioning. Journal of Applied Physiology, 62(1), 39–46. https://doi.org/10.1152/jappl.1987.62.1.39
- Cordain, L., & Stager, J. (1988). Pulmonary structure and function in swimmers. Sports Medicine, 6(5), 271–278. https://doi.org/10.2165/00007256-198806050-00002
- Couto, M., Kurowski, M., Moreira, A., Bullens, D. M. A., Carlsen, K. H., Delgado, L., Kowalski, M. L., & Seys, S. F. (2018). Mechanisms of exercise-induced bronchoconstriction in athletes: Current perspectives and future challenges. Allergy, 73(1), 8–16. https://doi.org/10.1111/all.13224
- Couto, M., Stang, J., Horta, L., Stensrud, T., Severo, M., Mowinckel, P., Silva, D., Delgado, L., Moreira, A., & Carlsen, K.-H. (2015). Two distinct phenotypes of asthma in elite athletes identified by latent class analysis. Journal of Asthma, 52(9), 897–904. https://doi.org/10.3109/02770903.2015.1067321
- Doherty, M., & Dimitriou, L. (1997). Comparison of lung volume in Greek swimmers, land based athletes, and sedentary controls using allometric scaling. British Journal of Sports Medicine, 31(4), 337–341. https://doi.org/10.1136/bjsm.31.4.337
- Durmic, T., Lazovic, B., Djelic, M., Lazic, J. S., Zikic, D., Zugic, V., Dekleva, M., & Mazic, S. (2015). Sport-specific influences on respiratory patterns in elite athletes. Jornal Brasileiro de Pneumologia, 41(6), 516–522. https://doi.org/10.1590/s1806-37562015000000050
- Dweik, R. A., Boggs, P. B., Erzurum, S. C., Irvin, C. G., Leigh, M. W., Lundberg, J. O., Olin, A.-C., Plummer, A. L., & Taylor, D. R. (2011). An official ATS clinical practice guideline: Interpretation of exhaled nitric oxide levels (FeNO) for clinical applications. American Journal of Respiratory and Critical Care Medicine, 184(5), 602–615. https://doi.org/10.1164/rccm.9120-11ST
- Fitch, K. D. (2012). An overview of asthma and airway hyper-responsiveness in Olympic athletes. British Journal of Sports Medicine, 46(6), 413–416. https://doi.org/10.1136/bjsports-2011-090814
- Haahtela, T., Malmberg, P., & Moreira, A. (2008). Mechanisms of asthma in Olympic athletes – practical implications. Allergy, 63(6), 685–694. https://doi.org/10.1111/j.1398-9995.2008.01686.x
- Hull, J. H., Ansley, L., Price, O. J., Dickinson, J. W., & Bonini, M. (2016). Eucapnic voluntary hyperpnea: Gold standard for diagnosing exercise-induced bronchoconstriction in athletes? Sports Medicine, 46(8), 1083–1093. https://doi.org/10.1007/s40279-016-0491-3
- Jakeways, N., McKeever, T., Lewis, S. A., Weiss, S. T., & Britton, J. (2003). Relationship between FEV1 reduction and respiratory symptoms in the general population. European Respiratory Journal, 21(4), 658–663. https://doi.org/10.1183/09031936.03.00069603
- Langdeau, J. B., Day, A., Turcotte, H., & Boulet, L. P. (2009). Gender differences in the prevalence of airway hyperresponsiveness and asthma in athletes. Respiratory Medicine, 103(3), 401–406. https://doi.org/10.1016/j.rmed.2008.09.023
- Lazovic, B., Mazic, S., Suzic-Lazic, J., Djelic, M., Djordjevic-Saranovic, S., Durmic, T., Zikic, D., and Zugic, V. (2015). Respiratory adaptations in different types of sport. European Review for Medical and Pharmacological Sciences, 19(12), 2269–2274.
- Lazovic-Popovic, B., Zlatkovic-Svenda, M., Durmic, T., Djelic, M., Djordjevic Saranovic, S., & Zugic, V. (2016). Superior lung capacity in swimmers: Some questions, more answers! Revista Portuguesa de Pneumologia (English Edition), 22(3), 151–156. https://doi.org/10.1016/j.rppnen.2015.11.003
- Mäki-Heikkilä, R., Karjalainen, J., Parkkari, J., Valtonen, M., & Lehtimäki, L. (2020). Asthma in competitive cross-country skiers: A systematic review and meta-analysis. Sports Medicine, 50, 1963–1981. https://doi.org/10.1007/s40279-020-01334-4
- Mazic, S., Lazovic, B., Djelic, M., Suzic-Lazic, J., Djordjevic-Saranovic, S., Durmic, T.…Zugic, V., (2015). Respiratory parameters in elite athletes–does sport have an influence? Revista Portuguesa de Pneumologia, 21(4), 192–197. https://doi.org/10.1016/j.rppnen.2014.12.003
- Miller, M. R., Hankinson, J., Brusasco, V., Burgos, F., Casaburi, R., Coates, A.…Wangler, J., (2005). Standardisation of spirometry. European Respiratory Journal, 26(2), 319–338. https://doi.org/10.1183/09031936.05.00034805
- Mountjoy, M., Fitch, K., Boulet, L. P., Bougault, V., van Mechelen, W., & Verhagen, E. (2015). Prevalence and characteristics of asthma in the aquatic disciplines. Journal of Allergy and Clinical Immunology, 136(3), 588–594. https://doi.org/10.1016/j.jaci.2015.01.041
- NAAF. (2021). Allergy prevalence [Web site]. Norwegian asthma and allergy association. https://www.naaf.no/fokusomrader/allergi-og-overfolsomhet/fakta-om-allergi/
- Qin, R., An, J., Xie, J., Huang, R., Xie, Y., He, L., Xv, H., Qian, G., & Li, J. (2021). FEF25-75% is a more sensitive measure reflecting airway dysfunction in patients with asthma: A comparison study using FEF25-75% and FEV1%. The Journal of Allergy and Clinical Immunology: In Practice, 9(10), 3649–3659.e6. https://doi.org/10.1016/j.jaip.2021.06.027
- Quanjer, P. H., Stanojevic, S., Cole, T. J., Baur, X., Hall, G. L., Culver, B. H., Enright, P. L., Hankinson, J. L., Ip, M. S. M., Zheng, J., & Stocks, J. (2012). Multi-ethnic reference values for spirometry for the 3-95-yr age range: The global lung function 2012 equations. European Respiratory Journal, 40(6), 1324–1343. https://doi.org/10.1183/09031936.00080312
- Rochat, I., Cote, A., & Boulet, L. P. (2021). Determinants of lung function changes in athletic swimmers. A review. Acta Paediatrica, 111(2), 259–264. https://doi.org/10.1111/apa.16095
- Rosser-Stanford, B., Backx, K., Lord, R., & Williams, E. M. (2019). Static and dynamic lung volumes in swimmers and their ventilatory response to maximal exercise. Lung, 197(1), 15–19. https://doi.org/10.1007/s00408-018-0175-x
- Sherter, C. B., Connolly, J. J., & Schilder, D. P. (1978). The significance of volume-adjusting the maximal midexpiratory flow in assessing the response to a bronchodilator drug. Chest, 73(5), 568–571. https://doi.org/10.1378/chest.73.5.568
- Silvestri, M., Crimi, E., Oliva, S., Senarega, D., Tosca, M. A., Rossi, G. A., & Brusasco, V. (2013). Pulmonary function and airway responsiveness in young competitive swimmers. Pediatric Pulmonology, 48(1), 74–80. https://doi.org/10.1002/ppul.22542
- Stang, J., Stensrud, T., Mowinckel, P., & Carlsen, K.-H. (2016). Parasympathetic activity and bronchial hyperresponsiveness in athletes. Medicine & Science in Sports & Exercise, 48(11), 2100–2107. https://doi.org/10.1249/MSS.0000000000001008
- Sue-Chu, M., Larsson, L., Moen, T., Rennard, S. I., & Bjermer, L. (1999). Bronchoscopy and bronchoalveolar lavage findings in cross-country skiers with and without “ski asthma”. European Respiratory Journal, 13(3), 626–632. https://doi.org/10.1183/09031936.99.13362699